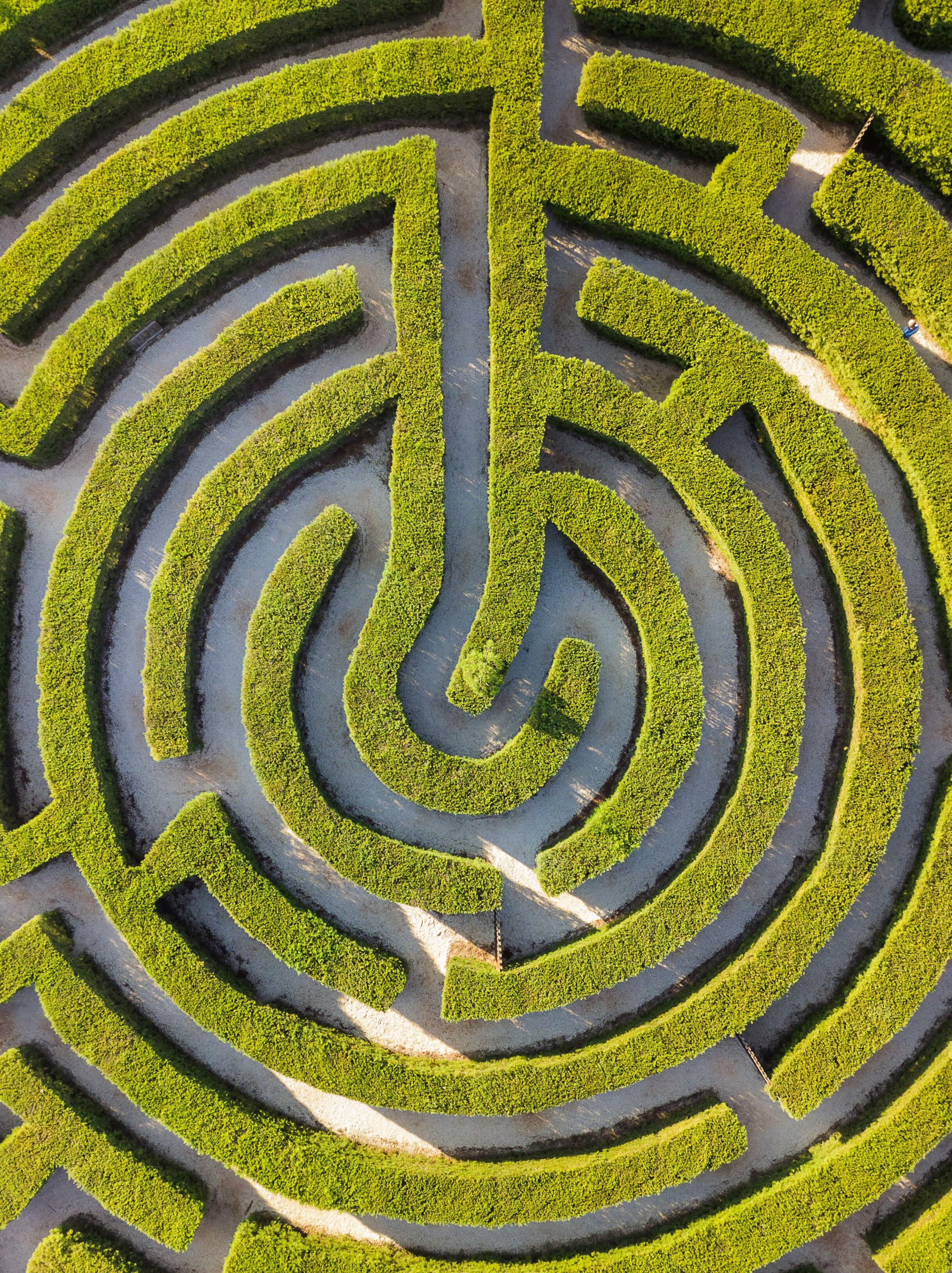
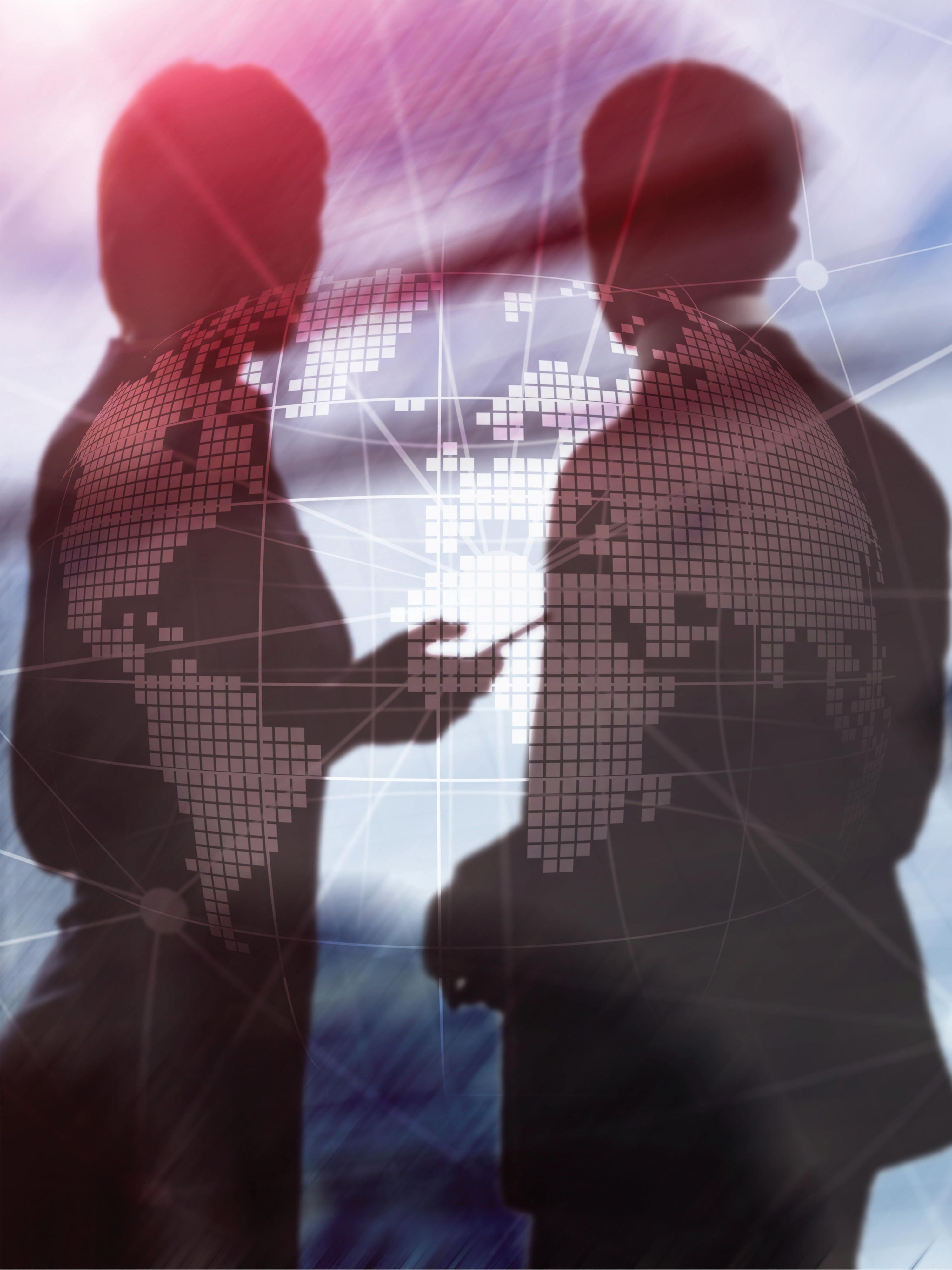
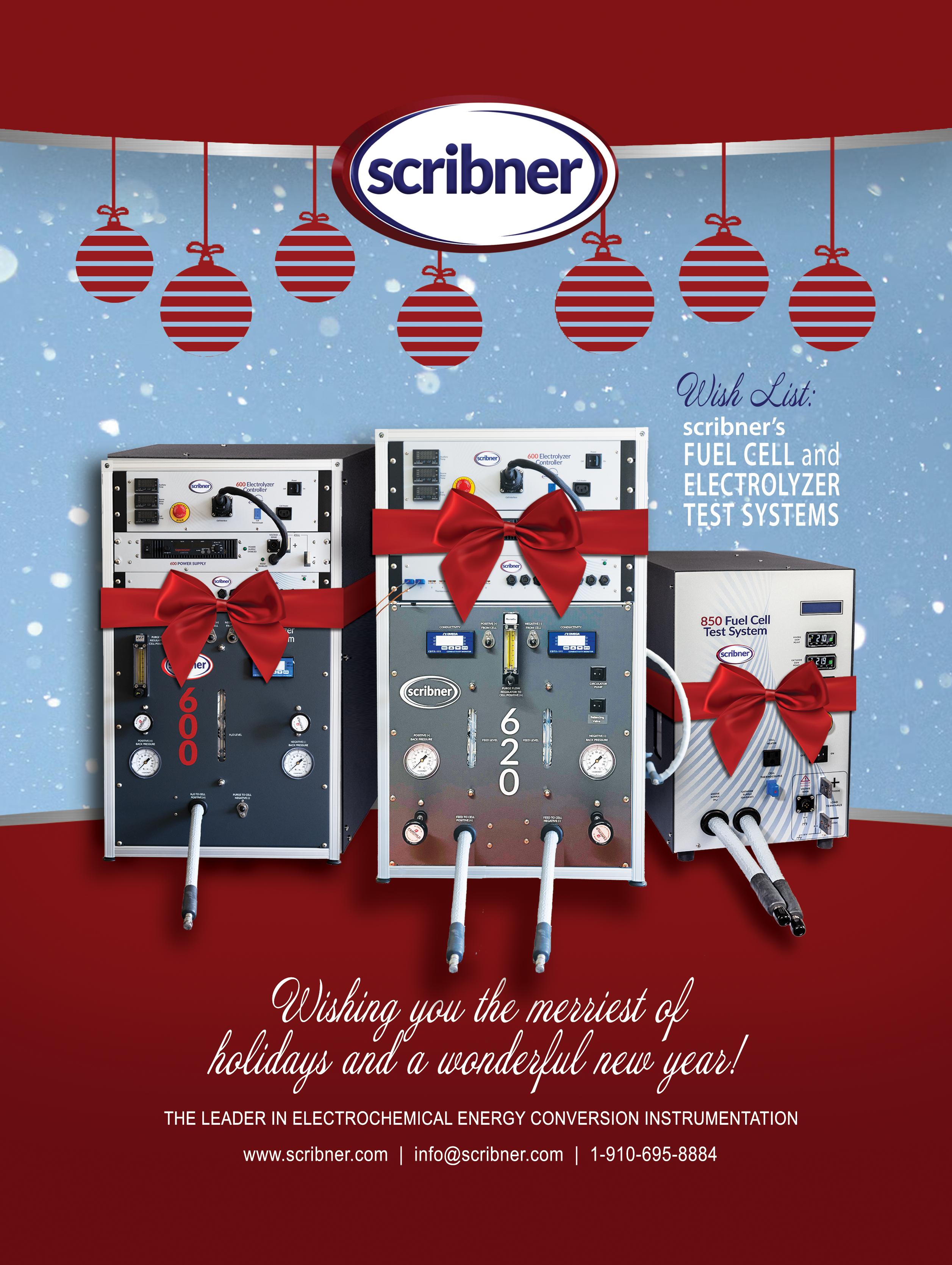
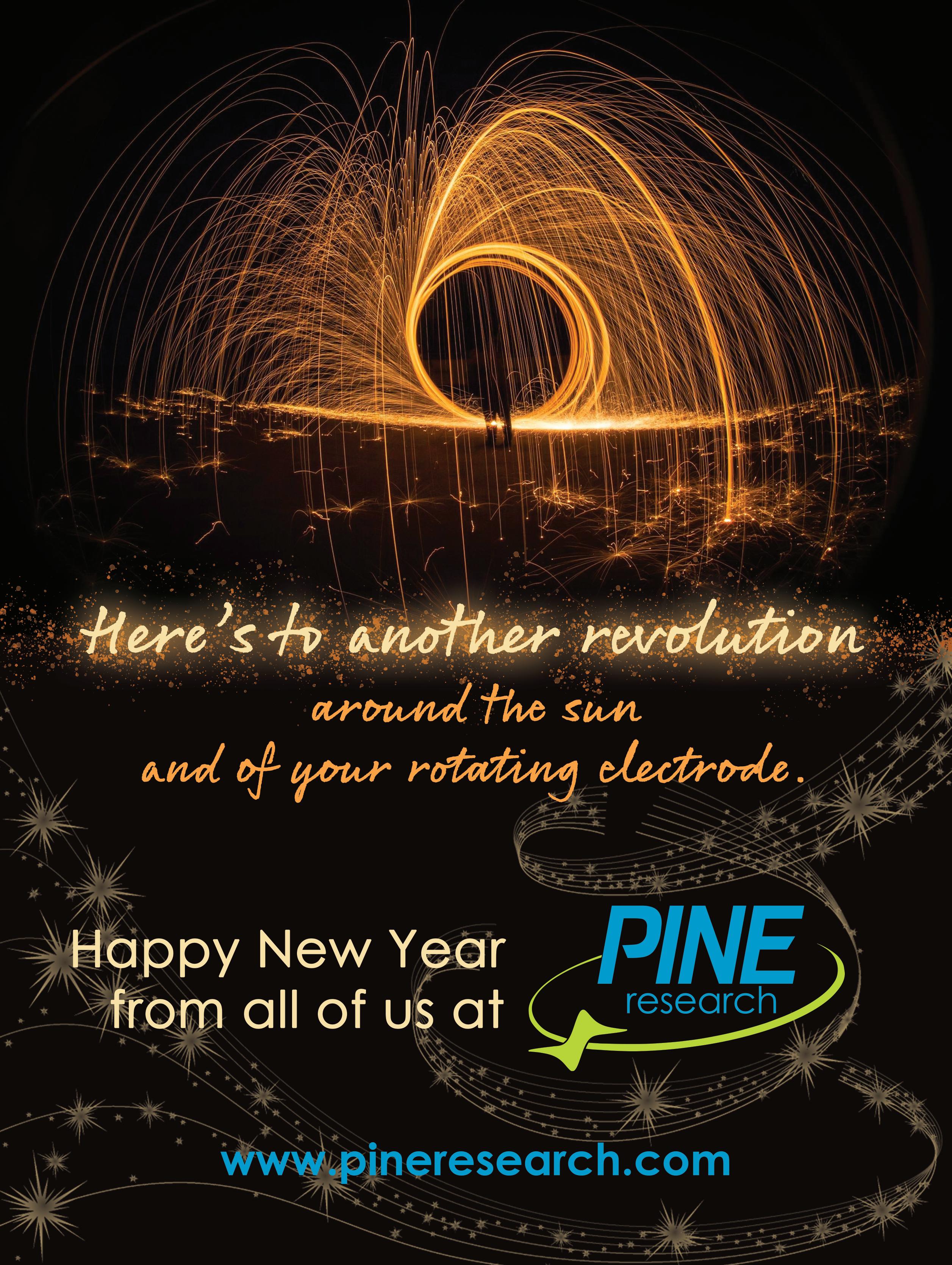
It may come as a surprise that content for the Winter issue of Interface is actually completed by the middle of September. The seemingly early due date is needed to allow for the design and production process required to create the beauty that you are looking at right now. I always struggle trying to figure out what to write about that will still have legs in three months (I can see the nodding heads). Our department is in the midst of our PhD qualifying exams, an annual fall event that stirs memories/nightmares even after [deleted] years have passed since my experience with it. For those readers who have not suffered through experienced such an exam, one component of all PhD qualifiers is an oral exam. Although it varies from department to department, the broad characteristics are the same: on the order of four faculty ask the candidate technical questions with the entire exam lasting ~ 2hrs. Sometimes questions are about a mini-proposal the candidate has written, sometimes the questions are about field-specific fundamentals. In all cases, the candidate is going through what will be one of the greatest anxiety-causing experiences of their professional life. It represents a critical professional gateway, and the anticipation of the event is almost as brutal as the event itself. But there is no path to a PhD without going through that gate.
Successful completion demonstrates not only a grasp of the subject matter, but also an ability to synthesize different aspects of the knowledge base as required to attack a technical question. In addition, professional life is largely an oral exam. Yes, we capture our thoughts and arguments in our papers in the academic world, but real-time technical discussions are often key in decisions about government policies, industrial directions, liability judgements, as well as scientific acceptance of one’s work. We have all had the experience of thinking of a better response than the one we gave minutes, hours, or days after an argument (for those of you who argue). The ability to think on one’s feet while under stress can be a key skill.
Because each questioner has a time limit (e.g., 20 min), they often cut the student off once it is clear that the student knows the answer, moving on to the next topic. One key goal is to find the point where the student doesn’t know the answer to determine their ability to think through problems, as that is the essence of research. As the sign outside my office says, quoting Albert Einstein: “If we knew what we were doing, it wouldn’t be research.” Somewhat counterintuitively, in many cases it is the students who have done very, very well academically who suffer the most. They are not used to not knowing the answer, as that is how they achieved their high grades. The first experience of the distress of being intellectually lost is jarring. For those who were a bit full of themselves, it is eye-opening to realize that maybe they aren’t all that and a bag of chips. Facing our own limitations is hard, but being okay with not knowing or being wrong is critical to successful research (not to mention life).
For many, if not most, students, the oral exam is a turning point in their development. After passing the gauntlet of questions from four grumpy faculty, students who had been lacking in self-confidence realize how smart they are. They become more willing to risk being wrong in the pursuit of understanding. For (sometimes) the first time in their life, they can tell that guy we all have on our shoulder who provides constant critiques that he can shut up. It divides their professional life into two eras: before the exam and after the exam. They even walk a bit taller. Of course, PhD oral exams have analogies in other career paths as well. Heather still vividly recalls every question posed during her anesthesiology board examination just as I still remember one of my examiners saying, “Well, no, not really,” after each of my answers to his questions. I still think my passing must have been due to a numerical mistake when scores were added. Of course, the $50 that I slipped the committee chair may have helped, too.
So, the moral of the story is that it is often via the scariest challenges that we grow the most (except those involving snakes). We often face them because we want something that is on the other side of them. It is during those times that we should be brave and scared. We need to believe in ourselves like we believe in others.
Until next time, be safe and happy.
Published by: The Electrochemical Society (ECS) 65 South Main Street Pennington, NJ 08534-2839, USA Tel 609.737.1902, Fax 609.737.2743 www.electrochem.org
Editor: Rob Kelly
Contributing Editors: Christopher L. Alexander, Chris Arges, Scott Cushing, Ahmet Kusolgu, Donald Pile, Alice Suroviec
Director of Publications: Adrian Plummer
Director of Community Engagement: Shannon Reed
Production Editor: Kara McArthur
Graphic Design & Print Production Manager: Dinia Agrawala
Staff Contributors: Frances Chaves, Genevieve Goldy, Mary Hojlo, Christopher J. Jannuzzi, John Lewis, Anna Olsen, Jennifer Ortiz, Beth Schademann, Francesca Spagnuolo
Advisory Board: Brett Lucht (Battery), Dev Chidambaram (Corrosion), Durga Misra (Dielectric Science and Technology), Philippe Vereecken (Electrodeposition), Jennifer Hite (Electronics and Photonics), Mani Manivannan (Energy Technology), Cortney Kreller (High-Temperature Energy, Materials, & Processes), John Weidner (Industrial Electrochemistry and Electrochemical Engineering), Jakoah Brgoch (Luminescence and Display Materials), Hiroshi Imahori (Nanocarbons), James Burgess (Organic and Biological Electrochemistry), Robbyn Anand (Physical and Analytical Electrochemistry), Ajit Khosla (Sensor)
Publications Subcommittee Chair: Colm O'Dwyer
Society Officers: Turgut Gür, President; Gerardine Botte, Senior Vice President; Colm O’Dwyer, 2nd Vice President; James (Jim) Fenton, 3rd Vice President; Marca Doeff, Secretary; Elizabeth J. Podlaha-Murphy, Treasurer; Christopher J. Jannuzzi, Executive Director & CEO
Statements and opinions given in The Electrochemical Society Interface are those of the contributors, and ECS assumes no responsibility for them.
Authorization to photocopy any article for internal or personal use beyond the fair use provisions of the Copyright Act of 1976 is granted by The Electrochemical Society to libraries and other users registered with the Copyright Clearance Center (CCC). Copying for other than internal or personal use without express permission of ECS is prohibited. The CCC Code for The Electrochemical Society Interface is 1064-8208/92.
ISSN : Print: 1064-8208 Online: 1944-8783
The Electrochemical Society Interface is published quarterly by The Electrochemical Society (ECS), at 65 South Main Street, Pennington, NJ 08534-2839 USA. Subscription to members is part of membership service. © Copyright 2022 by The Electrochemical Society. *“Save as otherwise expressly stated.”
The Electrochemical Society is an educational, nonprofit 501(c)(3) organization with more than 8,500 scientists and engineers in over 75 countries worldwide who hold individual membership. Founded in 1902, the Society has a long tradition in advancing the theory and practice of electrochemical and solid state science by dissemination of information through its publications and international meetings.
“... professional life is largely an oral exam.”
Expanding on my earlier statement in the summer 2022 issue of Interface,
I wish to share my views with you on the pressing challenges regarding sustainable energy and the environment. I will take an entirely global perspective in viewing these complex and difficult issues, irrespective of local or regional energy practices and trends that can mislead to tunnel vision. I would also note that the views expressed in this article are solely my own and do not necessarily represent or reflect the collective view of our Society.
Energy is central to almost every aspect of life and human activity and has historically been dominated by fossil fuels that dramatically impact the climate and the environment. The resulting CO2 constitutes more than 80% of the total greenhouse gas emissions responsible for climate change and the continued warming of our planet (Fig. 1). Climate change is an existential problem. Being cognizant of this imminent danger, ECS recently issued a public statement pledging the commitment of its members to work toward sustainable solutions and advocating science-driven policies to combat climate change.
Pathways to transition into a clean sustainable energy economy pose serious challenges with difficult options. There is no silver bullet, no single recipe. Energy security requires a multi-pronged approach to achieve diversity in sustainable technologies and input sources. However, all technological options present their own advantages and pitfalls. Besides,
energy policies and practices significantly impact the climate, environment, food and water production, and even mass population migrations.
I truly believe that renewable energy represents the clean, sustainable future, and is essential for decarbonizing our energy economy, and in particular, electric power generation. Impressively, renewables have now achieved a global installed capacity of over 2,500 GW that provide more than 25% of the world’s electricity production.1 But there is still a long, winding, and rocky road ahead for full transition to zero-carbon energy. Moreover, most renewables such as solar, wind, marine, and geothermal are un-dispatchable sources (i.e., power that is not always available at the time of demand) with inherently intermittent and variable power outputs, which—without storage—pose challenges for grid management. For renewables to grab the lion’s share in the global electricity mix demands major advances in energy storage materials and technologies as well as upgrades to our electric grid systems.
In that regard, much progress has been made in Li-ion batteries, which have revolutionized portable electronics and transportation. I am proud to note that the impactful work of ECS members has contributed significantly to this remarkable achievement, as recognized by the 2019 Nobel Prize in Chemistry awarded to our longtime members Drs. Whittingham, Goodenough, and Yoshino, and by many ECS awards to our distinguished members. But there is still more needed to further grow renewables and decarbonize the global energy economy.
(continued on next page)
FROM
From the President (continued from previous page)
Clearly, large-scale energy storage is indispensable for renewables and is a critical component of the clean-energy economy. Unfortunately, the world lags far behind in adequate storage capacity, sometimes leading to negative pricing. For example, to store even four hours of the 2,500 GW renewable capacity would require 10,000 GWh of storage. But only 192 GW has so far been installed globally, providing only 800 GWh of storage capacity.2 And only less than four GW of the 192 GW of installed capacity is electrochemical storage, while 95% is made up by pumped-hydro storage that is available only in very select geographic regions. In reality, the shortfall may even be worse, as the US Department of Energy considers 10–12 hours of storage duration as the target for grid applications. Such deficiency in storage also presents an opportunity for ECS members to have dramatic impact.
Naturally, chemical fuels with exceptionally high energy densities (e.g., 33,570 Wh/kg for liquid H2 compared to 380 Wh/kg of theoretical energy density for Li-ion batteries) offer opportunities for long-term and large-scale energy storage and conversion, especially for non-carbon fuels such as green hydrogen and ammonia that yield environmentally benign products. These are all areas of primary interest and expertise of ECS members, where we can continue to make impactful contributions toward sustainable solutions.
Although renewables have recently made impressive gains, fossil fuels continue to provide about 80% of the overall energy needs of the world and nearly two-thirds of our electricity production. In all likelihood, dramatic or disruptive shifts are not expected for the near future. Indeed, the US Energy Information Administration International Energy Outlook 2020 projects that fossil fuels will still be responsible for 68% of the global energy mix in 2050. So, in my view, summarily wishing away fossil fuels would be quite shortsighted. Hence, there is an urgent need to develop advanced fossil fuel–based power technologies that allow total carbon capture to achieve zero-carbon power. We also need to implement and enforce an appropriate “price” for carbon emissions across the globe to incentivize investment in scalable carbon capture technologies. Currently, carbon trading prices are less than 50% of the estimated cost of capturing it, which provides no economic incentive to capture CO2 emissions.
The United Nations Environment Programme 2019 Emissions Gap Report urges a nearly 50% reduction in emissions of greenhouse gases to 25 GtCO2e (i.e., CO2 equivalent) by 2030 to limit the temperature rise to below 1.5oC. This target would have required annual reductions of 7.6% until 2030 if we had started back in 2020, which we did not, and will now require 15.4% annual reductions in emissions to achieve the same goal if action can be taken by 2025. I hope the global community will collectively commit to jump-start dramatic reductions by 2025 during the COP27 meeting in Egypt in November 2022 (shortly after the time of this writing).
In the meantime, energy-related CO2 emissions into the atmosphere have steadily grown to over 36 GtCO2 annually to push CO2 levels above 410 ppmv in the atmosphere. To be sure, capturing 36 gigatons of CO2 every year poses unprecedented industrial challenges. By comparison, the largest industrial capacities the world has built so far are 4.1 Gt/yr for cement production and 1.5 Gt/yr for petrochemicals. Obviously, these capacities are dwarfed by the annual CO2 emissions. Hence, carbon capture under the current status quo will require massive industrial buildup at unprecedented scales.
Based on the global landscape presented above, I posit that we modify our current energy strategy, which is built largely on reducing or eliminating carbon from the input side of energy and electricity production, but with little or no regard to CO2 released into the atmosphere on the output side. I urge equal emphasis be placed on the output side of energy production and that we implement CO2 capture—especially at point sources such as coal- and natural gasfired power plants, and steel and cement manufacturing.
Given that combustion-based technologies with unabated emissions directly impact climate change, I also propose that we rapidly phase out air-based combustion of fossil fuels, and instead transition to oxygen-based conversion processes that negate the need for CO2 separation from flue streams and allow easy capture of CO2. This pathway may allow a smooth transition to achieve net-zero energy. In this regard, fuel cells offer efficient energy conversion and production of electricity from gaseous and solid fuels with full CO2 capture.
Pathways to commercializing energy technologies are typically risky and expensive. Economics frequently plays a decisive role in allocating resources and investments in new concepts toward a clean economy. However, deferred or missed opportunities in new technology concepts and investments may later cost us dearly in other ways. For example, between 2005 and 2019 the climbing cost of natural disasters linked to intensified climate change exceeded a total of $1.16 trillion in the United States alone.3
Although challenges to achieving sustainable energy are complex, I remain hopeful as solutions for such pressing societal challenges can only be guided and accomplished by science and innovation. Hence, the collective expertise and unique abilities of ECS members will be central to advancing sustainable solutions for many of these global grand challenges.
It is also clear that policies to address these pressing issues be built on science. Consequently, one of our responsibilities is to inform and educate the public and public officials to help implement sciencedriven policy decisions. We help accomplish this by publishing only high-quality, peer-reviewed work in our esteemed flagship journals, JES and JSS. Last year, we further expanded our journal portfolio by successfully launching two new titles, ECS Sensors Plus and ECS Advances. I strongly urge our members to support our journals with their high-quality work.
We also initiated collaborative efforts with industry and other professional organizations to develop education and training programs for a much-needed workforce skilled in energy storage technologies and materials, with an initial emphasis on Li-ion batteries. I call upon our members to contribute to such outreach efforts and devise new programs in their areas of expertise that further address global challenges.
In closing, transforming major segments of the global energy economy to clean and sustainable energy solutions requires a multidisciplinary, multi-pronged strategy as well as technological and resource diversity to ensure energy security while tackling climate change and global warming. Clearly, renewables are the cornerstone to achieving a sustainable future, but not without the availability of large-scale storage. In the meantime, transition will also require implementing carbon pricing as well as zero-carbon technologies for fossil fuels. I strongly encourage international collaborations and cooperation among our members to address these major global challenges. With our collective expertise and wisdom, I trust our ECS community will lead the way to further advance science and technology toward a sustainable future.
Turgut M. Gür ECS Presidenthttps://orcid.org/0000-0002-2218-4766
1. International Renewable Energy Agency (IRENA), Statistics Time Series
2. U.S. Department of Energy, DOE Global Energy Storage Database
3. A. B. Smith, “2018’s Billion Dollar Disasters in Context,” NOAA Climate.gov
With outstanding resolution and research-grade precision and accuracy, BCS-800 Series battery cyclers stand out for advanced applications including dQ/dV, HPC & EIS.
www.biologic.net
Shaping the future. Together.
Electrochromic materials (ECM) change color or transparency when an electric field is applied. Over the last 50 years, these materials have been developed for a variety of commercial applications including window coatings, displays, mirrors, and sensors. These applications benefit from color tunability, fast switching times, and low power use due to the memory effect exhibited by many ECM where the color is held without the need for constant power. There are several categories of ECM including conductive polymers, organic transition metal compounds, transitional metal oxides, and Prussian blue-like compounds. [1]
The interaction of materials with light ultimately depends on their population of electrons in energy levels which are influenced by the molecular environment. Electrons may absorb energy from light to jump to higher energy levels and release energy to relax down to a lower energy level. The key point is that the energy absorbed or released is of a fixed value that corresponds to the energy difference between levels. Some wavelengths of light are absorbed while others are not because they do not fit the levels present in the molecular environment. Color, as seen by the human eye, is a result of the wavelengths of light that are not absorbed (i.e. the wavelengths that are transmitted or reflected) by the material. In the case of ECM, the molecular environment is altered when voltage is applied in a way that the absorption characteristics change. ECM are often applied as thin films on transparent materials so the resulting color changes appear as a tint or change in transparency/opacity; such is the case with smart windows. [2]
As there are several categories of ECM, there are also different mechanisms for the color changes that they exhibit. The following examples illustrate some of the molecular changes that occur in common ECM:
Transition metal oxides like tungsten trioxide (WO3) change from pale yellow to intense blue when reduction occurs. An electron is gained and tungsten transitions from WIV to WV, this is accompanied by the intercalation of charge-balancing cations from the electrolyte (M+) or protons (H+).
Prussian Blue is a pigment used extensively in inks and lacquers that exhibits electrochromic behavior. This coordination compound consists of cationic iron centers with anionic cyanide ligands. The iron centers are of mixed valence meaning that the iron in the structure exists as FeIII and FeII. Intervalence charge transfer between FeIII and FeII absorbs orange-red light,
giving rise to the typical blue color. When the majority of iron is in the reduced Fell state this charge transfer process is eliminated and the material is colorless. [3]
Passengers with the window seat traveling on Boeing’s 787 Dreamliner aircraft (image 1) can experience the colorchanging properties of ECM. Window shades have been replaced with dimmable windows that offer several levels of shading. This gives passengers the possibility to watch the view from their windows with their desired level of brightness, as opposed to the binary “open or closed“ window shades. Dimmable windows are also used in modern workspaces to improve the health and productivity of employees by reducing headaches and eyestrain and allowing optimal natural light levels that help workers feel energized [4]. Dimmable windows deliver significant energy and cost savings by blocking solar radiation and thus reducing the need for air conditioning.
Image 1: Electrochromic dimmable windows in the Boeing 787 Dreamliner.
Currently, researchers are investigating ways to derive more sustainability benefits from ECMs through the development of electrochromic energy storage (EES) devices. The EES concept integrates the smart color-switching functionality of ECMs with battery or supercapacitor-based energy storage. Thin-film batteries share structures and characteristics with ECM devices, for example, the intercalation of cations that accompanies color-switching reduction reactions is reminiscent of Li-ion batteries. Energy could be stored within the darkened state of an electrochromic window during the daytime to be used for electricity when the sun goes down [5]. A recent article published in ACS Applied Energy Materials describes an EES system based on nickel-carbonate-hydroxide (NHC) with high optical contrast in the colored/charged state that becomes colorless upon discharge. The NHC film employed has a unique structure made up of a dense underlayer with a porous nanowire surface that enables capacitive charge storage [6].
Evaluation and characterization of ECM is done on the
laboratory scale using a combination of electrochemical and spectroscopic techniques. Since the primary applications for these materials are either displays, or shading/ blocking light, there is a focus on the spectroscopic behavior as well as the visual appearance. When the ECM is used to display information the wavelength for the colored state of the ECM is extremely important. Human eyes are not equally sensitive to all colors, they are most sensitive to green. [1] Thus, ECM used in display applications are tested for the reliability of their coloring. For most applications is it also desirable to have an ECM that can reliably switch from bleached to colored state and back thousands of times; for window glazing the expectation is 40 000 switching cycles and a 20 year lifetime. This criterion is quantified as the cycle life.
For applications where the ECM is used in a large-scale device, the electrical resistance of the ECM and the overall setup is important. On the square-meter scale (i.e. in the case of windows) there can be a significant voltage drop from the electrical contacts and across the device. This can lead to slow or uneven coloration and an increase in the power required to accomplish the color change. Electrochemical impedance spectroscopy is used to predict how resistances within the material and the device will affect performance. [7]
The EES systems mentioned above introduce new evaluation criteria related to the material‘s ability to store charge. In general, the relevant evaluation parameters depend on the intended application for the ECM.[1] Several common parameters are listed and described in Table 1.
Electrochromic devices are already in use in daily life, often going unnoticed. They reduce annoying glare in automobile rearview mirrors, tint office windows, and frost glass panels for privacy in bedrooms and bathrooms. New applications extend beyond comfort and look towards sustainability as electrochromic devices strive to offer energy savings and perhaps even new options for energy storage. The future of electrochromic materials is bright, even when that means being “dim”.
[1] Kraft, A. Electrochromism: a fascinating branch of electrochemistry. ChemTexts. 2019. 5(1), 1-18. doi: 10.1007/s40828-018-0076-x.
[2] Shchegolkov, A.V.; et al. A Brief Overview of Electrochromic Materials and Related Devices: A Nanostructured Materials Perspective. Nanomaterials. 2021, 11, 2376-2408. doi: 10.3390/nano11092376.
[3] Mortimer, R.J. Switching colors with electricity. American Scientist. 2013. 101(1) doi: 10.1511/2013.100.38.
[4] Hedge, A. Daylight and the workplace study https://view.com/sites/default/files/ documents/research-brief-daylight-and-the-workplace.pdf
[5] Yang, P.; et al. Electrochromic energy storage devices. Materials Today. 2016. 19(7) 394-402. doi: 1-.1016/j.mattod.2015.11.007.
[6] Yin, X. Large-scale color-changing thin film energy storage device with high optical contrast and energy storage capacity. ACS Appl. Energy Mater. 2018, 1 1658–1663. doi: 10.1021/acsaem.8b00120
[7] Mohanadas, D. et al. Bifunctional ternary manganese oxide/vanadium oxide/ reduced graphene oxide as electrochromic asymmetric supercapacitor. Ceramics International. 2021, 47(24) 34529-34537. doi: 10.1016/j.ceramint.2021.08.368.
[8] Hassab, S. et al. A new standard method to calculate electrochromic switching time. Sol. Energy. 2018, 185 54-60. doi: 10.1016/j.solmat.2018.04.031.
[9] Lampert, C. et al. Durability evaluation of electrochromic devices – an industry perspective. Sol. Energy. 1999, 56 449-463. doi: 10.1016/S0927-0248(98)00185-8.
[10] Liu, Q. et al. Charge-transfer kinetics and cyclic properties of inorganic all-solid-state electrochromic device with remarkably improved optical memory. Sol. Energy, 2018. 174 545-553. Doi: 10.1016/jsol.mat.2017.09.012.
Coloration efficiency (CE)
Contrast ratio (CR)
The ratio of the change in optical density (OD) at a specified wavelength to the charge (Q) passed. Ideally, the CE is high corresponding to a large color contrast for little charge input. The CE value indicates the power consumption levels for the electrochromic device.
The ratio of light transmission (TM) in the bleached state to the TM in the colored state, at a specified wavelength. Ideally, the CR is high at wavelengths (λ) relevant to the application.
The transmission response at a fixed wavelength is measured in the colored and bleached states to determine the OD. Simultaneously, the charge (Q) passed during color switching is measured.
The transmission response at the wavelength of interest is monitored in the colored and bleached states to calculate the CR.
[1]
[1]
Switching time
Quantification of the time required to switch between colored and bleached states. This parameter is heavily influenced by the nature of the ECM and also by the test setup. The switching time measured at the laboratory scale may not be representative of the device in a large area (i.e. m2) application.
Cycle life Durability assessment of the ECM and device over typical lifetime conditions. Significant changes in switching speed, light transmission, visual appearance (i.e. inhomogeneity) throughout the lifetime test indicate that the device may not have acceptable performance in the target application.
Open circuit optical memory
Table 1:
Measurement of the device’s ability to maintain a defined state (i.e. colored or bleached) without applied power. Generally, the ECM will spontaneously bleach over time due to leakage currents or thermal changes. Having a high optical memory is desirable because this translates to low power consumption.
The transmission response at a fixed wavelength is monitored during applied potential pulses with varied pulse lengths. A plot of contrast vs. pulse length is modeled with the first-order differential equation to determine τ and ΔTmax
Performance parameters (i.e. contrast ratio, switching time), and visual appearance are monitored during an accelerated aging test protocol that involves subjecting the device to thousands of switching cycles in variable conditions (temperature, light exposure, humidity)
The desired state (generally colored or darkened) is applied and the power source is then removed (the system is put to open circuit conditions). Transmission and open circuit potential are monitored over time.
[1.8]
[1.9]
Parameters used for characterization and evaluation of electrochromic materials and devices.
Biographical sketches and candidacy statements of the nominated candidates for the annual election of ECS officers.
Gerardine (Gerri) Botte is Professor and the Whitacre Chair in Chemical Engineering at Texas Tech University (TTU), and the Founding Director of the National Science Foundation Engineering Research Center for Advancing Sustainable and Distributed Fertilizer Production (CASFER). A visionary and recognized leader in electrochemical science and technology, she has over 24 years of experience in the fundamental understanding and development of electrochemical processes as they relate to the intersection of energy, water, and food sustainability. In 2014, she was named Fellow of The Electrochemical Society for her contributions and innovation in electrochemical processes and engineering. Prior to TTU, Botte was University Distinguished Professor (lifetime award) and Russ Professor of Chemical and Biomolecular Engineering at Ohio University, Founder and Director of the Center for Electrochemical Engineering Research, and Founder and Director of the National Science Foundation Consortium for Electrochemical Processes and Technology—an Industry University Cooperative Research Center. She has 207 publications including 61 granted patents. An entrepreneur, Botte has been involved in the commercialization of technologies, and founded and co-founded companies. She received her PhD in 2000 (under Ralph White’s direction) and ME in 1998, both in Chemical Engineering from the University of South Carolina. Prior to graduate school, Botte worked in a petrochemical plant as a process engineer involved in the production of fertilizers and polymers. Botte completed a BS in Chemical Engineering at the Universidad de Carabobo (Venezuela) in 1994.
An active ECS member since 1998, Botte has served in leadership roles that include member of the Board of Directors and Executive Committee; Chair, ECS Industrial Electrochemistry and Electrochemical Engineering (IEEE) Division; and member, Interdisciplinary Science and Technology Subcommittee, Honors and Awards Committee, and ECS Fellows Award Committee. She has contributed articles to Interface, organized and co-organized nine Society symposia, and co-edited the corresponding ECS Transactions volumes,
Vimal Chaitanya is currently Professor and Director of Manufacturing Systems and Research Programs at New Mexico State University (NMSU). Previously, he served as the Vice President for Research (VPR) of NMSU for over 10 years. As VPR, he was responsible for the university’s entire research enterprise. The average yearly R&D expenditure for externally sponsored research was $142 million during his tenure as VPR. About 250 faculty and staff reported to him directly or indirectly. Prior to becoming VPR at NMSU, he founded and directed a state recognized center of excellence at University of Central Florida (UCF) with its own faculty lines and budget. He also developed and implemented dedicated MS and PhD programs in materials science and engineering.
Dr. Chaitanya has over 40 years of experience in research, which includes corrosion, hot corrosion, oxidation, solid state device processing, chemical mechanical planarization, additive manufacturing, and the food-energy-water nexus. As a faculty member, he has mentored many undergraduate and about 30 graduate students. He has received the Researcher of the Year Award five times, Teaching Award twice, UCF Leadership Award (1999), UCF Excellence in Professional Service Award (2002), and NMSU Synergy Faculty Leadership Award (2022).
As a student, he attended his first ECS meeting in 1982 and started contributing as a young faculty member with his students from 1986. He has been a regular attendee and member of ECS since 1994. Over the years, he has served on the Education Committee, Awards and Honors Committee, and Nomination Committee, while chairing various sub-committees, notably the ECS Summer Fellowship Subcommittee. He also served as lead organizer of the General Student Poster Session for several years and as organizer of various symposia. He has served in the ECS Dielectric Science and Technology Division as Chair and as a member of the ECS Board of Directors.
I am honored to be nominated as a candidate for the 3rd Vice President of The Electrochemical Society. If elected, I look forward to serving the interests of the Society and its members. Today, we
Francis D’Souza is currently Regents Professor of Chemistry and Materials Science and Engineering at the University of North Texas (UNT) and is also part of UNT’s Applied Materials and Manufacturing Processing Institute. Prior to joining UNT in 2011, he was Professor of Chemistry at Wichita State University (WSU). He received a PhD from the Indian Institute of Science, and held postdoctoral positions at the University of Houston and the Université de Bourgogne.
Dr. D’Souza’s research covers a wide area of chemistry, nanophotonics, electrochemistry, and materials science. Principal research interests include supra and nanomolecular chemistry of photosensitizercarbon nanomaterials, advanced functional materials for light energy harvesting and photovoltaics, electrochemical and photochemical sensors and catalysts, and nanocomposite hybrid materials for energy storage and utilization. Dr. D’Souza has authored or co-authored over 475 publications, given over 400 conference talks, and edited 10 Handbooks on Carbon Nanomaterials, resulting in over 21,000 citations with a cumulative h-index of 74.
Honors and awards include Fellow of The Electrochemistry Society, Fellow of the Royal Society of Chemistry, Fulbright Specialist Scholar, ACS-DFW Section Doherty Research Award, Chemical Research Society of India Medal, UNTResearch Leadership Award, UNT-Regents Professor, UNT-Toulouse Scholar Award, GIAN Fellow of Government of India, UNTDistinguished Professor, Japan Society for the Promotion of Science Fellow, and WSU Excellence in Research Award.
As an active member of The Electrochemical Society since 1993, Dr. D’Souza has served ECS at a number of levels. He was a member of the ECS Board of Directors from 2004 to 2008. He previously served as Chair, Vice Chair, Secretary, and Treasurer of the ECS Nanocarbon Division and is currently a Member at Large of that division. As Chair of the ECS Nanocarbon Division, he was instrumental in establishing and securing endowments for both the Smalley Research Award and the Young Investigator Awards. He continues to serve either as chair or member of several Society-level committees, including the Honors and Awards Committee, the Meeting Subcommittee, and the Fellow
(continued from previous page)
among others. She started the ECS IE&EE Division Outreach Program. Its first activity took place at the 210th ECS Meeting in Cancun in 2006; since then, it has taken place 16 times with at least 847 participants at different ECS meetings.
One of the things that makes me most excited about electrochemical and solid state science and technology is that it is a core platform with applications in different aspects of our lives. While exciting, there is still a lag in education about what electrochemical technologies can do. I believe that ECS, being the premier solid state and electrochemical science and technology society in the world, will lead a paradigm change mitigating this education gap while enabling sustainable education in the field. If elected, I will lead ECS to expand into programs that explain to different audiences the importance and fundamental principles of electrochemistry. For example, programs targeted to the next generation of scholars (STEM), industry, policy makers, and investors. We could find opportunities for all this during the biannual meetings. Outreach programs can become a continuous event in our biannual meetings to attract the next generation to electrochemical science. Such programs could include our ECS Student Chapters. Forums and sessions that discuss commercialization aspects of electrochemical-based technologies can become part of our programs and help reach out to funding agencies, policy makers, and investors. I will help facilitate joint collaborations between ECS, federal funding agencies, and industry to organize and sponsor symposia on topics regarding frontiers and opportunities for electrochemical technologies.
I am fully committed to, and supportive of, the ECS Free the Science effort. If elected, I will work with ECS and members to raise the resources to minimize the cost burden of open access. It is important to continue attracting young authors to submit their best papers to our journals and to support rising stars in electrochemical and solid state science. If elected, I will help generate resources to increase the number of awards for young authors. In addition, I think it is essential to consider dissemination of knowledge to attract the next generation and to engage industrial and policy makers. In that respect, education-based apps can play a significant role. I will seek partnerships with our members and industrial sponsors to develop such opportunities.
I applaud the ECS Diversity Statement. The symposium on diversity and inclusion at the 236th ECS Meeting in Atlanta is an example of many other initiatives that can
continue. I will lead ECS to implement best practices in diversity and inclusion and seek collaboration with other professional organizations to support these efforts.
ECS is an integral part of my professional career. It is my family, where I have been accepted and welcomed since I was a student, and where I interact with many distinguished scientists from all over the world who today are my peers and friends. It is an honor to be nominated for this position and I look forward to growing the ECS family and serving all of you.
Vimal Chaitanya (continued from previous page)face global challenges and opportunities in areas such as the water-energy nexus, solid state device design and fabrication, green manufacturing, material performance in harsh environments, etc. With its multiple divisions, global footprint, and talented membership, The Electrochemical Society is poised to contribute significantly. ECS has a history of shaping the future of science and scientists, and I will continue this legacy by following the footsteps of our visionary leadership and invest in it.
There is no better way to invest in the future of science and technology than to invest in the students, postdocs, and young scientists. If elected, I will bring my 20 years of administrative experience, zeal for research, and enthusiasm for STEM education to bear on the success of engaging young as well as budding scientists with our “member-driven” society. I will also work to create more opportunity for industries and industrial members to get directly involved with our academic researchers to create research and education frontiers that address the critical needs. In this equation, participation of federal funding agencies is important. If elected, I will seek to create forums for the participation of funding agencies in our meetings. I believe The Electrochemical Society can play a pioneering role in bringing all the stakeholders together to identify and resolve research challenges, develop an educated workforce, and work toward building a sustainable ecosystem.
COVID-19 pandemic has changed our perspective and challenged the way we do business. We learned to effectively communicate with each other, teach, and even perform experimental research remotely. ECS responded to the COVID challenge by hosting online and hybrid meetings, which broke all previous records for meeting participation. Various ECS committees continued their work unhindered through online meetings and digital media. This would not have been possible without the commitment of ECS membership and the dedication of ECS staff. I will work tirelessly
to search for and help implement practices in the new era which serve our needs.
I can adequately represent both wet electrochemistry and solid state sciences, having worked in both areas. I have the experience of working with faculty researchers from various disciplines to create opportunities and develop partnerships with industries and federal labs. I will work with all divisions, local sections, student chapters, and corporate affiliate members to develop programs and forums that can be locally disseminated to the public to bring awareness of the importance of electrochemistry and solid state sciences in daily life and create interest among the younger generations to pursue their education and careers in this field.
Finally, as a vice president of ECS, I will be committed to work together with all members, elected officers, and professional staff to identify and implement initiatives for sustained growth of ECS and to enable ECS members to reach their professional goals.
D’SouzaSubcommittee, as well as the Acheson, Bard, Callinan, Haddon, Smalley, and Wagner Award Subcommittees. To date, he has coorganized over 40 symposia for the fall and spring biannual meetings of the Society.
Dr. D’Souza has also served for the last 10 years as Technical and Associate Editor of the ECS Journal of Solid State Science and Technology.
It is my great honor to be one of the two candidates for the 3rd Vice President of The Electrochemical Society. Electrochemical science and technology now play a vital role in our modern life, several examples of which are in the production of renewable energy, energy storage, corrosion prevention, pollution control, sensors and biosensors, and greenhouse gas reduction, to name a few. Electrochemical science is also at the forefront of large-scale solutions to meet the challenges of climate change and global warming. If elected to a leadership role in ECS, my mission will be to establish stronger collaborative relationships among scientific communities, promote young scientists, help develop clean energy technologies, and bridge the gap between academia, industry, and policymakers, while also promoting equity, diversity, and inclusion at every level of the Society. I will continue to serve ECS and its members constructively, collaboratively, and respectfully, and look forward to the possibility of doing so in a higher leadership role in the future.
From October 9 to 13, 2022, the 242nd ECS Meeting—the Society’s second in-person and online meeting—convened in Atlanta, GA, with 2,619 participants (2,588 registrants and 31 non-technical guests) from 65 countries. The meeting encompassed 48 symposia with 441 sessions. A total of 2,628 abstracts were accepted with 1,869 oral talks, 616 posters, and 312 digital presentations, including 430 invited talks and 35 ECS award and keynote talks. Students presented 1,092 abstracts, with 762 oral talks and 366 posters. Digital content was uploaded by 269 digital presenters and included 312 digital presentation files, of which 109 were digital student presentations. (Recordings of the ECS Awards and Recognition Ceremony and Plenary Presentation are available on the ECS YouTube Channel at https://www.youtube.com/user/ ECS1902.)
More than 500 members came together at the second ECS Members Reception which preceded the Opening Ceremony on Sunday evening. Colleagues and new peers networked while enjoying a raffle, food, and open bar. Several members received prize giveaways as part of attending:
• Yudong Wang, University of Louisiana at Lafayette – ECS Lifetime Membership
• Marc Bertrand, Université de Montréal – $250 Amazon Gift Card
• Elsa Briqueleur, Université de Montréal – $250 Amazon Gift Card
•
The event was a success and will be part of the 243rd ECS Meeting in Boston, MA.
this was only the second in-person ECS Awards and Recognition Ceremony since 2019, Turgut explained that award winners from previous years would be acknowledged as well as 2022 award winners.
Turgut presented the 2022 Leadership Circle Award to Duracell Corporation (represented by Dan Gibbons, Principal Engineer and Research Fellow) for their 65 years of institutional membership and dedication to ECS.
Next, Turgut awarded the 2021 Norman Hackerman Young Author Award to Philip Minnmann and Simon Burkhardt from Justus Liebig Universität, Giessen-Institute of Physical Chemistry, for their JES article, “Quantifying the Impact of Charge Transport Bottlenecks in Composite Cathodes of All-Solid-State Batteries.” The award is for the best paper published in the Journal of The Electrochemical Society by a young author or co-authors in the preceding volume year.
Following introductory remarks, Turgut opened the Awards and Recognition Ceremony to celebrate the achievements of today’s greatest researchers in electrochemistry and solid state science. As
The 2021 Bruce Deal & Andy Grove Award for outstanding work published by a young author in JSS went to Franz Selbmann from the FraunhoferInstitute, for his paper, “LowTemperature Parylene-Based Adhesive Bonding Technology for 150 and 200 mm Wafers for Fully Biocompatible and Highly Reliable Microsystems.”
Turgut invited ECS Senior Vice President Gerardine (Gerri) Botte to recognize the next recipients. She thanked division chairs whose terms had ended for their dedicated service:
• Stanko Brankovic, Electrodeposition Division, 2017–2019
• Masayuki Itagaki, Corrosion Division, 2018–2020
• Petr Vanysek, Physical and Analytical Electrochemistry Division, 2019–2021
• Philippe Vereecken, Electrodeposition Division, 2019–2021
• Jakoah Brgoch, Luminescence and Display Materials Division, 2019–2021
Gerri then thanked Gessie Brisard, ECS Treasurer from 2018 to 2022, for her inspired stewardship of ECS’s finances, and 2021–2022 ECS President Eric Wachsman for his visionary leadership and for helping the Society emerge from the COVID-19 shutdown to better days ahead.
Gerri welcomed multiple years of new Fellows of The Electrochemical Society, starting with the members of the Class of 2020 present at the meeting:
• Rodney Borup, for seminal contributions to the understanding of polymer electrolyte fuel cell durability.
• Andrew Herring, for creative contributions to the science and technology of polymer electrolyte membranes.
• Stefano Passerini, in recognition of his outstanding contributions to the field of electrochemical energy storage.
• Venkataraman Thangadurai, for major contributions to the development of highly conductive solid electrolytes
• Jie Xiao, for her scientific and technical contributions in emerging electrochemical energy storage.
• Gleb Yushin, for his development and mass commercialization of low surface area size-preserving nanocomposites.
She then introduced the members of the 2021 Class of Fellows present at the meeting:
• Anja Boisen for her groundbreaking, impactful, and pioneering contributions to the field of nanomechanical sensors, and for being a role model who inspires women around the world.
• Stanko Brankovic for fundamental contributions to electrochemical material science.
• Douglas Hansen in recognition of distinguished contributions to the advancement of corrosion, biochemical, and electrochemical sciences and engineering.
• Jagjit Nanda for pioneering work on solid state batteries, Liion batteries, flow batteries, and service to the Society.
• Xiao-Dong Zhou for outstanding scientific and technological contributions to fuel cells, electrolyzers, and batteries, and for his dedicated service to the Society.
Gerri ended by recognizing the 2022 Class of Fellows:
• Michel Armand in recognition of his pioneering and outstanding contributions to the field of electrochemical energy storage.
• Perla Balbuena for contributions to the understanding of electron and ion transport mechanisms driving the formation of solid-electrolyte interphases.
• Gerbrand Ceder for pioneering work on designing and understanding battery materials using first principles computational modeling.
• Wilson Chiu for his contributions to fundamental understanding of 3D electrochemical and transport processes.
• Andrew Hoff in recognition of contributions to the advancement of science and technology in the areas of solid state electronics and bioengineering.
• Gao Liu for contributions to the fundamental understanding of the functions of the polymer binder in composite electrodes.
• Brett Lucht for outstanding contributions to the research and development of non-aqueous electrolytes for electrochemical storage devices.
• Janine Mauzeroll for the development of next generation electroanalytical tools for biological and corrosion research. (continued on next page)
(continued from previous page)
• Nguyen Minh for his impactful contributions to materials engineering, design, manufacturing processes, and systems.
• Deborah Myers for impactful and sustained contributions to electrochemistry and electrocatalysis.
• James Noël for impactful and sustained contributions in the areas of materials and corrosion science.
• Elizabeth Podlaha-Murphy for her seminal contributions in understanding the electrodeposition processes of alloy and composite materials.
• Vijay Ramani for fundamental and applied research contributions at the interface of electrochemical energy conversion and storage and materials science.
• Yasuhiro Shimizu for his groundbreaking, impactful, and pioneering contributions to the field of semiconductor gas sensors.
Turgut thanked Gerri and announced the 2022-2023 ECS Toyota Young Investigator Fellowship recipients. Each Young Investigator Fellow receives a one-year, $50,000 grant to pursue research in batteries, fuel cells and hydrogen, and future sustainable technologies. With this year’s recipients, we celebrate that the ECSToyota partnership has now provided over $1.4 million of support for young professionals and scholars! He noted that applications for the next Young Investigator Fellowships are due by January 31, 2023. The 2022-2023 ECS Toyota Young Investigator Fellows present their research at the 244th ECS Meeting in Gothenburg, Sweden, in October 2023. They are:
• Betar M. Gallant, Massachusetts Institute of Technology
• Yayuan Liu, Johns Hopkins University
• Jacob S. Spendelow, Los Alamos National Laboratory
• William Tarpeh, Stanford University
Turgut introduced the winners of the 2020 and 2022 Charles W. Tobias Young Investigator Awards which recognize outstanding scientific and/or engineering work in fundamental or applied electrochemistry or solid state science and technology by a young scientist or engineer. Bryan McCloskey, University of California, Berkeley, received the 2020 award for quantitative insights into oxygen activity and degradation mechanisms in high-energy batteries. He presented his award talk, “Understanding Reactivity at Electrode-Electrolyte Interfaces in Li-O2 and Li-ion Batteries” at the digital PRiME meeting in October 2020. The 2022 recipient is Fikile Brushett, Massachusetts Institute of Technology, for pioneering advances in understanding and controlling the fundamental processes that govern performance, cost, and lifetime of the next generation of flow electrochemical systems for energy storage and conversion. He presented his award talk, “Advancing Porous Electrodes for Electrochemical Systems” at the meeting.
Turgut presented the 2021 Olin Palladium Award to Gerald Frankel, The Ohio State University, for sustained and seminal contributions in corrosion science. Prof. Frankel presented his talk, “Pitting Corrosion Retrospective” at the digital 240th ECS Meeting in October 2021.
Two winners of the Edward Goodrich Acheson Award were honored. Esther Takeuchi, Stony Brook University, received the 2020 Acheson Award for inventive contributions to the Li/SVO battery, an enabling electrochemical technology discovery which has saved millions of human lives. Prof. Takeuchi presented her award talk, “Investigation of Batteries over Multiple and Length and Time Scales”, at the 240th ECS Meeting in October 2021. The 2022 Acheson Award was presented to Yue Kuo of Texas A&M, for exceptional contributions to solid state science and technology, and for outstanding service to The Electrochemical Society. Prof. Kuo delivered his award talk, “Exploration of Amorphous Thin Film Electronics,” at the meeting.
Winners of the 242nd ECS Meeting Z01 General Student Poster Session Awards, as well as Society, division, and section awards, were recognized throughout the course of the meeting, including some from years when in-person meetings were not held. These 2022 division awards were given at the meeting:
• Battery Division Early Career Award Sponsored by Neware Technology Limited: Feng Lin, Virginia Tech
• Battery Division Postdoctoral Associate Research Award Sponsored by MTI Corporation and the Jiang Family Foundation: Paul Coman, University of South Carolina
• Battery Division Postdoctoral Associate Research Award Sponsored by MTI Corporation and the Jiang Family Foundation: Alexis Maurel, University of Texas at El Paso
• Battery Division Research Award: Bor Yann Liaw, Idaho National Laboratory
• Battery Division Research Award: Atsuo Yamada, University of Tokyo
• Battery Division Student Research Award Sponsored by Mercedes-Benz Research & Development: Singyuk Hou, University of Maryland, College Park
• Battery Division Student Research Award Sponsored by Mercedes-Benz Research & Development: John Holoubek, University of California, San Diego
• Corrosion Division H. H. Uhlig Award: Mark Orazem, University of Florida
• Corrosion Division Morris Cohen Graduate Student Award: Mariko Kadowaki, National Institute for Materials Science
• Corrosion Division Rusty Award for Mid-Career Excellence: David Bastidas, The University of Akron
• Electrodeposition Division Early Career Investigator Award: Fudong Han, Rensselaer Polytechnic Institute
• Electrodeposition Division Research Award: Nikolay Dimitrov, Binghamton University (SUNY)
• Energy Technology Division Walter Van Schalkwijk Award in Sustainable Energy Technology: Katherine Ayers, Nel Hydrogen US
• High Temperature Materials Division J. B. Wagner, Jr. Young Investigator Award: Liangbing Hu, University of Maryland, College Park
• High Temperature Materials Division Outstanding Achievement Award: Scott A. Barnett, Northwestern University
• Industrial Electrochemistry and Electrochemical Engineering Division H. H. Dow Memorial Student Achievement Award: Arghya Patra, University of Illinois Urbana-Champaign
• Luminescence and Display Materials Division Outstanding Achievement Award: Kailash Mishra, OSRAM Sylvania Lighting Research Center (retired)
• Physical and Analytical Electrochemistry Division Max Bredig Award in Molten Salt and Ionic Liquid Chemistry: Tom Welton, Imperial College London
• Sensor Division Outstanding Achievement Award: Anja Boisen, Danmarks Tekniske Universitet
• Section awards from 2021 and 2022 were given at the meeting:
• 2022 Europe Section Alessandro Volta Medal: Jerry Barker, Faradion Limited
• 2022 Europe Section Alessandro Volta Medal: Stefano Passerini, Helmholtz Institute Ulm
• 2021 Europe Section Heinz Gerischer Award: D. Noel Buckley, University of Limerick
Christopher Jannuzzi, ECS CEO and Executive Director, provided the meeting’s introductory remarks. He welcomed event participants in Atlanta and those participating via video stream. Chris gave an overview of the week ahead and thanked symposia sponsors and exhibitors, meeting attendees, digital participants, volunteers, and staff. Chris noted the critical support that divisions and sections provide to students and early career attendees through their travel funding programs.
Turgut Gür, ECS President, took the floor and welcomed attendees and participants to the second in-person meeting since the pandemic. He described the unprecedented challenges facing the world that ECS members are playing a significant role in combatting, especially in the area of the climate change crisis. Turgut announced the board’s recent approval of the ECS Statement on Climate Change With this statement, ECS pledges to advocate for integrating science
in the implementation of effective strategies and sustainable solutions to battle climate change.
Turgut introduced the speaker delivering the 242nd ECS Meeting Lecture, M. Stanley Whittington, Distinguished Professor of Chemistry at the University of Binghamton of the State University of New York. Just before appearing at the last ECS meeting in Atlanta, Stanley learned that he, John Goodenough, and Akira Yoshino had received the 2019 Nobel Prize in Chemistry for the development of lithium-ion batteries. Turgut said how lucky we were to celebrate Stanley’s award with him at that meeting. He then stressed how much had happened in the world since that meeting, most of which underscores just how critical Stanley’s work is today, and will be for the foreseeable future and beyond.
Prof. Whittingham presented “The Critical Role of Energy Storage in the Electric Economy and Overcoming Climate Change” to a rapt audience. After a discussion of the history of the development of electric transportation and the Li-ion battery, he described the need for researchers to find new materials to make the next generation batteries that are lower cost, have a stronger domestic supply chain, and are safer. Prof. Whittingham reviewed some of the recent research trends that address these challenges. A Q&A session moderated by Jie Xiao followed the talk.
Jie
(continued from previous page)
The Z01 General Student Poster Session presented 112 posters in person and digitally. The following won awards:
1st Place: $1,500 cash award
Emmanuel Peprah-Yamoah, East Tennessee State University Z01-2392 “Nitrogen-Doped and Phosphorus-Doped Carbon Fiber Ultramicroelectrodes As Electrochemical Sensors for Detection of Hydrogen Peroxide”
2nd Place: $1,000 cash award
Ashley Amalia David, Florida State University Z01-2380 “Analyzing the Soret Coefficient Using Time Resolved Fourier Transform Infrared Spectroscopy (FTIR)”
Thank you to the judges of the 242nd ECS Meeting Z01 General Student Poster Session.
In person:
• Stefan De Gendt, KU Leuven
• Uroš Cvelbar, Jozef Stefan Institute
• Jason Keleher, Lewis University
• John Staser, The Ohio State University
Virtual judges:
• David Hall, University of Cambridge
• Leah Rynearson, University of Rhode Island
• Bhuvana Vukkum, North Carolina State University
• Thad Druffel, University of Louisville
• William Mustain, University of South Carolina
• Sadman Sakib, McMaster University
Lucky visitors to the ECS Exhibit Booth won raffle prizes:
• ECS Lifetime Membership: Jiseon Choi, Korea Institute of Machinery & Materials
• Complimentary 2023 ECS Biannual Meeting Registration: Samantha Gateman, Western University
• ECS Monograph, LaQue’s Handbook on Marine Corrosion, 2nd Edition: Maitri Uppaluri, University of Texas at Austin
• 242nd ECS Meeting Exhibitor Raffle Basket: Current Chemicals
ECS extends its deepest gratitude to the general meeting sponsors for generously supporting the 242nd ECS Meeting. Thank you to the meeting symposia sponsors and exhibitors, whose support and participation contributed directly to the success of the meeting, for developing the tools and equipment driving scientific advancement, and for sharing your innovations with the electrochemical and solid state communities.
Meeting exhibitors displayed their products and services in the Exhibit Hall and Digital Exhibitor and Vendor Guide (DEVguide). Viewers can scan the latest electrochemistry and solid state science products with ease as the DEVguide navigates seamlessly on any platform.
SILVER
• American Elements
• Comsol, Inc.
• Metrohm USA, Inc.
• Pine Research Instrumentation
• Wildcat Discovery Technologies
BRONZE
• SK on
Almost 300 students and early-career professionals mingled in a relaxed setting and enjoyed light hors d’oeuvres and refreshments at the Student Mixer. Pine Research Instrumentation and Wildcat Discover Technologies sponsored the event.
•
•
• Gelest
• Case Western Reserve University (Case School of Engineering)
• Office of Naval Research
• QuantumScape
Students
SILVER
• Corning
• TEL
• GlobalFoundries BRONZE
• Applied Materials
• ASM
• Daicel
• Energy Assurance
• Lam Research
• LG Energy Solution
• Mitsui Kinzoku
• Sila
• U.S. Army Research Office
• Air Liquide Advanced Materials
• Arbin Instruments
• Covalent Metrology
• Lam Research
• Neware Technology Limited
• Nissan Group of North America
• Tecnochimica
• Admiral Instruments
• Advanced Cell Engineering
• Ametek-Scientific Instruments (PAR/Solatron)
• Arbin Instruments
• BASi
• BioLogic USA
• Calumix Technologies
• Comsol, Inc.
• Current Chemicals
• Cyclikal, LLC
• DataPhysics Instruments USA Corp.
• De Nora
• Department of Homeland Security Science and Technology Directorate
• ECS - The Electrochemical Society
• Gamry Instruments
• Hiden Analytical
• IOP Publishing
• IVIUM Technologies
• Malvern Panalytical Metrohm USA
• MTI Corporation
• OCI Vacuum Microengineering
• PalmSens BV
• Pamarco
• Pine Research Instrumentation
• Royal Society of Chemistry
• Scribner Associates
• SH Scientific Corporation
• Spectro Inlets ApS
• TA Instruments
• UL Research Institutes
• VSPARTICLE B.V.
• Wildcat Discovery Technologies
• Wiley
• Xenocs
• Zurich Instruments USA
JES is the flagship journal of The Electrochemical Society. Published continuously from 1902 to the present, JES remains one of the most highly cited journals in electrochemistry and solid state science and technology.
ECS Sensors Plus is a one-stop shop journal for sensors. This multidisciplinary, Gold Open Access journal provides an international platform for publishing high-quality impactful articles and promoting scholarly communication and interactions among scientists, engineers, and technologists whose primary interests focus on materials, structures, properties, performance, and characterization of sensing and detection devices and systems, including sensor arrays and networks.
ECS Advances is a multidisciplinary, Gold Open Access forum of peer-reviewed, high-quality content covering all technical areas supported by the Society. ECS Advances publishes full-length original work, brief communicationstyle papers, perspectives, review articles, and special issues.
As we entered the fall of 2022, an eerie trend in the publications community seemed to be impacting ECS and every publisher of peer-reviewed content, regardless of the subject matter. Our team of peer reviewers, which had been moving at a much slower pace over the last several months, appeared to be slowing even more, negatively impacting our peer review timelines. In some rare cases, our editorial teams struggled for weeks to garner the support of reviewers who turned down our requests to review manuscript submissions. Even our most committed reviewers declined to review manuscripts in their areas of expertise, citing lack of capacity to complete the reviews. Conversations with other organizations revealed that this trend was being experienced throughout the publications community.
This resulted in a call to action to activate and strengthen our pool of peer reviewers at the 242nd ECS Meeting. By engaging directly with meeting participants, we actively recruited postdoctoral ECS community members to review their fellow researchers’ manuscripts. We emphasized and elevated the message that all members of our constituency should be committed to the peer-review process. The efforts of peer reviewers support their own journey to publication.
Thus, where ECS and its constituents share the mission to advance science and technology by disseminating research, successfully disseminating this research depends on our constituents’ engagement and support of one another by reviewing the manuscripts of their subject-matter peers—lifting as we climb.
This message of mentorship and supporting scientific advancement through peer review is especially critical given the August 25 US Office of Science and Technology Policy (OSTP) memorandum calling for “free, immediate, and equitable access to federally funded research.” The memo created quite a stir among research community members receiving US federal government funding. However, here at ECS, we are ready for the road ahead and any turns it may reveal. All ECS journals are either Gold or Green Open Access; our member discounts make our open access article processing fees among the most competitive in our subject area; our publishing partner IOP is growing the reach of open access publishing by expanding the number of transformative agreements worldwide; and ECS authors can promote their work and related data on their personal website(s), institutional website(s), repositories, scientific social networks, and third-party websites. While many publishers are waiting for additional guidance from OSTP, ECS is ready and in position to provide the international scientific community with a forum to publish their work without fear of noncompliance.
While some look toward 2023 with speculation and perhaps fear of the unknown, ECS had the foresight in 2014 to launch Free the Science. Knowing that the future of science would be open, ECS adopted strategies to become a true community publisher for electrochemical and solid state science and technology research. We are ready for the road ahead!
The ECS Board of Directors met on Thursday, October 13, in conjunction with the 242nd ECS Meeting in Atlanta, October 9–13. This meeting was only the Board’s second in-person gathering since October 2019, and there was no shortage of important topics to discuss.
First and foremost was approving the 2023 budget.
Unlike budgets in recent years, which focused more on ensuring that ECS’s core programs and services were at least break-even, if not returning surplus to support the Society’s mission, this budget seeks to invest heavily in ECS’s future. The ECS Board approved a budget that contains nearly $1M in such investments, including launching a new education initiative designed to provide critical battery manufacturing workforce development resources; reaching out to new and potential members around the globe; hosting our first ECS meeting in Europe in well over a decade; and covering all 2023 article processing charges (APCs) in our two new OA journals, ECS Sensors Plus and ECS Advances. True, we are operating in a time of
rising interest rates and economic uncertainty, but given the strength of ECS’s operations and our financial reserves, we are uniquely positioned for growth in the coming years—if we make the proper investments now. This budget is the start of that growth!
In addition to the budget, the Board held in-depth discussions on divisions’ field of interest statements, especially with respect to nanotechnology; ways to redefine membership to include more of the broader ECS community as members; and the potential for establishing a simpler, more transparent reporting mechanism for division finances.
There was lively discussion over the course of the four-hour meeting, which concluded by thanking the following members whose terms had ended: Shirley Meng, Battery Division Chair; Jamie Noël, Corrosion Division Chair; and Jessica Koehne, Sensor Division Chair. Our sincerest thanks to Shirley, Jamie, and Jessica for their years of service and dedication to the ECS Board and the Society!
And of course, we welcomed new members who joined us for their first ECS Board meeting: Uroš Cvelbar, DS&T Division Chair; Qiliang Li, EPD Division Chair; Maria Inman, IE&EE Division Chair; Jeff Blackburn, NANO Division Chair; and Jennifer Hite, Chair of the Interdisciplinary Science and Technology Subcommittee. Welcome to the ECS Board, and here’s wishing you all the best as you begin your terms. The Society is grateful and honored that you are serving in this most important of capacities.
The Electrochemical Society Publications Subcommittee, Technical Affairs Committee, and Board of Directors congratulate the newly appointed and reappointed members of our Editorial Board. It is through the unwavering commitment of our Editorial Board Members that The Electrochemical Society family of journals continues to realize great success. Thank you for your service to ECS!
Nae-Lih (Nick) Wu
Associate Editor for Batteries and Energy Storage
January 1, 2014–December 31, 2025
Thierry Brousse
Associate Editor for Batteries and Energy Storage
June 1, 2012–December 31, 2025
Janine Mauzeroll
Technical Editor for Organic and Bioelectrochemistry
December 1, 2016–December 31, 2025
Xiao-Dong Zhou
Technical Editor for Fuel Cells, Electrolyzers, and Energy Conversion
January 1, 2020–December 31, 2026 JES & JSS
Kailash Mishra
Technical Editor for Luminescence and Display Materials, Devices, and Processing
October 1, 2011–September 30, 2023
Dirk Guldi
Technical Editor for Carbon Nanostructures and Devices
July 15, 2022–July 14, 2025
Won Bin Im
Associate Editor for Luminescence and Display Materials, Devices, and Processing
July 11, 2022–July 14, 2023
Stefano Cinti Technical Editor for Sensors
January 1, 2023–December 31, 2025
Netz Arroyo Technical Editor for Sensors
January 1, 2023–December 31, 2025
The Spring 2023 issue of Interface will be a Special Topics issue on Neuromorphic Computing.
Spring 2023 will also include the Highly Cited Researchers, columns from our new contributing editors, and Looking at Patent Law, as well as the latest news about people, students, and the Society.
Chair Maria Inman and Secretary/Treasurer Elizabeth Biddinger presented the 2022 IE&EE H. H. Dow Memorial Student Achievement Award to Arghya Patra at the 242nd ECS Meeting. Patra is a PhD candidate and Mavis Future Faculty Fellow in the University of Illinois Urbana-Champaign’s Department of Materials Science and Engineering. Dow Chemical Company funds the award to encourage promising young engineers and scientists to continue careers in electrochemical engineering and applied electrochemistry.
The award presentation took place before Patra gave his award talk, “Electrochemically Grown Highly Textured Thick Ceramic Oxide Films for Energy Storage: A New Manufacturing Paradigm for Cathode Materials.” In it, he described his work in Prof. Paul V. Braun’s research group on direct electrodeposition of thick ternary ceramic oxide films as an alternate scalable manufacturing technique for fabrication of binder-and-additive free cathode materials for secondary batteries.
Brett Lucht, Chair University of Rhode Island
Jie Xiao, Vice Chair
Jagjit Nanda, Secretary Xiaolin Li, Treasurer Doron Aurbach, Journals Editorial Board Representative
Dev Chidambaram, Chair University of Nevada Reno
Eiji Tada, Vice Chair
Rebecca Schaller, Secretary/Treasurer Sannakaisa Virtanen, Journals Editorial Board Representative
Uroš Cvelbar, Chair
Jožef Stefan Institute
Sreeran Vaddiraju, Vice Chair
Zhi David Chen, Secretary Thorsten Lill, Treasurer Peter Mascher, Journals Editorial Board Representative
Natasa Vasiljevic, Chair University of Bristol
Luca Magagnin, Vice Chair
Andreas Bund, Secretary Antoine Allanore, Treasurer Takayuki Homma, Journals Editorial Board Representative
Electronics and Photonics
Qiliang Li, Acting Chair/Vice Chair George Mason University
Vidhya Chakrapani, 2nd Vice Chair
Zia Karim, Secretary
Erica Douglas, Treasurer
Fan Ren, Journals Editorial Board Representative Jennifer Bardwell, Journals Editorial Board Representative
William Mustain, Chair University of South Carolina
Katherine Ayers, Vice Chair Minhua Shao, Secretary
Hui Xu, Treasurer
Xiao-Dong Zhou, Journals Editorial Board Representative
High-Temperature Energy, Materials, and Processes
Sean R. Bishop, Chair Sandia National Laboratories
Cortney Kreller, Sr. Vice Chair
Xingbo Liu, Jr. Vice Chair Teruhisa Horita, Secretary/Treasurer Xiao-Dong Zhou, Journals Editorial Board Representative
Industrial Electrochemistry and Electrochemical Engineering
Maria Inman, Chair Faraday Technology, Inc.
Paul Kenis, Vice Chair
Elizabeth Biddinger, Secretary/Treasurer John Harb, Journals Editorial Board Representative
Luminescence and Display Materials
Rong-Jun Xie, Chair
Xiamen University
Eugeniusz Zych, Vice Chair
Dirk Poelman, Secretary/Treasurer Kailash Mishra, Journals Editorial Board Representative
Nanocarbons
Jeff L. Blackburn, Chair National Renewable Energy Laboratory
Ardemis Boghossian, Vice Chair
Yan Li, Secretary
Hiroshi Imahori, Treasurer Francis D’Souza, Journals Editorial Board Representative
Organic and Biological Electrochemistry
Sadagopan Krishnan, Chair
Oklahoma State University
Song Lin, Vice Chair
Jeffrey Halpern, Secretary/Treasurer Janine Mauzeroll, Journals Editorial Board Representative
Physical and Analytical Electrochemistry
Andrew Hillier, Chair
Iowa State University
Stephen Paddison, Vice Chair
Anne Co, Secretary
Svitlana Pylypenko, Treasurer David Cliffel, Journals Editorial Board Representative
Larry Nagahara, Chair
Johns Hopkins University
Praveen Kumar Sekhar, Vice Chair
Dong-Joo Kim, Secretary
Leyla Soleymani, Treasurer
Ajit Khosla, Journals Editorial Board Representative
Sheikh Ali Akbar, Ohio State University
Shekhar Bhansali, Florida International University
Brett Lucht, University of Rhode Island
Vice Chair
Jie Xiao, Pacific Northwest National Laboratory Secretary
Jagjit Nanda, Stanford Linear Accelerator Center Treasurer
Xiaolin Li, Pacific Northwest National Laboratory Members at Large
Veronica Augustyn, North Carolina State University
Tom Barrera, LIB-X Consulting
Dominic Bresser, Karlsruher Institut für Technologie
Jason Croy, Argonne National Laboratory
Neil Das Gupta, University of Michigan
Robert Dominko, National Institute of Chemistry
Josh Gallaway, Northeastern University
Bing Joe Hwang, National Taiwan University of Science & Technology
Nobuyuki Imanishi, Mie University
Xiaolin Li, Pacific Northwest National Laboratory
Bin Li, Idaho National Laboratory
Boryann Liaw, Idaho National Laboratory
Jun Lu, Argonne National Laboratory
Yi-Chun Lu, The Chinese University of Hong Kong
John Muldoon, Toyota Research Institute of North America
Jennifer Rupp, Massachusetts Institute of Technology
Dan Steingart, Columbia University
Venkataram Thangadurai, University of Calgary
Roseanne Warren, University of Utah
Kang Xu, United States Army Research Laboratory
Guihua Yu, University of Texas at Austin
Marina Yakovleva, Livent Corporation
Won-Sub Yoon, Sungkyunkwan University
Dev Chidambaram, University of Nevada, Reno
Vice Chair
Eiji Tada, Tokyo Institute of Technology Division Secretary/Treasurer
Rebecca Schaller, Sandia National Laboratories
Members at Large
Yaiza Gonzalez-Garcia, Technische Universiteit Delft
Rajeev Gupta, North Carolina State University
Michael Rohwerder, Max-Planck-Institut für Eisenforschung GmbH
Eric Schindelholz, Ohio State University
Brendy Rincon Troconis, University of Texas at San Antonio
Hiroaki Tsuchiya, Osaka University
Larry Nagahara, Johns Hopkins University
Vice Chair
Praveen Kumar Sekhar, Washington State University Secretary
Dong-Joo Kim, Auburn University Treasurer
Leyla Soleymani, McMaster University
Michael T. Carter, KWJ Engineering, Inc.
Pengyu Chen, Auburn University
Bryan Chin, Auburn University
Seyedehaida (Aida) Ebrahimi, Pennsylvania State University
Jay Grate, Pacific Northwest National Laboratory
Peter Hesketh, Georgia Institute of Technology
A. Robert Hillman, University of Leicester
Gary Hunter, NASA Glenn Research Center
Takeo Hyodo, Nagasaki University
Sangmin Jeon, Pohang University of Science and Technology
Mira Josowicz, Georgia Institute of Technology
Jing Li, NASA Ames Research Center
Chung-Chiun Liu, Case Western Reserve University
Vadim Lvovich, NASA Glenn Research Center
Sushanta Mitra, University of Waterloo
Rangachary Mukundan, Los Alamos National Laboratory
Milad Navaei, Georgia Institute of Technology
Ramaraja Ramasamy, University of Georgia
Antonio Ricco, NASA Ames Research Center
Michael Sailor, University of California San Diego
Yasuhiro Shimizu, Nagasaki University
Aleksandr Simonian, National Science Foundation
Joseph Stetter, KWJ Engineering, Inc.
Thomas Thundat, The University at Buffalo
Raluca van Staden, National Institute of Research for Electrochemistry
Petr Vanysek, Northern Illinois University
Nianqiang Nick Wu, University of Massachusetts Amherst Tomoyuki Yasukawa, University of Hyogo
Turgut Gür, Chair
President, Spring 2023
Gerardine Botte Senior Vice President, Spring 2024
Colm O’Dwyer Second Vice President, Spring 2025
James Fenton Third Vice President, Spring 2026
Marca Doeff Secretary, Spring 2024
Elizabeth Podlaha-Murphy Treasurer, Spring 2026
Christopher Jannuzzi Executive Director, Term as ED Audit
Eric Wachsman, Chair
Immediate Past President, Spring 2023
Turgut Gür President, Spring 2023
Gerardine Botte
Senior Vice President, Spring 2024
Elizabeth Podlaha-Murphy Treasurer, Spring 2026
Robert Micek Nonprofit Financial Professional, Spring 2025
Alice Suroviec, Chair Spring 2025
Svitlana Pylypenko Spring 2024
Paul Gannon Spring 2024
Stephen Maldonado Spring 2025
David Hall Spring 2025
Wen She Spring 2026
Samantha Gateman Spring 2026
Walter Van Schalkwijk Spring 2023
Tobias Glossman Spring 2023
Mohammad Sabeti Spring 2024
Liam Witteman Spring 2023
Marca Doeff Secretary, Spring 2024
Neal Golovin
Eric Wachsman, Chair
Chair, Individual Membership Committee, Spring 2023
Immediate Past President, Spring 2023
Johna Leddy Past Officer, Spring 2023
Esther Takeuchi Past Officer, Spring 2024
Marca Doeff Secretary, Spring 2024
Elizabeth Podlaha-Murphy Treasurer, Spring 2026
Elizabeth Podlaha-Murphy, Chair
Treasurer, Spring 2026
E. J. Taylor Spring 2023
Bruce Weisman Spring 2023
Dong-Joo Kim Spring 2025
Robert Micek Spring 2025
Marca Doeff Secretary, Spring 2024
Tim Gamberzky Chief Operating Officer, Term as COO
Shelley Minteer, Chair Spring 2023
Vimal Chaitanya Spring 2024
Mikhail Brik Spring 2024
Alanah Fitch Spring 2025
Shigeo Maruyama Spring 2025
Jean St-Pierre Spring 2025
Andrew Hoff Spring 2026
Dev Chidambaram Spring 2026
Shirley Meng Spring 2026
Nianqiang Wu Spring 2023
John Flake Spring 2023
Fernando Garzon Spring 2023
Turgut Gür President, Spring 2023
Neal Golovin, Chair Spring 2023
Alice Suroviec Spring 2023
Uroš Cvelbar Spring 2023
John Staser Spring 2024
Shirley Meng Spring 2024
Shuthi T. Kumar Raj Spring 2025
Qizhi Liu Spring 2025
Jiaxin Duan Spring 2024
Ashwin Ramanujam Spring 2023
Alex Peroff
Chair, Institutional Engagement Committee, Spring 2025
Marca Doeff Secretary, Spring 2024
Alex Peroff, Chair Spring 2025
Hemanth Jaganathan Spring 2023
Thomas Barrera Spring 2023
David Carey Spring 2023
Yuyan Shao Spring 2024
Christopher Beasley Spring 2024
Karen Poe Spring 2024
Yoko Yamakoshi Spring 2025
Santosh Vijapur Spring 2025
Yaw Obeng Spring 2025
Neal Golovin
Eric Wachsman, Chair
Immediate Past President, Spring 2023
Vimal Chaitanya Spring 2023
Jie Xiao Spring 2023
Andy Herring Spring 2023
James Fenton
Christopher Jannuzzi
Gerardine Botte, Chair
Third Vice President, Spring 2023
Executive Director, Term as ED
Senior Vice President, Spring 2023
Turgut Gür President, Spring 2023
Eric Wachsman
Stefan De Gendt
James Fenton
Colm O’Dwyer
Jennifer Hite
Christopher Jannuzzi
Immediate Past President, Spring 2023
Second Immediate Past President, Spring 2023
Chair, Meetings Subcommittee, Spring 2023
Chair, Publications Subcommittee, Spring 2023
Chair, ISTS Subcommittee, Spring 2025
Executive Director, Term as ED
Colm O’Dwyer, Chair
James Fenton, Vice Chair
Krishnan Rajeshwar
Robert Savinell
Ajit Khosla
Second Vice President, Spring 2023
Third Vice President, Spring 2023
JSS Editor, 31 December 2024
JES Editor, Spring 2024
ECS Sensors Plus Editor, Fall 2024
Robert Kelly Interface Editor, Spring 2025
Pawel Kulesza Spring 2024
Ahmet Kusoglu Spring 2024
Venkataraman Thangadurai Spring 2023
James Fenton, Chair
Colm O’Dwyer, Vice Chair
Third Vice President, Spring 2023
Second Vice President, Spring 2023
Jianlin Li Spring 2023
Francis D’Souza Spring 2024
Peter Mascher Spring 2025
Jennifer Hite, Chair Spring 2025
Alice Suroviec Spring 2023
Uroš Cvelbar Spring 2023
Chockkalingam Karuppaiah Spring 2023
Scott Calabrese Barton Spring 2023
Alok Srivastava Spring 2024
Rangachary Mukundan Spring 2024
James Fenton Spring 2024
Christopher Johnson Spring 2025
James Noël Spring 2025
Greg Jackson Spring 2025
Jeff L. Blackburn Spring 2025
Luca Magagnin Spring 2025
James Fenton, Chair
Brett Lucht
Third Vice President, Spring 2023
Chair, Battery Division, Fall 2024
Dev Chidambaram Chair, Corrosion Division, Fall 2024
Larry Nagahara
Qiliang Li
William Mustain
Chair, Sensor Division, Fall 2024
Acting Chair, Electronics and Photonics Division, Spring 2023
Chair, Energy Technology Division, Spring 2023
Sadagopan Krishnan Chair, Organic and Biological Electrochemistry Division, Spring 2023
Andrew Hillier Chair, Physical and Analytical Electrochemistry Division, Spring 2023
Natasa Vasiljevice
Chair, Electrodeposition Division, Fall 2023
Sean Bishop Chair, High Temperature Materials Division, Fall 2023
Rong-Jun Xie Chair, Luminescence and Display Materials Division, Fall 2023
Uroš Cvelba
Chair, Dielectric Science and Technology Division, Spring 2024
Jeff Blackburn Chair, Nanocarbons Division, Spring 2024
Maria Inman Chair, Industrial Electrochemistry and Electrochemical Engineering Division, Spring 2024
Jennifer Hite Chair, Interdisciplinary Science and Technology Subcommittee, Spring 2025
Society Historian
Roque Calvo Spring 2023
American Association for the Advancement of Science
Christopher Jannuzzi
Science History Institute
Ekaterina Pomerantseva
National Inventors Hall of Fame
Shelley Minteer
Chair, Individual Membership Committee, Spring 2023
Elizabeth Podlaha-Murphy Treasurer, Spring 2026
Term as Executive Director
Heritage Councilor, Spring 2023
Chair, Honors & Awards Committee, Spring 2023
The 5th Organic Battery Days (OBD) Workshop held at the University of Houston
on October 13 to 14, 2022 continued a series of events that began in Uppsala, Sweden, in 2017. Prof. Yan Yao, Hugh Roy and Lillie Cranz Cullen Distinguished Professor, Department of Electrical and Computer Engineering and the Texas Center for Superconductivity at the University of Houston (TcSUH), chaired the workshop. ECS was a sponsor of the event, which took place in the United States for the first time. Texas A&M University and Pacific Northwest National Laboratory served as organizing partners.
The 2022 OBD aimed to strengthen communication and cooperation among battery experts from academic organizations, research institutes, and manufacturing companies. Eighty attendees exchanged knowledge and experience on the most recent developments in organic batteries, discussing the design and synthesis of organic electroactive materials, reaction mechanisms, the study of these materials in various electrochemical systems (e.g., multivalent ion batteries, flow cells), and emerging applications.
Distinguished researchers Kenichi Oyaizu of Waseda University, Héctor D. Abruña of Cornell University, and Michael J. Aziz of Harvard University presented plenary talks. Prof. Oyaizu’s presentation centered on polymers for high-power-density organic batteries. Prof. Aziz spoke on recent progress in aqueous organic flow batteries. Prof. Abruña joined virtually to discuss novel architectures and experimental strategies for organic electrical energy storage. In addition, researchers from 11 countries presented 17 invited and 10 contributed talks, and 11 posters.
While most of the meeting was in person, a special Virtual Poster Session provided an opportunity for those who could not physically attend to participate. In keeping with OBD tradition, three Best Poster Prizes were awarded to outstanding presentations. Recipients were Erin S. Picton of the University of Houston, Rambabu Darsi of Université Catholique de Louvain, and Sam Kopfinger of the University of Chicago. Abstracts are available on the workshop website
The 5th OBD placed particular attention on involving students and young professionals, who, in turn, generously volunteered their time and talent to help plan and implement the workshop. The ECS University of Houston Student Chapter generously hosted a Welcome to Houston BBQ on October 12 and served as hosts to participants throughout the workshop.
The workshop organizers are grateful for crucial financial support from Vigor, Neware Corp., Sugino Corp., Pacific Northwest National Laboratory, and the Cullen College of Engineering and the Texas Center for Superconductivity at the University of Houston
ECS is delighted to welcome Advanced Cell Engineering as its newest Institutional Member at the Sustaining Level. Advanced Cell Engineering (ACE) develops and licenses advanced battery technologies that have a meaningful impact on electric vehicle performance. ACE is developing a continuous stream of
innovations, including an Advanced LFP chemistry with significantly higher energy density, unsurpassed safety, and long cycle life. ACE chemistry can be used in any cell format and produced on standard manufacturing equipment. For more information about Advanced Cell Engineering or to explore career opportunities, please visit https://www. advancedcellengineering.com/.
This year’s Gordon Research Seminar on Electrochemistry took place from September 10 to 11 in Ventura, CA, and was chaired by Dr. Elizabeth Corson (Stanford University Postdoctoral Fellow) and cochaired by Jeremy Dawkins (PhD candidate at McGill University). The seminar, titled Fundamental and Applied Electrochemistry in Analytical, Nanoscale, and Energy, spanned a wide breadth of topics in the field. Students had the opportunity to give oral and poster presentations of their work. The seminar included 14 oral presentations and 68 poster presentations by students and postdocs, including cutting-edge results on porous coaxial twin-electrodes, samarium redox catalysts for reductive coupling reactions, highthroughput aqueous redox flow batteries, and much more.
A mentorship panel discussed “Excellence in Scientific Communication.” Four invited principal investigators hosted the panel: Dr. Debra Rolison (U.S. Naval Research Laboratory) moderator, Profs. William Tarpeh (Stanford University), Jeffrey Dick (Purdue University), and Hannah Shafaat (Ohio State University). The panelists’ presentations on their work and their philosophies on scientific communication spanned scientific presentations, mentoring students, and writing manuscripts for publication.
A main goal of the conference was to bring together diverse perspectives from students, postdocs, speakers, panelists, and discussion leaders. We sought to include people of all races, ethnicities, national origins, gender identities, gender expressions, sexual orientations, ages, disabilities, socio-economic statuses, classes, and religions. The attendees reflected these values with 46% identifying as women, 58% identifying as people of color, and 12% participating from international institutions.
In a vote at the end of the seminar, Alessandra Zito (University of California, Irvine) was elected chairperson of the next conference with Joy Zheng (Massachusetts Institute of Technology) acting as co-chair. We wish them great success with their new, well-deserved positions and the 2024 seminar!
We are extremely grateful to all the participants, presenters, and contributors for supporting events like these which are essential to our scientific community. We hope that they continue their support for young, creative, and diverse students who require these seminars to learn, teach, and ultimately to flourish. The Electrochemistry Society was a proud sponsor of the 2022 Gordon Research Seminar.
The
Each year, ECS awards up to four summer fellowships to support graduate students continuing their research from June through August in a field of interest to the Society. The ECS Summer Fellowships program comprises four named awards:
• Vimal H. Chaitanya, Chair, New Mexico State University
•
•
EdwardG. Weston
Fellowship Joseph W. Richards Fellowship F.M. Becket Fellowship H. H. Uhlig FellowshipThe Society also awards the Colin Garfield Fink Summer Fellowship to one postdoctoral scientist or engineer who is a member in good standing. The Summer Fellowship and Colin Garfield Fink Fellowship recipients are each awarded US $5,000.
Congratulations to the five 2022 recipients!
•
•
•
Electrochemical advanced oxidation processes present an appealing option to treat reverse osmosis concentrate (ROC) from industrial and municipal wastewater facilities, particularly considering higher ionic conductivity than influent wastewater due to RO retention of ionic species.1-6 Compared to conventional advanced oxidation processes (AOPs) that require precursor chemicals and often complex engineering (e.g., O3/H2O2/UV, Fenton process),7 an electrochemical process benefits from the chemical-free operation, small footprint, and modular design that fit with an distributed treatment scheme at varying flow capacities, as well as the opportunity to exploit renewable energy sources.8 An electrochemical process can degrade pollutants or valorize constituents in ROC via direct electron transfer at the electrode surface (i.e., anode or cathode) 9-13 or generate in-situ strong oxidizing radicals such as •OH and SO4•− , 14-18 (Fig. 1A). An anodic oxidation scheme can avoid the challenges of relying on radicals, including mass transfer limitation due to the short life of radicals and performance loss due to radical scavenging.
The relatively high cost of electrodes, especially for anodic oxidation (e.g., borondoped diamond), the generation of toxic byproducts in the effluent (e.g., disinfection byproducts), and electrodes’ fouling and scaling in complex wastewater matrices have impeded the widespread implementation of electrochemical treatment. Complex wastewater matrices from various sources,
including industrial and municipal sectors, contain multiple anions and cations that could be problematic to the performance of electrochemical treatment. While there has been a great focus on halides, which can pose the risk of compromising effluent quality, other constituents can affect cell performance by directly interacting with the electrode surface in a manner that inhibits their desired reactions. Cations with various reduction potentials can form scale on the cathode surface and deactivate the desired cathodic reduction (Fig. 1B). One notable advance in electrode material design is an effort to downsize the catalysts from traditional nanoscale to sub-nanoscale or
even to the single-atom limit. It is noteworthy that downsizing metal catalysts (e.g., Pt, Co, Pd) from nanoscale clusters to single-atom catalysts (SACs) could not only improve the selectivity toward redox reactions involving target pollutants but also the longevity of catalysts in complex water matrices. This strategy has also been previously employed to improve selectivity and mitigate parasitic reactions and poisoning effects in other electrochemical applications. This feature of SACs originates from their limited adsorption sites that enable nearly 100% atomic efficiency and hinder side reactions that are commonly observed in nanoparticle counterparts. (A)
Fig. 1. (A) Reaction pathways for in-situ generated radicals ( OH, SO4 , Cl ) via electrochemical redox processes. (B) Parasitic/competing for anodic and cathodic reactions in complex water matrices containing various cations and anions that may result in the formation of toxic byproducts and/or deactivation and poisoning of the catalyst.
Fig. 2. (A) Cyclic voltammograms of Co1-NCB-SS in various electrolytes with and without NOM at the scan rate of 50 mV/s. The ionic conductivity for all electrolytes is 2.1 mS/cm. The stronger oxidation peak at around 1.9 V vs. RHE in the presence of NOM confirms the interaction of NOM molecules with the electrode surface. (B) The cyclic voltammograms of Co1-NCB-SS with three different NOM concentrations. The stronger redox peak with higher NOM concentration proves the redox interaction of NOM molecules with the Co1-NCB-SS electrode.
We present an electrochemical oxidation process employing Co SAC (Co1) anchored onto N-doped carbon black (Co1-NCB) as an electrocatalyst. We construct an electrode using Co1-NCB and a single-cell electrochemical reactor that treats synthetic ROC, mimicking the characteristics of real ROC generated after the tertiary treatment of municipal and industrial wastewater. We target natural organic matter (NOM) to simulate a scenario wherein discharge total organic carbon (TOC) is regulated. This project will address the critical bottleneck in electrochemical processes (i.e., decreasing cost and improving efficiency) by developing cost-effective and selective electrode materials that can treat or valorize ROC for safe discharge or reuse. The overarching goal is to develop an electrochemical prototype to degrade a wide range of organic contaminants, minimize electrodes’ fouling and scaling, and recover valuable resources (e.g., metals and salts), contributing to achieving “pipe parity” and “circular economy” using small-scale and modular electrochemical water treatment systems.
We selected stainless steel as an initial substrate to prepare the Co1-NCB-loaded electrode (Co1-NCB-SS) for its costeffectiveness and relatively high anodic stability against corrosion. We observed a higher current density within the positive scan range (1.0 to 2.7 V vs. RHE) during cyclic voltammetry (CV) when synthetic ROC was used compared to control electrolytes that do not contain NOM (i.e., Na2SO4, Na2SO4 + NaCl, and synthetic ROC without NOM) (Fig. 2A). Note that the salt concentrations were adjusted for all electrolytes to obtain a similar ionic conductivity (2.1 ± 0.2 mS/ cm). We further observed a pronounced
peak at around 1.9 V vs RHE along the oxidative pathway in the presence of NOM. Considering that NOM in the electrolyte led to higher current density (i.e., activity) and the appearance of a distinctive oxidation peak, we postulate the direct electrochemical interaction of NOM with the Co1-NCB-SS electrode surface. We further observed that the intensity of the oxidation peak at 1.9 V vs. RHE substantially increased when NOM concentration was increased from 60 to 1000 mg C L-1 (Fig. 2B). These results collectively suggest direct electron transfer processes between NOM molecules and the Co1-NCB-SS electrode and highlight Co1’s catalytic capability. In Fig. 2A, we also observed a much smaller peak at around the same NOM oxidation potential (~2.0 V), even in the absence of NOM. This peak might be attributed to the adsorbed –OH or –O on the catalyst surface, which is common at potentials greater than 1.7 V vs. RHE, assuming >0.5 V beyond water oxidation thermodynamic potential (2H2O → O2 + 4 H+ + 4 e-; Eo = 1.23 V vs RHE, involving OH* and O* as adsorbed intermediate species). Among electrolytes without NOM, the activity was the highest for the case when the electrolyte contained Cl-. We attribute this to Cl- oxidation reaction (Cl- + H2O → HOCl + H+ + 2e-; Eo = 1.7 V vs. RHE). We accordingly observed that the activity was the lowest for the case of synthetic ROC without NOM. This electrolyte contained cations (e.g., Ca2+, Mg2+), and therefore a lower concentration of Cl- was added to reach the target conductivity value of 2.1 mS/cm. These cations also do not interact with the positively charged electrode (i.e., anode). Oxidation of SO42- is unlikely since the standard redox potential for direct SO42oxidation at the anode (SO42- → SO4 •− + e-;
Eo = 3.1 V vs. RHE) is more positive than the potential range CV was conducted.
Future work will focus on developing and demonstrating a modular electrochemical reactor capable of treating ROC from different facilities with large variations in the concentration of ions, organics, salts, and recalcitrant pollutants. Our system will also be able to adapt to the broad geographic and temporal variations in ROC constituents.
The author gratefully acknowledges The Electrochemical Society’s support through the Colin Garfield Fink Summer Fellowship. The author also thanks his postdoc mentor, Prof. Jaehong Kim, for his guidance and support. The author would also like to acknowledge the members of the Kim group in the Department of Chemical and Environmental Engineering at Yale University for their input and feedback on this project.
The Electrochemical Society. DOI: 10.1149/2.F03224IF
Mohammadreza (Reza) Nazemi is currently an assistant professor in the Department of Mechanical Engineering at Colorado State University (CSU). His group’s research at CSU focuses on challenges associated with renewable energy utilization, storage, and transport.
His group aims to contribute to the future electrification, decarbonization, and sustainability of various modern industries, including transportation, chemical, and wastewater treatment, with positive social and economic outcomes.
1. P. Westerhoff, H. Moon, D. Minakata, and J. Crittenden, Water Research, 43, 3992 (2019).
2. R. C. Scholes, A. N. Stiegler, C. M. Anderson, and D. L. Sedlak, ACS Environmental Au, 1, 7 (2021)
3. T. Zhou, T.-T. Lim, S.-S. Chin, and A. G. Fane, Chem Eng J, 166, 932 (2011).
4. E. Dialynas, D. Mantzavinos, and E. Diamadopoulos, Water Research, 42, 4603 (2008).
5. G. Pérez, A. R. Fernández-Alba, A. M. Urtiaga, and I. Ortiz, Water Research, 44, 2763 (2010).
6. G. Hurwitz, E. M. V. Hoek, K. Liu, L. Fan, and F. A. Roddick, Chem Eng J, 249, 180 (2014).
7. Q. Xiang, Y. Nomura, S. Fukahori, T. Mizuno, H. Tanaka, and T. Fujiwara, Curr Pollut Rep, 5, 294 (2019).
8. B. P. Chaplin, Environmen Sci: Process Impacts, 16, 1182 (2014).
9. J. Xie, C. Zhang, and T. D. Waite, Water Research, 216, 118319 (2022).
10. J. R. Jasmann, P. B. Gedalanga, T. Borch, S. Mahendra, and J. Blotevogel, Environ Sci Technol, 51, 12619 (2017).
11. A. Fenti, Y. Jin, A. J. H. Rhoades, G. P. Dooley, P. Iovino, S. Salvestrini, D. Musmarra, S. Mahendra, G. F. Peaslee, and J. Blotevogel, Chem Eng J Adv, 9, 100205 (2022).
12. R. B. Young, N. E. Pica, H. Sharifan, H. Chen, H. K. Roth, G. T. Blakney, T. Borch, C. P. Higgins, J. J. Kornuc, A. M. McKenna, and J. Blotevogel, Environ Sci Technol, 56, 2455 (2022).
13. S. Garcia-Segura, X. Qu, P. J. J. Alvarez, et al., Environ Sci: Nano, 7, 2178 (2020).
14. C. Weng, Y.-H. Chuang, B. Davey, and W. A. Mitch, Environ Sci Technol, 54, 12593 (2020).
15. M. Weng, and J. Pei, Desalination, 399, 21 (2016).
16. Y. Li, C. J. Miller, L. Wu, and T. D. Waite, Environ Sci Technol, 56, 5820 (2022).
17. S.-D. Wang, L.-X. He, L. Zhou, S.-D. Xian, and J.-H. Liu, Sep Purif Technol, 291, 120964 (2022).
18. Y. Liu, X. Fan, X. Quan, Y. Fan, S. Chen, and X. Zhao, Environ Sci Technol, 53, 5195 (2019).
Extreme fast charging (XFC) of lithium-ion batteries (LIBs) in 10 minutes is one of the main goals of the US Advanced Battery Consortium for low-cost, fast-charged electric vehicles by 2023.1 However, existing LIBs cannot achieve these XFC goals without significant capacity fade over cycling due to complex XFC degradation modes. One of the key XFC failure mechanisms is dead Li plating on the graphite anode.2 While numerous methods have detected Li plating, they lack three-dimensional non-invasive visualization of dead Li on graphite anodes in full cells during battery cycling.3
Herein, we demonstrate the viability of high-resolution (spatial resolution: 10–15 µm) neutron micro-computed tomography (µCT) for in-situ characterization of dead Li on graphite anodes (thickness: ~130 µm) in full cells containing NMC cathode, that were cycled at 1C and 6C. We used neutrons because they are particularly sensitive to detecting Li on graphite due to the large difference in their total neutron cross-sections (Li = 72 barns; C = 6 barns4).
To leverage high spatial resolution neutron µCT, we designed a neutron-friendly battery. Our cell design constituted a 2032 format coin cell with several custom modifications, namely using an anhydrous deuterated electrolyte, fluorinated gasket, Al spacer
Fig. 1. (A) Design of neutron-friendly lithium-ion battery for fast charging. (B) Electrochemical characterization of custom battery showing fast-charging performance at 6C followed by a slow discharge at C/2.
(cathode), Cu coated Al spacer (anode), custom Al spring, PVDF separator, and 3–4 mm diameter battery electrodes5 (Fig. 1A). Our modified battery demonstrated excellent neutron transmission at the separatorelectrolyte-anode interface as well as typical XFC cycling at 6C between 3.0 V and 4.1 V (Fig. 1B).
We performed neutron µCT at the ICON beamline6 at The Swiss Spallation Neutron Source at the Paul Scherrer Institut in Switzerland. We imaged two NFBs in the
discharged state cycled at 1C for four XFC cycles and at 6C for six XFC cycles (Fig. 2). Our imaging results show that dead Li plates in a circular fashion on both the graphite anodes (XY images in Figure 2(A-B)). Here, the XY images correspond to top-view 2D slices of a 3D reconstructed volume. We notice dead Li only on one side of the anode cycled at 1C. Whereas, µm-scale dead Li deposits can be seen all around the graphite anode cycled at 6C. Moreover, we observe a mixture of isolated and continuous dead
Li deposits at 6C. These findings suggest that at faster charging rates, the morphology of dead Li is mossy-like. Additionally, the amount of dead Li deposits qualitatively seems to increase at 6C as compared to 1C. Note that we observed dead Li at 1C because of the high-capacity graphite and NMC electrodes (based on reversible C/10 capacity of ~ 330 mAh/g for 0.005 to 1.5V vs Li) used. The next steps in analysis are quantifying the amount of dead Li in 3D and correlating it to capacity fade to propose a mechanism of morphological evolution of dead Li at 6C.
Finally, our cell design and imaging results pave the way for in-situ and operando neutron imaging studies of battery chemistries including Li-metal batteries that plate and strip plated Li, solid-state Li-S batteries, and Li-Si cells.
The author would like to thank The Electrochemical Society for the 2022 Edward G. Weston Summer Research Fellowship. Funding was also provided from the Vehicle Technologies Office of the US Department of Energy’s Office of Energy Efficiency and Renewable Energy under the guidance of the Advanced Battery Cell Research Program (eXtreme fast charge Cell Evaluation of Lithium-ion batteries, XCEL). The Stanford Synchrotron Radiation Lightsource, SLAC National Accelerator Laboratory, is supported by the US Department of Energy, Office of Science, Office of Basic Energy Sciences under Contract No. DE-AC02-76SF00515. The author would like to acknowledge her PhD advisors (Prof. Michael F. Toney, Dr. Johanna Nelson Weker), and her research collaborators at the Paul Scherrer Institut (Prof. Markus Strobl, Dr. Anders Kaestner, Dr. Pavel Trtik), ETH-Zurich (Prof. Vanessa Wood, Mr. Markus Wied), Argonne National Laboratory (Ms. Alison R. Dunlop, Dr. Andrew N. Jansen, Mr. Bryant J. Polzin, Mr. Stephen E. Trask), and the NIST Center for Neutron Research (Dr. Jacob M. LaManna) for making these experiments possible.
The Electrochemical Society. DOI: 10.1149/2.F04224IF
Fig. 2. Top (XY: middle) and cross-sectional (YZ: right; XZ: bottom) view high-resolution 2D neutron images of a 3D volume showing dead Li plated on thick graphite anodes cycled at 1C for 4 cycles (A) and at 6C for 6 cycles (B). Note that both the batteries were imaged in the discharged state. Red dashed circle represents the approximate boundary of the cycled graphite anode. Yellow dashed lines on the XZ and YZ images in (A-B) correspond to the XY slices shown in the middle images.
Maha Yusuf is a finalyear PhD candidate in Chemical Engineering at Stanford University with support from Schlumberger Faculty for the Future Award (2018–2023) and Stanford DARE (Diversifying Academia Recruiting Excellence) Fellowship (2020–2022). In her thesis research, she uses imaging diagnostic tools to understand the failure mechanisms of lithium-ion batteries during extreme fast charging. Maha has won awards that include the 2022 American Chemical Society CAS Future Leader; 2020 Stanford Distinguished Student Energy Lecturer; and 2020 and 2021 ECS and American Institute of Chemical Engineers Travel Grants. She holds an MS in Chemical Engineering from Stanford (2017) and BE from the National University of Sciences and Technology, Pakistan (2013).
https://orcid.org/0000-0001-7908-2915
1. Y. Liu, Y. Zhu, and Y. Cui, Nat Energy, 4(7), 540 (2019).
2. Q. Liu, C. Du, B. Shen, X. Cheng, Y. Ma, G. Yin, and Y. Gao, RSC Adv, 6(91), 88683 (2016).
3. P. P. Paul, E. J. McShane, A. M. Colclasure, et al., Adv Energy Mater, 11(17), 2100372 (2021).
4. https://www.nist.gov/ncnr/neutronscattering-lengths-list
5. M. Yusuf, J. M. LaManna, M. Preefer, P. P. Paul, D. N. Agyeman-Budu, M. F. Toney, and J. N. Weker, ECS Meeting Abstracts, 4, 441 (2021).
6. A. P. Kaestner, S. Hartmann, G. Kühne, G. Frei, C. Grünzweig, L. Josic, F. Schmid, and E. H. Lehmann, Nuclear Instruments and Methods in Physics Research Section A: Accelerators, Spectrometers, Detectors and Associated Equipment, 659(1), 387 (2011).
Hydrogen, which can be readily produced by electrochemical water splitting, has been hailed as one of the most promising green energy sources.1-2 In a water electrolyzer, catalysts are needed for the hydrogen evolution reaction (HER) at the cathode, which are traditionally based on platinum-based materials. Recently, carbon-based nanocomposites have emerged as viable alternatives, which are mostly prepared by pyrolysis or wet chemistry methods, which are time- and energy-consuming.3 Recently, we have exploited magnetic induction heating (MIH) for ultrafast and green preparation of high-performance HER electrocatalysts.4-5
During a typical MIH process (Fig. 1a), the sample is sandwiched between two thin iron sheets in a small assembly and put inside an induction coil. Upon the application of a high-frequency electromagnetic field, eddy currents are generated across the iron sheets. By virtue of Joule’s heating, the surface of the iron sheets will reach a temperature of over 1000 °C within seconds, and thus, thermalradiatively, precursors can be decomposed into carbon-metal nanocomposites. At the end of the heating process, the assembly can be dropped into cold ethanol (~ -78 °C) to quench the reaction. Fig. 1b depicts a temperature diagram from MIH and traditional tube furnace or hydrothermal methods (~ 5 °C min-1), where MIH shows a significantly faster (up to 2400 times) heating rate within the range of 50 to 200 °C s-1. In a recent study, we used the scheme in Fig. 1c to prepare Ru nanoparticles (NPs) supported on carbon paper, which entailed two major steps: deposition of RuCl3 salts onto carbon paper, and MIH heatingquenching at ambient temperature.
For Ru-300 that was prepared by MIH at an eddy current of 300 A for just 6 s, fine NPs were formed on carbon and manifested a size range of 2 to 10 nm, with an average diameter of 6 nm (Fig. 2a and inset). The NPs feature clearly defined lattice fringes with interplanar separations of 0.135 and 0.205 nm (Fig. 2b), which can be ascribed to the hcp Ru (11-20) and (10-11) facets (JCPDS-ICDD #06-0663), respectively.
At a lower current of 200A (Ru-200), amorphous NPs were the main products (Fig. 2c), whereas a higher heating current of 600A yielded chunks of metallic Ru (Ru-600, Fig. 2d). Therefore, MIH can be exploited for the ready control of the size and structure of the Ru NPs by varying the heating current. Note that although
Fig. 1. (a) Photographs of the MIH heating and quenching process. (b) Heating temperature as a function of the heating current and time by MIH, in comparison to tube furnace and hydrothermal methods. (c) Schematic illustration of ultrafast preparation of metal/carbon catalysts using MIH.
the reaction was conducted at ambient temperature, the carbothermal reduction rendered the formation of metallic Ru NPs. The as-prepared carbon-supported Ru NPs exhibited a significant HER activity in 1 M KOH (Fig. 2e); and Ru-300 displayed the best HER activity, with a low overpotential of only -12 mV to reach 10 mA cm-2 (η10), outperforming commercial Pt/C catalysts.
Ru-300 also possessed excellent stability, showing only a 9 mV decay of η10 after 2000 cycles (Fig. 2f).
In summary, a novel MIH method was developed for the ultrafast and green preparation of carbon-supported Ru NPs toward HER in alkaline media, where the size and morphology of the Ru NPs can be
Fig. 2 TEM image of (a, b) Ru-300, (c) Ru-200, and (d) Ru-600. Inset to panel (a) is the size distribution of Ru-300. (e) HER polarization curves of Ru-200, Ru-300, Ru-400, Ru-600, and RuCl3 in 1 M KOH, and (f) stability tests of Ru-300.
readily manipulated by the heating current and duration. It is expected that MIH can be exploited for the design and engineering of high-performance nanocomposite catalysts for important electrochemical energy technologies.
The author gratefully acknowledges the ECS Joseph W. Richards Summer Fellowship for funding support and my advisor Prof. Shaowei Chen for his guidance and support.
The Electrochemical Society. DOI: 10.1149/2.F05224IF
Qiming Liu received his BE in Materials Chemistry in 2018 from Central South University, China. He is currently pursuing a PhD in Chemistry at the University of California, Santa Cruz, under the supervision of Prof. Shaowei Chen. His research focuses on single-atom catalysts toward energy conversion, ligand functionalization of nanoparticles, and ultrafast synthesis of electrocatalysts. Qiming received the 2022 International Precious Metals Institute Student Award, 2021 Sigma XI Grant in Aid of Research, and UCSC Dissertation Year Fellowship.
https://orcid.org/0000-0001-5839-5453
1. B. Z. Lu, Q. M. Liu, and S. W. Chen, ACS Catal, 10(14), 7584 (2020).
2. Z. W. Seh, J. Kibsgaard, C. F. Dickens, I. B. Chorkendorff, J. K. Norskov, and T. F. Jaramillo, Science, 355(6321), eaad4998 (2017).
3. Q. Liu and S. W. Chen, Trends Chem (2022).
4. Q. Liu, B. Lu, F. Nichols, J. Ko, R. Mercado, F. Bridges, and S. Chen, Sus Mat, 2(3), 335 (2022).
5. B. Lu, Q. Liu, C. Wang, Z. Masood, D. J. Morris, F. Nichols, R. Mercado, P. Zhang, Q. Ge, H. L. Xin, and S. Chen, Research 2022, 9756983 (2022).
Li-ion and Li metal batteries are widely used for portable devices due to their high gravimetric and volumetric energy densities and cyclability.1, 2 However, overcharging and extreme discharging of the battery can lead to overheating and thermal runaway, while improper use can lead to fires and explosions.3, 4 The volatility and flammability of the organic solvents (ethylene carbonate/dimethyl carbonate) used as electrolytes are the major sources of these thermal stability issues. Therefore, an alternative to flammable electrolytes, room temperature ionic liquids (RTILs) electrolyte chemistry is investigated due to its high thermal and electrochemical stability. Here, the fundamental understanding of developing high temperature Liion battery materials is evaluated using electrochemistry and depth-dependent X-ray spectroscopy techniques. Fig. 1 shows the electrochemical performance of the LiNi0.5Mn0.3Co0.2O2 (NMC532) cathode cycled against the Li metal anode at high temperature. The voltage profiles exhibited huge polarization and capacity fade during continuous charge discharge cycles (Fig. 1a). This phenomenon is due to poor electrode/electrolyte interface stability between the reactive NMC cathode and the ionic liquid electrolyte.5 After an interface stabilization method based on boron-based additives, Fig. 1b shows the NMC532 cathode exhibiting the reversible
Fig. 1. Galvanostatic charge discharge performance of NMC532 cathode at 100 oC in ionic liquid electrolyte. (a) ionic liquid electrolyte without additives, (b) with additives 2 wt % LiDFOB in 0.8M LiTFSI-Py14TFSI ionic liquid.
performance of charge discharge reactions in pyrrolidinium-based ionic liquid at 100 oC. After stabilizing the CEI, understanding possible redox couple evolution at different depth levels is critical to evaluate the reversible contribution of redox couple in NMC cathode at 100oC. In this investigation, soft and hard X-ray synchrotron-based X-ray absorption spectroscopy (XAS) techniques were used to differentiate the surface and bulk electronic structure evolution of the cathode at high temperature operation. In general, the XAS spectra
provide significant information about the electronic structure and local environmental changes in the absorbing atoms of Li-ion battery electrode material.6 During high valence states, the core electrons will have strong binding toward the nucleus; therefore the photoionization process requires more energy to eject the electrons from the core level.7 Conceivably, when an absorbing atom is at its high oxidation state, the XAS spectra shift to high energy; conversely, a lower
oxidation state will shift the XANES spectra toward low energy. The Ni K edge spectra shift to high energy when the cathode is fully charged, and the edge energy is reversed back to pristine during discharge (Fig. 2a). It clearly indicates that the Ni redox reaction is reversible at high temperature in the bulk of the material. Further, surface sensitive (~ 5 nm) soft X-ray measurements probe the L edge of transition metal where 2p electron is excited to 3d state. Differences in oxidation state, electron spin-state, coordination, and crystal field strength all produce deviations in the L3 and L2-edges. Here, the surface sensitive TEY detection mode was used to understand the Ni redox state variation at different states of charge. The ratio of L3 doublet has a direct correlation with its Ni valence state. At a fully charged state, the doublet peak ratio is high due to the oxidation of Ni2+ ions in the NMC pristine cathode, confirming the reversible Ni redox reaction even at the nano-scale depth region (Fig. 2b).
In summary, the importance of interface stabilization for achieving high temperature stability of the NMC cathodes at 100 oC was evaluated, and the Ni redox reversibility was tracked using surface and bulk-sensitive X-ray spectroscopy techniques. We believe that this interfacial understanding at high temperature will lead to the transformation of ambient temperature Liion battery technology into high temperature applications.
The author would like to thank The Electrochemical Society for awarding him the 2022 F. M. Becket Summer Fellowship. He also would like to thank his thesis advisor, Prof. Leela Arava for constantly providing support for his graduate research. Also, the author would like to thank Dr. Conan Weiland and Dr. Cherno Jaye, NIST, (NSLS-II, Brookhaven National Laboratory) and Dr. Mahalingam Balasubramanian (APS, Argonne National Laboratory) for their excellent support during the synchrotron beamtime experiments.
The Electrochemical Society. DOI: 10.1149/2.F06224IF
Fig. 2. XAS analysis of NMC cathode cycled at different conditions. (a) Ni K edge XANES spectra (b) Ni L edge XAS spectra in TEY mode. (XANES: X-ray Absorption Near Edge Spectroscopy, TEY: Total Electron Yield) (Probing depth ~5 nm)
Sudhan Nagarajan is a PhD student in the Department of Mechanical Engineering at Wayne State University advised by Prof. Leela Arava. Sudhan’s current research focuses on understanding the structure-property relationships of potential cathode materials using advanced synchrotron spectroscopy probes and microscopy investigation for next generation Li-ion batteries. Sudhan actively uses the Brookhaven, Argonne, and Berkeley National Laboratories’ scientific facilities. His work was acknowledged with the 2021 Wayne State University Thomas Rumble Scholar Award; participation in the 23rd National School on Neutron and X-ray Scattering, Argonne and Oak Ridge National Laboratories (July 2021); and 2017 FAER McAfee Scholar Award. The author of 12 articles (h-index 9), he completed a Bachelor of Technology at the Central Electrochemical Research Institute, Tamil Nadu, India, in 2017.
1. K. Xu, Chem. Rev., 104, 4303 (2004).
2. E. Quartarone and P. Mustarelli, Chem. Soc. Rev., 40, 2525 (2011).
3. J. B. Goodenough and Y. Kim, Chem. Mater., 22, 587 (2010)
4. T. M. Bandhauer, S. Garimella and T. F. Fuller, J. Electrochem. Soc., 158, R1 (2011).
5. S. Nagarajan, C. Weiland, S. Hwang, M. Balasubramanian, and L. M. R. Arava, Chemistry of Materials, 34, 4587 (2022).
6. K.-W. Nam, S.-M. Bak, E. Hu, X. Yu, Y. Zhou, X. Wang, L. Wu, Y. Zhu, K.-Y. Chung, and X.-Q. Yang, Advanc Funct Mat, 23, 1047 (2013).
7. J. Alvarado, C. Wei, D. Nordlund, T. Kroll, D. Sokaras, Y. Tian, Y. Liu, and M. M. Doeff, Materials Today, 35, 87 (2020).
Reactive oxygen and nitrogen species (ROS/RNS) are known to play an essential role in cell signaling, disease development and progression.1 The production of ROS/RNS in living cells can be induced by diacylglycerol-lactone (DAG-lactone) through activation of protein kinase C, an important therapeutic target for cancer and other diseases.2 In a previous report, nano-electrochemistry was performed to evaluate the production of ROS/RNS inside a human breast cell (MCF-10A) treated with DAG-lactone.3 Simultaneously, the formation of large intracellular vacuoles was observed using a microscope.3 These results suggest a possibility that the ROS/RNS were stored in the intracellular vacuoles. The experiments carried out during this summer were aimed to elucidate the relationship between the intracellular production of vesicles and ROS/RNS.
Conventional resistive-pulse sensing4,5 was employed under the same experimental conditions as in ref. 3 to find out if nanosized vesicles are formed after adding DAG-lactone. A 120 nm-diameter quartz nanopipette (Fig.1a) was inserted inside an MCF-10A cell, and a voltage of V = 400 mV was applied between the Ag/AgCl reference electrodes (Fig. 1b). The currenttime recording (Fig.1c) shows that many resistive spikes appeared about 21 min after adding DAG-lactone. No similar resistive pulses were detected without DAG-lactone. A typical resistive pulse shown in Fig.1d indicates that a vesicle went through the pipette orifice and partly blocked the ion current. These current blockages suggest that DAG-lactone induces the formation of nanosized intracellular vesicles.
Electrochemical resistive-pulse (ERP) sensing5,6 was used to investigate the correlation between ROS/RNS production and vesicle formation. In an ERP measurement, when a vesicle goes through the pipette orifice, it blocks the faradaic current, producing a resistive pulse (green peak; Fig. 2a). If a vesicle contains redox species (e.g., ROS/RNS in Fig. 2a), the faradaic current spikes caused by oxidation of redox species during its collision with the pipette inner wall (purple peak) follows the resistive pulse. A 160-nm-diameter platinized carbon pipette (Fig.2b), used as a working electrode, was inserted into an MCF-10A cell at V= +850 mV (Fig. 2a). No faradaic current spikes were observed initially. However, numerous current blockages paired with faradaic current
Fig. 1. Monitoring vesicle production within an MCF-10A human breast cell induced by the addition of DAG-lactone via resistive-pulse sensing. (a) TEM image of a 120 nm-diameter quartz nanopipette. (b) Schematic diagram of the experimental setup. (c) Current–time recording inside the cell about 21 min after adding DAG-lactone. (d) Representative current transient labeled with the red asterisk in (c). 10 mM PBS solution contained 10 µM DAG-lactone and 0.1% v/v DMSO, V = 400 mV.
Fig. 2. Electrochemical resistive-pulse sensing of ROS/RNS inside cellular vesicles within an MCF-10A breast cell. (a) Schematic diagram of the experimental setup. (b) TEM image of a 160-nm-diameter platinized carbon pipette. (c) Current–time recording inside the cell about 43 min after adding DAGlactone. (d) Representative current transient labeled with the red asterisk in (c). 10 mM PBS solution contained 1 mM FcMeOH, 10 mM K4[Fe(CN)6], 10 µM DAG-lactone and 0.1% v/v DMSO, V = +850 mV vs Ag/AgCl. (continued on next page)
from previous page)
spikes appeared in about 43 min after adding DAG-lactone (Fig. 2c). A typical current transient (Fig. 2d) was produced by a vesicle going through the pipette orifice and colliding with the inner surface. The ROS/ RNS contained in the vesicle were released during the collision and oxidized at the platinum surface. The results indicate that the produced intracellular vesicles contained ROS/RNS.
In summary, we monitored in real-time the formation of intracellular vesicles in response to cell treatment with DAG-lactone and established the presence of ROS/RNS in those vesicles. The results can improve our understanding of oxidation stress and related processes in biological cells. The goal of our future work is to establish the relationship between the ERP amplitude and the vesicle size and estimate the concentrations of ROS/ RNS in single vesicles. This approach is potentially useful for evaluating the efficacy of therapeutic agents aimed at inducing oxidative stress to trigger cell death, by measuring ROS/RNS concentration in vesicles.
The Electrochemical Society. DOI: 10.1149/2.F07224IF
The author gratefully thanks ECS for the support provided through the H. H. Uhlig Summer Fellowship. The author would also like to thank her advisor, Dr. Michael V. Mirkin, for his guidance and support.
Rui Jia is a fifth-year PhD student at the Graduate Center, City University of New York, doing research in Prof. Michael V. Mirkin’s group at Queens College, CUNY. Her research interests include the development of nanopipette-based sensors, electrochemical analysis, and bio-electrochemistry.
1. H. Sies, V. V. Belousov, N. S. Chandel, M. J. Davies, D. P. Jones, G. E. Mann, M. P. Murphy, M. Yamamoto, and C. Winterbourn, Nat Rev Mol Cell Biol, 23, 499 (2022).
2. V. E. Marquez and P. M. Blumberg, Acc Chem Res, 36, 434 (2003).
3. Y. Li, K. Hu, Y. Yu, S. A. Rotenberg, C. Amatore, and M. V. Mirkin, J Am Chem Soc, 139, 13055 (2017).
4. Y. Wang, D. Wang, and M. V. Mirkin, Proc R Soc A, 473, 2016093 (2017).
5. R. Pan, K. Hu, R. Jia, S. A. Rotenberg, D. Jiang, and M. V. Mirkin, J Am Chem Soc, 142, 5778 (2020).
6. R. Pan, K. Hu, D. Jiang, U. Samuni, and M. V. Mirkin, J Am Chem Soc, 141, 19555 (2019).
Dr. David Lockwood’s research-work-related photograph entitled “Raman spectroscopy of solids” received Honorable Mention in the category “At a Glance” of the International Union of Pure and Applied Physics 100th Anniversary (IUPAP 100) Photo Contest and was exhibited at UNESCO headquarters in Paris, France, on July 8, 2022 for the opening ceremony of the International Year of Basic Sciences for Sustainable Development (IYBSSD2022). His entry was chosen for the cover of the UNESCO booklet that was distributed at the UNESCO exhibit. A copy of the cover is shown to the right. The 12 winning entries were also presented on 13 July 2022 during the IUPAP 100 Symposium hosted by the ICTP in Trieste, Italy.
For over 11 years, Dr. Kailash Mishra has served our Society as Technical Editor of Luminescence and Display Materials, Devices, and Processing for the ECS Journal of Solid State Science and Technology (JSS). In October 2023, he will end his 12-year term as an editor of the journal.
A Fellow of the Electrochemical Society, Kailash has been a very active and dedicated member of ECS since he joined the Society in 1998. He became a member of the Editorial Board of the Journal of the Electrochemical Society in 2011. In the following year, JSST was launched, and shortly thereafter Kailash created the first-ever focus issue for an ECS journal. To date, Kailash has organized five focus issues. Each issue has had a great impact on the phosphor community.
Kailash served as the Chair of the Luminescence and Display Materials (LDM) Division of ECS from 2009 to 2011. His enthusiasm for advancing the division is unmatched. He has organized at least
five symposia for the division, which have attracted speakers with great international reputation. They have not only added great value to the division, but have also yielded five focus issues of JSS with articles contributed by those invited speakers.
Kailash is now retired from Osram Sylvania, having worked for over 32 years in the phosphor research area as both an individual researcher and a research manager. He is an internationally renowned scientist in the field of luminescent materials. He has published more than 100 papers in leading journals, and co-edited several books and proceedings volumes.
Thanks to his great contributions to the ECS journals and great achievements in the fundamental research of luminescent materials, Kailash was selected as the 2022 winner of the LDM Division award.
During his 12-year tenure as Technical Editor, his diligence, dedication, and positive spirit have greatly improved the reputation and the popularity of the ECS journals, helping them better serve both the academic and the industrial communities.
Interface is searching for People News for upcoming issues. If you have news you would like to share with the Society about a promotion, award, retirement, or other milestone event, please email the content to: kara.mcarthur @electrochem.org.
1940–2022
Michael J. Graham of Ottawa, Canada, long-time member of the Corrosion Division and recipient of the H. H. Uhlig Award, died on August 2, 2022, after courageously living with cancer over several years. He was 82. Dr. Graham was born on March 21, 1940, in Carlisle, UK. His early education was at the Creighton School in Carlisle. He went on to the University of Liverpool, where he was awarded his BSc in Physical and Inorganic Chemistry (1962) and PhD in Surface Chemistry (1965).
Following Liverpool, he began a 36-year career at the National Research Council of Canada, starting as a post-doctoral fellow and progressing in 1990 to Principal Research Officer. Michael is survived by his wife and dear friend of many years, Lynda Pilkington, his daughter, Karen Lavallee, his sons, John and Andrew, and the mother of his children, Marion Payne.
Over Michael’s research career he published more than 250 papers on his work in corrosion science and engineering, thin oxide film formation on metals and semiconductors, surface passivation, high temperature oxidation, and the application of surface analytical techniques to degradation of materials. His leadership responsibilities
Royce Wilton Murray, Kenan Professor Emeritus of Chemistry at the University of North Carolina at Chapel Hill, died on July 6, 2022 at the age of 85. Dr. Murray was a leading scholar in the field of Analytical Chemistry and Electrochemistry. He was born January 9, 1937 in Birmingham, Alabama. He graduated from Birmingham Southern College with a major in Chemistry in 1957 and went on to Northwestern University to complete his PhD in Analytical Chemistry in 1960. That same year he joined the faculty of the Chemistry Department at the University
at the National Research Council of Canada included Head of Metallic Corrosion and Oxidation, Head of Chemical Characterization, Head of Surfaces and Interfaces, and Chemistry Division representative to NRC-wide planning and management committees.
The high esteem his research colleagues have for his work is evident in the many awards and recognition he received over his career, including the Queen Elizabeth Diamond Jubilee Medal (2002), U. R. Evans Award of the UK Institute of Corrosion (1994), W. R. Whitney Award of NACE (2003), the T. P. Hoar prize of the UK Institute of Corrosion Science and Technology (1983), Chair of the International Corrosion Council (1996), Chair of the Gordon Research Conference on Corrosion (1985), Co-chair of the 9th International Congress on Metallic Corrosion (1984), Fellow of NACE (1994), and Fellow of the UK Institute of Corrosion.
In addition to his love for research in surface science, Michael was an avid golfer, captained his cricket club in Ottawa, and played bridge nearly weekly with close friends for over 40 years. Michael will be particularly remembered for his love of the Gordon Research Conferences on Corrosion where he was an active participant in the science and recreational aspects of all but one Conference over 47 years. He will be remembered for his sharp mind, determination, fairmindedness, soft-spoken nature, and genuine kindness.
This notice was contributed by Doug Sinclair, AT&T Bell Labs (retired).
of North Carolina at Chapel Hill, where he spent 55 years teaching and perfoming research. He was chair of the Chemistry Department from 1979 to 1984. He was an internationally renowned scholar, pioneering research in the areas of chemically modified electrodes and the electrochemistry of superconductors and nanoparticles.
Dr. Murray joined ECS in 1987 and was a long-standing member of the PAE Division. He was recognized as an Awarded Life Member, received the Carl Wagner Memorial Award in 1987, was elected a Fellow of The Electrochemical Society in 1995, and received the Olin Palladium Award in 1997. Among many other honors in his career was election to the National Academy of Sciences and the American Academy of Arts and Sciences in 1991, and in 2009, the naming of the University of North Carolina at Chapel Hill Chemistry Department building in his honor.
In the last few years, hydrogen has been elevated to a new position as the key player in the decarbonization efforts in line with the commitments to reduce emissions of greenhouse gases (GHGs), achieve carbonneutrality by 2050, and reach the Paris Agreement targets to limit global warming to 1.5°C, compared to pre-industrial levels.1-4 The potential of hydrogen in the transition to clean energy was discussed in detail in the Winter 2021 issue of Interface, which was entirely dedicated to the multisectoral use of hydrogen produced from diverse pathways in a wide range of key sectors. I contributed to that
Overall, Hydrogen has been gaining momentum across all sectors and roadmaps and among policy makers, with growing interest in clean “green” hydrogen due also in part to recent developments in the United States, such as:
• The US Department of Energy’s Hydrogen Earthshot, which aims to reduce the cost of clean hydrogen by 80% to $1 per 1 kilogram in 1 decade (“1-1-1”).
• The Infrastructure Investment and Jobs Act (US Congress HR3684) which defined clean hydrogen to mean hydrogen produced with a carbon intensity ≤ 2 kgCO2-equivalent/kg(H2).
• The announcement of the Regional Clean Hydrogen Hubs Project to support the development of clean hydrogen hubs to improve clean hydrogen production, processing, delivery, storage, and end use.
• The Inflation Reduction Act (IRA), which, among many provisions on clean energy, creates a new tax credit for the production of qualified clean hydrogen during a specified 10-year period. The act defines qualified clean hydrogen as hydrogen that is produced through a process that results in a lifecycle greenhouse gas (GHG) emissions rate of not greater than 4 kgCO2-eq./kg(H2).
• The US Department of Energy released a Draft Guidance in September 2022 to guide development of a Clean Hydrogen Production Standard (CHPS). Although not released as a regulatory standard, the document discusses the concepts and definitions set forth in the BIL (for “Clean Hydrogen”) and then in the IRA (for “Qualified Clean Hydrogen”).
Similar policy efforts for setting emissions thresholds have been implemented in Europe. In accord with the REPowerEU plan of 2022, which includes renewable hydrogen production, the European Commission recently published drafts that propose requirements for establishing a minimum threshold of 70% for greenhouse gas emissions savings of recycled carbon fuels.16 By using the fossil fuel comparator set at 94 gCO2eq/MJ, the GHG emission threshold for hydrogen becomes 3.38 kgCO2-eq./kg(H2) 4,16 This value is 70% lower than that of the fossil fuel comparator, including transport and other non-production emissions.4
issue with a Chalkboard article, Colors of Hydrogen, that discussed the status of the current and emerging hydrogen production pathways (Fig. 1) by presenting an updated view of the hydrogen colors paradigm based on a survey of many hydrogen reports and roadmaps.5 Most reports have since then continued to highlight, with growing emphasis, the pivotal role of hydrogen in helping the world achieve carbon neutrality and transform the clean energy sector,2-4,6-15 including the recently announced Clean Hydrogen Production Standard by the US Department of Energy and Global Hydrogen Review by the International Energy Agency (IEA).4
All these announcements and developments have not only fueled (pun intended) the role of clean hydrogen as part of a clean energy vision, but also intensified the communication of clean hydrogen, hydrogen colors, and emissions. While the abovementioned reports focus on the definition of clean hydrogen, in general, the term green has been adopted favorably, not only for hydrogen, but even for commodities and products made of green hydrogen; for example, green ammonia, green steel, and green cement. In fact, a quick review of recent reports on hydrogen reveal a wide range of vocabulary/ terminology discussed in context of clean hydrogen:
• Green hydrogen
• Sustainable hydrogen
• Zero-carbon hydrogen
• Near-zero carbon hydrogen
• Low-carbon hydrogen
• Decarbonized hydrogen
• Renewable hydrogen
• Electrolytic hydrogen
This article discusses hydrogen production pathways with a focus on the ongoing transition in the description of clean hydrogen from colors to an emissions-based definition of its cleanliness (Fig. 2). Thus, this article builds on the previous article5 with the hope of demystifying colors and extends the discussion on the limitations of color-coded hydrogen by providing a refined critique and assessment based on the emissions-pathways paradigm.
Hydrogen is an energy carrier that is the simplest chemical bond (i.e., between two hydrogen atoms). It can be produced by converting a diverse array of feedstocks that serve as the material source through processes that drive reactions (thermochemical, biochemical, (photo) electro-chemical) with energy input. These processes also lead to byproducts and/or carbon emissions, especially if the feedstock contains carbon atoms, or uses energy or heat that releases carbon emissions as part of the process. Thus, hydrogen production pathways can be classified in different ways, depending on the (i)
process, (ii) feedstock (material source), (iii) energy source, and (iv) resulting emissions (Fig. 1), with each pathway coming with a certain level of carbon (dioxide) emitted to the atmosphere. To ease the conversations on hydrogen production, these classifications have been simplified to a color-coding, with gray, blue, and green hydrogen the most common.5 The current and emerging pathways for hydrogen production pathways are visualized in Fig. 1 with the commonly used color-coding associations (shown in italic in parentheses).
The production process is organized based on the four key pathways, instead of colors:
1. Thermochemical pathways (from fossil fuel, including steam reforming of natural gas or coal gasification)
2. Biopathways, from reforming or pyrolysis of biogas to biomass gasification
3. Electrolytic pathways, from water-splitting using electricity
4. Advanced solar pathways, from solar thermochemical hydrogen to photo-biological processes
This list is not definitive, nor does it list all advanced pathways; rather it illuminates one way to reorganize the pathways in preparation for the ensuing discussion (Fig. 2).
As discussed in the previous article focusing on colors,5 and based on the pathways visualized in Figs. 1 and 2, the current color coding fails to capture all the hydrogen production pathways. In their Global Hydrogen Review report,14 the International Energy Agency (IEA) noted that “There is no international agreement on the use of these terms [colors] as yet, nor have their meanings been clearly defined.” For example, in IEA reports, low-carbon hydrogen includes those produced from renewable and nuclear electricity, biomass, and fossil fuels with Carbon Capture Utilization and Sequestration (CCUS), used without any color coding.4,14
Some of the limitations of the ill-defined color assignments can be summarized as follows.
Colors are defined based on a mixed bag of categories; pathway (processes), sources (electricity or fuels), or carbon emissions (high vs. low vs. zero), with no consistent nomenclature. For example, gray represents natural gas and steam-methane-reforming (SMR) (source + process) with high emissions; blue represents natural gas and SMR (source + process) with captured emissions (CCUS). But there is no emission threshold as to what capture rate constitutes blue hydrogen, which rather creates “shades of blue,” adding a level of uncertainty to color coding.
What is the color of direct-solar pathways? For example, solar thermochemical hydrogen (STCH) uses a thermochemical process to split water using solar heat. If this process produces green hydrogen, then not all green hydrogen comes from electrolysis. Thus, the definition of green hydrogen should expand to include other nonelectrolytic water-splitting processes as well.
Moreover, the colors are linked only to the pathways currently in use today, which begs the question: What is the color of biomass pathways? If one uses biogas (a.k.a. renewable natural gas) for stream reforming, would that also be gray or blue hydrogen? Or what would be the color of hydrogen from pyrolysis of biogas? Then, should turquoise hydrogen represent the pyrolysis process only, regardless of the feedstock?
Assuming that colors should also represent emissions, a key question emerges: Do the emissions refer to the emissions at the site of hydrogen production, or life-cycle carbon intensity? The term “green” hydrogen commonly refers to the former, and is therefore associated with zero-emissions. If, however, one accounts for life-cycle emissions, then the emission values would shift based on well-to-gate life-cycle emissions accounting for both upstream and downstream emissions, including leakage, transportation, delivery, processing, storage, materials, and losses. In fact, in DOE’s recent Clean Hydrogen Production Standard Guidance, a system boundary for life cycle targets included all key emissions sources associated with feedstock extraction or production, generation of electricity, feedstock delivery, hydrogen production, potential releases during CO2 transport, and carbon capture and sequestration of GHGs generated by the production process.
For example, while biopathways used in gasification or reforming could generate emissions during production, these could be captured
Fig. 1. Hydrogen production pathways illustrating the process, energy source, material/feedstock source, and emissions. The traditional color-coding is added to the hydrogen shown in italic in parentheses below the carbon-based definition of hydrogen, when available..
Electrochemical Society Interface
Fig. 2. Illustration of transition from (a) color-coded hydrogens to (b) hydrogen pathways that recognize the diverse source of feedstocks and energy input for major pathways with possible range for their associated emissions shown relative to each other (without absolute measures). “CCUS” refers to Carbon Capture, Utilization, and Storage.
on site. Thus, including life cycle emissions could lead to “negative” emissions due to the removal of carbon dioxide that would otherwise be released to air. For example, a Lawrence Livermore Foundation report on California noted that conversion of biomass to hydrogen using gasification with CCUS generates recoverable CO2, because nearly all of the carbon in biomass is captured during processing.8 With the emergence of bio and solar pathways, a wider range of technologies will be able to create clean hydrogen with near-zero emissions or even negative emissions, for certain biomass pathways, if life-cycle emissions are considered.2,10,17
All hydrogen from electrolysis with renewables (electricity) can be defined as “green,” but not all “green” hydrogen is necessarily electrolytic—as there are other pathways that can drive water-splitting reaction and produce green hydrogen with near-zero life-cycle emissions
Due to lack of standards that have been internationally agreed upon and adopted for clean hydrogen, the IEA differentiates the types of hydrogen by their production technology (pathway), and uses this as the basis for their current definition of low-carbon hydrogen.”14 In a similar vein, the Hydrogen Council defines green/renewable hydrogen as “produced from water electrolysis with renewable electricity, or alternatively reformed biomass with CO2 sequestration.”15
Some of the confusion in terms of using colors and defining clean hydrogen are rooted in 4, 14:
• Unclear use of dedicated vs. by-product hydrogen
• Use of blue hydrogen to indicate CCUS (carbon capture) but without any value as to what the CCUS entails in terms of the capture/utilization rate or the emission (reduction)
• Use of electrolytic hydrogen but without disclosing the source and emissions intensity of electricity
• Lack of clarity around whether emissions represent life-cycle emission or not
Of course, one may expect revision of these definitions and development of new international standards as conversations within the hydrogen community evolve.14,15 New or refined definitions could also lead to adoption of technology-agnostic emission-based definitions by de-emphasizing (or modifying) color coding due to its above-listed limitations (Fig. 2).
The carbon intensity of these hydrogen production processes varies widely depending on the technology as well as the analysis. Currently, almost the entire hydrogen demand in the world has been met by hydrogen produced from fossil fuels without CCUS. In 2021, the total hydrogen production in the world was 94 Million metric tonnes (Mt), 74 Mt of which was pure H2 4 The emissions from the global hydrogen production was over 900 Mt CO2 4 This results in an average value of 9.57 kgCO2-eq./kg(H2), which can be used as a comparison (starting) point.
According to one IEA report (2019),17 the CO2 intensity of hydrogen production from natural gas (via SMR) decreases from 9–10 kgCO2/kgH2 to ~4 kgCO2/kgH2 (with a CCUS capture rate of 56%), which is lower than the emissions of 17 kgCO2/kgH2 for electrolysis if one uses the world average for emissions from gasfired electricity.17 However, the emission values drop significantly to near-zero for electrolytic H2 if renewable electricity is used instead to drive water splitting to produce “green” hydrogen. Hence, one of the primary drivers of the emission intensity for electrolytic hydrogen is the carbon intensity of electricity generation (kgCO2/kWh). A quick examination of IEA’s Net-Zero Emissions (NZE)2,3 pathway analysis for CO2 emissions from future electricity generation reveals insights into the feasibility of clean hydrogen from electrolysis as an increasing share of renewable electricity toward 2050 with a projected negative carbon intensity by 2040 (Fig. 3).
The question is then how the emissions of hydrogen production change on a life-cycle basis for different pathways. Fig. 4 shows a comparison of life-cycle emissions for several pathways based on the DOE’s GREET analysis framework hosted by Argonne National Laboratory.18 The figure clearly shows the reduction in emissions when fossil fuels are used with carbon capture and a further decrease with the use of biomass gasification and gas reforming.18 Similarly, a 4 kgCO2eq/kg(H2) target can be achieved via electrolysis using low-carbon and clean energy sources. What stands out from Fig. 4, however, is the negative emissions for municipal solid-waste digestion, which underscores the potential of biomass pathways along with the value of life cycle carbon intensity assessment. Based on these values, many of the pathways could be capable of generating “clean hydrogen.”
Fig. 4. Emissions from hydrogen production methods, based on data (GREET model) from A. Elgowainy.18 (Ranges shown to reflect potential variability in upstream methane leak rates, plant energy efficiency, and CO2 capture rates. Baseline assumes 90% capture rate).18 The shaded area between 0 and 4 is added to show the thresholds for qualified clean hydrogen defined by IRA.
Recognizing the diverse pathways for hydrogen production, and the importance of its carbon footprint (over the entire life cycle), will likely lead to a re-organization of the categories and a refinement of “clean hydrogen” which now lacks a universal definition, or a methodological basis to calculate the carbon footprint for hydrogen production, although emission thresholds are a topic of active debate.11,12,14,15,19 The concept of emission thresholds based on life-cycle intensity has been adopted in the most recent Inflation Reduction Act, which uses a set of thresholds up to a 4 kgCO2equivalent/kg(H2) to define emission thresholds and intervals to incentivize decarbonized, clean hydrogen production (Fig. 5). The most recent IEA report noted the importance of regulations, including a “threshold of the emissions intensity of hydrogen production needed to qualify for policy benefits.”4 The IRA and similar efforts serve as examples toward such regulations and policy efforts.
Notwithstanding the ill-defined associations of color coding, the concept of colors is reaching a saturation point where it can no longer support the new pathways (bio, solar) with new colors as it already uses most of the primary colors (in the color wheel) (Fig. 6a). This requires a transition from the “color wheel” in favor of a more practical
and realistic system based on the carbon intensity of emissions, which can be seen as an intensity wedge (Fig. 6). By focusing on “pathways + intensity” instead, we can recognize the diverse pathways to produce hydrogen with low or zero emissions in a technology-agnostic manner (Fig. 6b). Thus, we go from the many colors of hydrogen to the many ways to make clean (or ‟green”) hydrogen. It must be noted, however, that if life-cycle emission is to be adopted, then the negative potential of certain bio pathways must be accounted for, which causes a shift in the intensity range (wedge) (Fig. 6b to c). Thus, one way to interpret the visualization of this paradigm shift to emissions-based classification is moving from various color (hues) to shades of green, from dark green (low-carbon) to green (zero-carbon / renewable) to light green (net negative emissions). This graphical demonstration (Fig 6) is not meant to provide definitive guidance but is rather used to help illustrate the paradigm shift and the limitations of the concept of the color wheel by using visual examples.
With the increasing emphasis on decarbonization goals, environmental justice, and net-neutrality efforts, clean hydrogen’s role is recognized more than ever, from roadmaps to net-zero scenarios, to clean energy bills and regulations. These concerted activities could benefit from effective means of communication, including new perspectives on clean hydrogen. This article serves as a collection
Fig. 3. Carbon intensity of electricity generation in the world, based on historical averages and the projections under the Net-Zero Emissions by IEA (data taken from NZE report and World Energy Outlook).2,3
Fig. 5. Decarbonized Hydrogen Tax Credit of Inflation Reduction Act of 2022. Tax eligibility percentage and credit for clean hydrogen production depending on its greenhouse gas (GHG) emissions on a life-cycle basis.
Fig. 6. A conceptual illustration of the transition from (a) the traditional “color wheel” concept for hydrogen to (b) an alternative representation of carbon emissions-based definition of hydrogen “intensity wedge.”
of notes in light of recent developments and interest in categorizing (clean) hydrogen production, especially with the enhanced focus on the transition to emissions intensity (Fig. 2). The above discussion is by no means definitive, as some colors’ associations may begin to fade in favor of carbon intensity of the hydrogen production processes, reduction of which is the primary driver behind R&D and deployment efforts, policies, and regulations for achieving a sustainable and equitable clean hydrogen ecosystem.
The views and opinions of the authors expressed herein do not necessarily state or reflect those of the United States Government or any agency thereof. Neither the United States Government nor any agency thereof, nor any of their employees, makes any warranty, expressed or implied, or assumes any legal liability or responsibility for the accuracy, completeness, or usefulness of any information, apparatus, product, or process disclosed, or represents that its use would not infringe privately owned rights.
The Electrochemical Society. DOI: 10.1149/2.F08224IF
Ahmet Kusoglu, Staff Scientist, Lawrence Berkeley National Laboratory Education: PhD (University of Delaware), Post-doctoral research at LBNL on fuel-cell membranes’ durability and performance.
Research Interests: Ionomers, Polymer Electrolytes, Mechanochemistry, StructureProperty Relationships, Hydrogen Technologies, Fuel Cells and Electrolyzers. Pubs + Patents: >75 peer-reviewed journal papers, 2 book chapters, >30 invited talks and tutorials at international meetings, academia, and industry forums.
Work Experience: Communications Director of the MillionMile Fuel Cell Truck (M2FCT) Consortium, and studies data visualization and data-driven stories on energy and environmental topics, including hydrogen and electrochemistry.
Awards: Srinivasan Young Investigator Award and ECS Toyota Young Investigator Fellowship.
Work with ECS: Contributing Editor, ECS Interface; ECS member for 10+ years; Member-at-large, Energy Technologies Division (ETD), Symposium co-organizer for Polymer Electrolyte Fuel Cells and Electrolyzers.
Website: https://kusoglulab.lbl.gov https://orcid.org/0000-0002-2761-1050
1. V. Masson-Delmotte, P. Zhai, A. Pirani, S.L. Connors, C. Péan, S. Berger, N. Caud, Y. Chen, L. Goldfarb, M.I. Gomis, M. Huang, K. Leitzell, E. Lonnoy, J.B.R. Matthews, T.K. Maycock, T. Waterfield, O. Yelekçi, R. Yu, and B. Zhou, in Climate Change 2021: The Physical Science Basis. Contribution of Working Group I to the Sixth Assessment Report of the Intergovernmental Panel on Climate Change, V. Masson-Delmotte, P. Zhai, A. Pirani, S.L. Connors, C. Péan, S. Berger, N. Caud, Y. Chen, L. Goldfarb, M. I. Gomis, M. Huang, K. Leitzell, E. Lonnoy, J. B. R. Matthews, T. K. Maycock, T. Waterfield, O. Yelekçi, R. Yu, and B. Zhou, Editors, p. 33, Cambridge University Press, Cambridge, UK (2021).
2. IEA, Net Zero by 2050, IEA, Paris (2021).
3. https://www.iea.org/topics/world-energy-outlook
4. IEA, Global Hydrogen Review 2022, IEA, Paris (2022).
5. A. Kusoglu, The Electrochemical Society Interface, 30, 44 (2021)
6. BloombergNEF, Tracking the Hydrogen Economy (2021).
7. Rhodium Group, Clean Hydrogen: A Versatile Tool for Decarbonization (2021).
8. Livermore Lab Foundation, Getting to Neutral: Options for Negative Carbon Emissions in California (2020).
9. Hydrogen Council, Hydrogen Decarbonization Pathways (2021).
10. US Department of Energy, DOE Hydrogen Program Plan (2020).
11. IEA, Hydrogen Tracking Report (2020).
12. IRENA, Green Hydrogen: A Guide to Policy Making (2020)
13. Rocky Mountain Institute, Hydrogen’s Decarbonization Impact for Industry (2020).
14. IEA, Global Hydrogen Review (2021).
15. Hydrogen Council, Hydrogen for Net-Zero: A Critical CostCompetitive Energy Vector (2021).
16. European Commission, Ares(2022)3836721 (2022).
17. IEA, The Future of Hydrogen (2019).
18. A. Elgowainy, GREET® Model for Life Cycle Analysis of Greenhouse Gas Emissions, in Department of Energy, H2IQ webinar (2021)
19. Hydrogen Council and McKinsey & Company, Hydrogen Insights Report (2021).
+ -
In this installment of the ‟Looking at Patent Lawˮ articles, we illustrate a high-level perspective of the impact of electrochemical science and engineering on innovation and technology development, as evidenced by inclusion within the patent literature.
The impact of electrochemical science and engineering that can be observed within the patent literature was previously indicated by the large number of citations to Journal of The Electrochemical Society articles in patents and patent applications.1 Specifically, during the time period 01/01/2010 to 02/14/2019, JES received five percent of the citations relative to other journals within The Electrochemical Society’s technical domain, a higher percentage than all other electrochemistry journals combined. In view of the importance of prior art citations in examining inventions at the United States Patent & Trademark Office (USPTO), journal citations in patents and patent applications should be included as a factor in evaluating journal quality.2 In this article, we take a broader perspective in considering the impact of electrochemical science and engineering on patented technological innovations.
We searched the USPTO patent database for the past 31 years using commercially available PatSnap software.a For this preliminary scan of the patent literature, we could have also used the publicly available patent search tools at the USPTO3 or Google Patents.4 The search was limited to US patents, which seemed reasonable as most patents are either first filed in the United States or first filed in a foreign jurisdiction and subsequently filed in the United States. The Boolean search string was “electrochem* OR electroly*” for US patents issued within the date range of 01/01/1991 to 12/31/2021. The “wildcard” search terms “electrochem*” and “electroly*” identified patents with the term electrochemistry, or electrochemical, or electrolysis, or electrolye, or electrolyzer within the written description of the patent.
In this article, we compare the total number of patents issued within a given time period to those patents directed toward electrochemical science and engineering, as defined by the query terms “electrochem*” OR “electroly*” in the specification. In addition, we present the trend of electrochemical science and engineering patents relative to all patents issued over the 31-year period. Finally, we present the most prevalent assignees/owners and inventors associated with the electrochemical science and engineering patents.
aAvailable from Patsnap Pte Ltd, Chiswick High Road London W4, 5YA United Kingdom (www.patsnap.com)
4.00% CAGR
Fig. 1. All US patents issued by year for the period 1991 through 2021 (CAGR – Compound Annual Growth Rate).
We searched US patents issued in the 31-year timeframe 01/01/1991 to 12/31/2021. During this timeframe, 6,234,813 US patents were issued. Of the total number of issued patents, 275,685 were related to electrochemical science and engineering as defined by the query terms “electrochem*” OR “electroly*.” The search query breadth was quite large and, as an example, returned US Patent No. 10,981,040 (issued April 20, 2021) directed toward “electrochemical milling” of a novel golf club head issued to Callaway Golf Company.
Fig. 2. Electrochemical Science and Engineering US patents issued by year for the period 1991 through 2021 (CAGR – Compound Annual Growth Rate).
We present the number of US patents (Fig. 1) and electrochemical science and engineering–related US patents (Fig. 2) by year for the 31-year period. Electrochemical-related patents represent 4.4% of all patents issued over the 31-year period.
The number of US-issued patents in Fig 1. and Fig. 2 generally follow similar trends, suggesting that “all” and “electrochemical” patents are subject to similar “external” forces that would impact the decision to file. Note that the time from patent filing to patent issue is approximately three to four years depending on the technology unit. Consequently, whatever these “external” forces are, there is a three-to-four-year lag in time. Both “all” and “electrochemical” USissued patents are trending upward over time. However, the compound annual growth rate (CAGR) for “all” patents and “electrochemical” patents are approximately 4.00 and 5.25%, respectively. Consequently, electrochemical science and engineering patents represented 3.2% of all patents in 1991 and 4.7% of all patents in 2021. In 2017 and 2018, the fraction of electrochemical science and engineering patents peaked at 4.9% of all patents. Over the past ten years, “electrochemical” patents represented nearly 5% of “all” issued patents. Assuming that the higher CAGR in electrochemical patents is significant, this data would suggest that the relative importance of electrochemical science and engineering was increasing compared to other technologies during this time.
From 2009 to 2021, the number of “all” issued patents and “electrochemical” issued patents began to increase faster. Specifically, the CAGR during this period was 5.75 and 6.76% for “all” and “electrochemical” issued patents, respectively. The increasing relative importance of electrochemical science and engineering seems logical as the electrochemical arts continue to play an important role in both traditional and new technologies, and in particular are at the forefront of addressing many issues associated with sustainability.5
In Fig. 3, we present the top 20 assignees of the electrochemical science and engineering patents as defined by the search query “electrochem*” OR “electroly*” for the 31-year period 1991 to 2021. Representatives from nearly all these companies have attended and/ or presented research talks at biannual ECS meetings, or published in ECS journals. The top assignee of electrochemical patents, Toyota, sponsors The ECS Toyota Young Investigator Fellowship to
In Fig. 4 we present the top 40 inventors on the electrochemical science and engineering patents as defined by the query “electrochem*” OR “electroly*” for the 31-year period 1991 to 2021. Nine of these top forty inventors appear in the ECS database as either being an ECS member or having attended an ECS biannual meeting.
Dr. Shunpei Yamazaki is the top inventor, with 870 patents during the 1991 to 2021 period. Dr. Yamazaki is an Emeritus member and a Fellow of the ECS. Interestingly, Dr. Yamazaki is employed by Semiconductor Energy Laboratory Co. Ltd., which is the fifth largest assignee of electrochemical patents (see Fig. 3).
Dr. Adam Heller is the inventor on 256 electrochemical patents during the 1991 to 2021 period. Dr. Heller is an Honorary member and a Fellow of the ECS. In 2007, Dr. Heller received the Presidential National Medal of Technology and Innovation for contributions to lithium batteries and glucose monitoring, specifically6
“… fundamental contributions to electrochemistry and bioelectrochemistry and the subsequent application of those fundamentals in the development of technological products that improved the quality of life of millions across the globe, most notably in the area of human health and well-being.”
Dr. Heller is co-inventor on the foundational patent “Enzyme Electrode” which permitted glucose monitoring at home by people with diabetes.7 The “Enzyme Electrode” patent was the subject of a “Looking at Patent Law” case study.8 Dr. Heller has received numerous ECS awards, including the Battery Division Award in 1978, the Physical and Analytical Electrochemistry Division David C. Grahame Award in 1987, the Vittorio de Nora Gold Medal in 1988, and the Europe Section Heinz Gerischer Award in 2015. Dr. Heller is the subject of an ECS Masters podcast.9
Fig. 3. Assignees of Electrochemical Science and Engineering US patents issued by year for the period 1991 through 2021.
“… encourage young professionals and scholars to pursue research into batteries, fuel cells and hydrogen, and future sustainable technologies.”5.25% CAGR
Dr. Esther Takeuchi is the inventor on 144 electrochemical patents during the 1991 to 2021 period. Dr. Takeuchi was awarded Lifetime membership and is a Fellow of the ECS. In 2008, Dr. Takeuchi received the Presidential National Medal of Technology and Innovation,10
“For her seminal development of the silver vanadium oxide battery that powers the majority of the world’s lifesaving implantable cardiac defibrillators, and her innovations in other medical battery technologies that improve the health and quality of life of millions of people.”
Dr. Takeuchi is co-inventor on the foundational patents leading to the successful commercialization of defibrillators.11,12 Dr. Takeuchi has received numerous awards for her inventions, including induction into the National Inventors Hall of Fame (2011),13 and is recipient of the European Inventor Award (2018).14 Dr. Takeuchi was recently elected member of the American Academy of Arts and Sciences (2021).15 One of Dr. Takeuchi’s more recent patents, directed toward “Electrochemical Eradication of Microbes on Surfaces of Objects,” was the subject of a “Looking at Patent Law” case study.16
Dr. Takeuchi has received numerous ECS awards, including the Battery Division Technology Award in 1995 and the Edward Goodrich Acheson Award in 2020. Dr. Takeuchi served as ECS President from 2011 to 2012. She is the subject of an ECS Masters podcast.17
In addition to these well-known ECS members, the search identified Nathan P. Myhrvold, who served as Chief Technology Officer at Microsoft Corp. Mr. Myhrovold is a co-inventor on US Patent No. 10,668,305 (issued June 2, 2020) directed toward “garments for therapeutic stimulation” employing electrochemical sensors.
In this installment of the “Looking at Patent Law” articles, we illustrate a high-level perspective of the impact of electrochemical science and engineering on innovation and technology development, as evidenced by the patent literature. We searched the United States Patent & Trademark Office (USPTO) database for US patents issued from 01/01/1991 to 12/31/2021. Over the past ten years, “electrochemical” patents represent nearly 5% of “all” issued patents. The increasing relative importance of electrochemical science and engineering seems logical as the electrochemical arts continue to play an important role in both and new traditional technologies and are at the forefront of addressing many issues associated with sustainability. The top assignee of “electrochemical” patents was Toyota, an Institutional member of the ECS and sponsor of the ECS Toyota Young Investigator Fellowship directed toward sustainable technologies. Most of the top assignees are represented by attendees or presenters at ECS biannual meetings. The top 40 inventors of “electrochemical” patents include a number of prominent ECS members. Dr. Shunpei Yamazaki is an ECS Fellow and the number one inventor with 870 patents. Dr. Adam Heller and Dr. Esther Takeuchi are both ECS Fellows and recipients of the Presidential National Medal of Technology and Innovation.
The current search was limited to issued US patents. Subsequent searches could be expanded to patent applications, as many do not issue as patents but constitute a significant corpus of prior art and technology. Subsequent searches will be narrowed with focused search terms and/or patent classification codes to focus on specific technologies within electrochemical science and engineering to align with The Electrochemical Society’s Divisions and Technical Interest Areas (TIAs).
The authors acknowledge the diligent efforts of Mr. Shannon C. Reed, MBA, Director of Community Engagement at The Electrochemical Society, for his diligent and thorough efforts in identifying the connections of the inventors cited in this article to The Electrochemical Society. The authors gratefully acknowledge Ms. Barbara Taylor for content review.
© The Electrochemical Society. DOI: 10.1149/2.F09224IF
E. Jennings Taylor, Founder of Faraday Technology, Inc. Research Interest: Faraday Technology, Inc. is a small business focused on developing innovative electrochemical processes and technologies based on pulse and pulse reverse electrolytic principles. Patent Background: Taylor leads Faraday’s patent and commercialization strategy and has negotiated numerous patents via field of use licenses as well as patent sales. He is admitted to practice before the United States Patent & Trademark Office (USPTO) in patents cases as a patent agent (Registration No. 53,676). Member of the American Intellectual Property Law Association (AIPLA).
Pubs & Patents: Numerous technical pubs and presentations, inventor on 40 patents.
Work with ECS: Member for 42 years, ECS Fellow. Website: http://www.faradaytechnology.com/ https://orcid.org/0000-0002-3410-0267
Maria Inman, Research Director, Faraday Technology, Inc.
Patent Background: Inman serves as principal investigator on project development activities and manages the company’s pulse and pulse reverse research project portfolio.
Pubs & Patents: In addition to technical pubs and presentations, she is competent in patent drafting and patent drawing preparation. She is an inventor on seven patents.
Work with ECS: Member for 25 years. Serves ECS as a member of many committees.
Awards: ASTM member Website: http://www.faradaytechnology.com/ https://orcid.org/0000-0003-2560-8410
1. D. L. Parr IV, C. J. Jannuzzi, E. J. Taylor, and J. Leddy, The Electrochem Soc Interface 30 (2), 12 (2021).
2. C. Jannuzzi and E. J. Taylor, The Electrochem Soc Interface, 29 (4), 8 (2020).
3. https://patft.uspto.gov/ (accessed 8-19-2022).
4. https://patents.google.com/advanced (accessed 8-19-2022).
5. P. J. A. Kenis, The Electrochem Soc Interface 29 (3), 41 (2020).
6. https://www.nationalmedals.org/laureates/adam-heller (accessed 8-23-2022).
7. Brian A. Gregg and Adam Heller, “Enzyme Electrodes” US Patent No. 5,262,035 issued November 16, 1993.
8. E. J. Taylor and M. Inman, The Electrochem Soc Interface 28 (3), 37 (2019).
9. https://www.electrochem.org/heller (accessed 8-23-2022).
10. https://www.uspto.gov/learning-and-resources/ip-programsand-awards/national-medal-technology-and-innovation/ recipients/2008 (accessed 8-23-2022).
11. Esther S. Takeuchi and William C. Thiebolt, “Preparation of Silver Vanadium Oxide Cathodes Using AgO and V2O5 as Starting Materials” US Patent No. 5,389,472 issued February 5, 1995.
12. Esther S. Takeuchi et. al., “Alkali Metal having an Improved Cathode Activated with a Nonaqueous Electrolyte having a Carbonate Additive” US Patent No. 6,221,534 issued April 24, 2001.
13. https://www.invent.org/inductees/esther-sans-takeuchi (accessed 8-23-2022).
14. https://www.epo.org/news-events/events/european-inventor/ finalists/2018/sans-takeuchi.html (accessed 8-23-2022).
15. https://www.amacad.org/new-members-2021 (accessed 8-232022).
16. E. J. Taylor and M. Inman, The Electrochem Soc Interface 31 (1), 29 (2022)
17. https://www.electrochem.org/takeuchi/ (accessed 8-23-2022).
This feature page is intended to let ECS award winning students and post-docs write a primary-author perspective on their field, their work, and where they believe things are going. This month we highlight the work of Muhammad Mominur Rahman, the Battery Division 2021 Student Research Award winner.
Li-ion batteries are ubiquitous in our lives, as they are used in many modern technologies. Li-ion batteries have dominated the market because of their high energy density and stability. However, sustainability is a big issue with Li-ion batteries, because many of these batteries utilize expensive and/or toxic materials such as Li, Ni, and Co.1 Hence, researchers have been actively working on developing alternatives to Li-ion batteries. One facile way of developing beyond Li-ion batteries is to replace the Li with more affordable Na. However, with the lower redox potential of Na+ and its higher density relative to Li+, Na-ion batteries provide inferior energy density than their Li-ion counterparts. Furthermore, Na-ion batteries suffer from stability issues because of the complex structural and chemical evolutions during battery cycling. Still, Na-ion batteries attract much attention because of cost effectiveness as they require relatively cheap components such as Na, cathodes without expensive elements such as Co and Ni, and an Al current collector instead of Cu. These advantages mean Naion batteries can be an attractive choice for large-scale energy storage such as grid energy storage.2
Layered oxide materials are widely studied as cathode candidates for Na-ion batteries. They are made of alternating layers of transition metal oxide and alkali ions such as Na (Fig. 1a). Na-layered oxides show intriguing structural and compositional complexities.3 Unlike their Li-layered counterparts, Na-layered oxides can incorporate many different low-cost transition metals such as Cu and Fe4 and can be synthesized with different layered structures (e.g., O3, O2, P3, P2).3 This versatility opens multiple avenues to explore for stable cathode materials for Na-ion batteries. Here, three strategies to design stable Na-layered oxide cathodes are discussed. Taking advantage of their compositional and structural flexibility, stable Na-layered oxide cathodes can be synthesized by 1) utilizing doping chemistry to manipulate the structural and chemical properties, 2) tuning the elemental distribution to optimize the surface and bulk chemistry, and 3) exploring the elemental/compositional space to design stable Na-layered oxide cathodes.
Doping Na-layered oxides with trace elements enables controlled modification of the properties of these materials. Further tunability can be achieved when lattice site-specific doping is performed. For example, Mg can be doped either in the transition metal site or in the alkali-ion site of the layered oxide (Fig. 1a). If Mg is sitting on the transition metal layer, it can suppress local structural distortion,5 or when it is sitting on the alkali-ion layer, it can act as a pillar against interlayer gliding.6 Ti doping in layered oxides enables better retention of lattice oxygen during cycling due to strong Ti-O bonding.7 Doping with both Mg and Ti enables synergistic utilization of their stabilization effects on layered oxide cathodes. For example, doping NaxLiyMn1-yO2 with Mg and Ti enables suppression of Jahn-Teller distortion and better overall oxygen retention.8 Li in NaxLiyMn1-yO2 allows access to a large capacity from the material through oxygen redox because of the formation of unhybridized O2p orbitals. Mg-Ti dual doping in this material leads to suppressed Mn dissolution, prevention of Li loss, stabilization of the lattice structure, and reversible redox reaction during cycling (Fig. 1a). Overall, this leads to stable electrochemical performance of Mg-Ti dual doped NaxLiyMn1-yO2
Layered oxide cathodes contain multiple transition metals (TM) with different degrees of hybridization between TM3d and O2p orbitals. Cathode particles interact dynamically with the electrolyte during cycling. Incompatibility of electrolyte with cathode material can lead to electrolyte decomposition, transition metal dissolution, and cathode surface reconstruction.9,10 During electrochemical cycling, the extent of hybridization of TM3d-O2p orbitals undergoes dynamic evolution, which influences their interaction with the electrolyte. Hence, tuning the TM3d-O2p hybridization can be an
Fig. 1. Approaches to develop stable layered oxide cathodes for Na-ion batteries. (a) Crystal structure of Nalayered oxides. The structure of these materials is made of alternating layers of transition metal oxides and Na. Doping Na-layered oxides with trace elements such as Mg and Ti in NaxLi1-yMnyO2 leads to mitigated Jahn-Teller distortion and reversible structural evolution. Reproduced with permission from Ref. 8. (b) Tuning elemental distribution in individual cathode particles. 3D heterogeneous elemental distribution in Na0.9Cu0.2Fe0.28Mn0.52O2 Reproduced with permission from Ref. 11. (c) Exploring the composition space of Na-layered oxides. Elemental distribution in primary particles of Na0.75Co0.125Cu0.125Fe0.125Ni0.125Mn0.5O2. Reproduced with permission from Ref. 13.
effective way of influencing the cathode-electrolyte interaction. Conventionally, layered oxide cathodes are synthesized with a homogeneous distribution of the transition metals. However, our work has shown that the TM3d-O2p hybridization can be tuned by controlling the distribution of transition metals in each individual cathode particle.11 This approach can lead to an advantageous depth-dependent redox reaction in the cathode particles and thereby influence their interaction with the electrolyte. Taking advantage of these facts, Na0.9Cu0.2Fe0.28Mn0.52O2 with a heterogeneous 3D elemental distribution (Fig. 1b) provides stable electrochemical performance. Hence, our study shows that optimizing the distribution of transition metals in individual cathode particles is an effective way of tuning the electrochemical performance.
One remarkable advantage of a Na-layered oxide is that it can tolerate many different transition metals in its structure because of the large size difference between Na ions (1.02 Å) and TM ions (0.5–0.7 Å).12 Such flexibility opens opportunities to explore the compositional space of Na-layered oxides, which provides further
tuning knobs for performance optimization. This wide design space allows creation of Na-layered oxide cathodes using cheap and abundant transition metals such as Fe. Taking advantage of the vast compositional space of Na-layered oxides, we designed a Na0.75Co0.125Cu0.125Fe0.125Ni0.125Mn0.5O2 cathode material using the majority of the first row transition metals (Fig. 1c).13 Stable electrochemical performance is achieved with earth-abundant transition metals such as Cu and Fe. Further optimization can be accomplished by designing cathode materials where Cu and Fe are the majority of the transition metals and completely eliminating Co and Ni.
Na-ion batteries based on Na-layered oxide cathodes are a promising and cost-effective alternative to Li-ion batteries. Wide compositional variations enable utilization of cheap and abundant elements, which potentially makes them environmentally friendly. Structural and chemical complexities can be exploited to design stable layered oxide cathodes. Incorporating trace elements in Nalayered oxides enables tuning the physicochemical properties of these materials, which further enables performance optimization. Purposefully designing the elemental distribution of cathode particles influences the cathode-electrolyte interaction and takes advantage of
depth-dependent redox reactions to stabilize cathode performance. Future development of Na-layered oxide cathodes may combine multiple approaches to controlling elemental distribution along with structural/compositional space exploration to design stable cathode materials.
This work was supported by the National Science Foundation under contracts no. DMR 1832613 and CBET 1912885. The authors would further like to acknowledge Virginia Tech College of Science Lay Nam Chang Dean’s Discovery Fund for support. © The Electrochemical Society. DOI: 10.1149/2.F10224IF
Muhammad Mominur Rahman, Postdoctoral Research Associate, Brookhaven National Laboratory Education: PhD in Chemistry (Virginia Tech). Research Interests: As a graduate researcher, his work involved developing design principles and advanced characterizations of cathode materials for Na/Li-ion batteries. Currently, he is working on developing electrolytes and advanced characterizations of Li-S and Li metal batteries as part of the DOE Battery500 Consortium.
Awards: ECS Battery Division Student Research Award (2021), Graduate School Doctoral Assistantship Award (2019), and Chemistry Research Award (2020) from Virginia Tech.
Feng Lin, Associate Professor of Chemistry, with courtesy appointments in the Department of Materials Science & Engineering and Macromolecules Innovation Institute, Virginia Tech Education: Bachelor’s degree in Materials Science and Engineering (Tianjin University), MSc and PhD in Materials Science (Colorado School of Mines). Research Interests: His research team focuses on tailoring the electrochemical processes in batteries, electrocatalysis, and smart windows. The research goal is to understand and control how the local chemical and structural heterogeneity (e.g., doping chemistry, grain boundaries, dislocations, electrochemical interface) governs the redox reactions in inorganic solids at relevant length scales.
Work Experience: Established his independent research group at Virginia Tech in Fall 2016. Prior to Virginia Tech, worked at QuantumScape Corporation as a Senior Member of Technical Staff (2015–2016), Lawrence Berkeley National Laboratory as a Postdoctoral Fellow (2013–2015), and National Renewable Energy Laboratory as a Graduate Research Assistant (2010–2012).
Awards: ECS Battery Division Early Career Award, Energy Storage Materials Young Scientist Award, and National Science Foundation CAREER award.
Scott Cushing, Assistant Professor of Chemistry, Caltech Education: BS in Physics, emphasis in Material Science and Chemistry and PhD in Physics, under Nick Wu and Alan Bristow (West Virginia University).
Research Interests: With a multidisciplinary background spanning Chemistry, Materials Science, and Physics, his research focuses on the creation of new scientific instrumentation that can translate quantum phenomena to practical devices and applications. The Cushing lab is currently pioneering the use of attosecond x-ray, time-resolved TEM-EELS, and ultrafast beams of entangled photons for a range of microscopy and spectroscopy applications.
Work Experience: Past appointments include Dept. of Energy EERE Postdoctoral Fellow, Prof. Stephen Leone Group University of California, Berkeley with a Co-Appointment at Lawrence Berkeley National Laboratory. Currently Senior Research Advisor for Pacific Integrated (PI) Energy, San Diego, CA.
Pubs & Patents: >60 publications, 3 patents, h-index >30, cited ~8,000 times
Awards: 2022 Cottrell Scholar, 2022 Shirley Malcom Prize for Excellence in Mentoring, 2019–2021 Young Investigator awards for DOE, AFOSR, ACS, and Rose Hill Foundation.
Work with ECS: ETD Division: assist with organizing and chairing symposium. Member for >15 years. Website: cushinglab.caltech.edu https://orcid.org/0000-0003-3538-2259
1. Z. Yang et al., Adv Energy Mater, 12, 2200383 (2022).
2. R. Van Noorden, Nature, 507, 26–28 (2014).
3. N. Yabuuchi, K. Kubota, M. Dahbi, and S. Komaba, Chem Rev, 114, 11636–11682 (2014).
4. L. Mu et al., Adv Mater, 27, 6928–6933 (2015).
5. G. Singh et al., J Phys Chem Solids, 70, 1200–1206 (2009).
6. Q.-C. Wang et al., J Am Chem Soc, 141, 840–848 (2019)
7. J. D. Steiner et al., ACS Appl Mater Interfaces, 11, 37885–37891 (2019)
8. M. M. Rahman et al., ACS Energy Lett, 6, 2882–2890 (2021)
9. M. Gauthier et al., J Phys Chem Lett, 6, 4653–4672 (2015).
10. F. Lin et al., Nat Commun, 5, 3529 (2014).
11. M. M. Rahman et al., Energy Environ Sci, 11, 2496–2508 (2018).
12. Y. Sun, S. Guo, and H. Zhou, Energy Environ Sci, 12, 825–840 (2019).
13. M. M. Rahman et al., ACS Mater Lett, 1, 573–581 (2019).
As climate change intensifies, weather patterns across the globe become far less patterned and more unpredictable. Instances of extreme heat, excessive rain resulting in floods, and droughts that may cause or intensify wildfires have severely stressed centralized water treatment and distribution facilities and compromised access to fresh water for many. Such events include the recent water crisis in Jackson, MS, USA, in which thousands of residents have not had reliable access to clean water for weeks due to a plant pump failure.1 Severe flooding in Pakistan buried one-third of the country in water, destroying crops and eliminating access to clean water.2 Currently, Europe is experiencing its worst drought in over 500 years, exacerbating wildfires and stressing electricity generation.3 These are just a few examples that I have read about in the news—undoubtedly, there are more around the world and there will inevitably be more to come. Even without such events, fresh water is a scarce commodity. Only 3% of all the water on Earth is considered to be fresh, and a large portion of that is frozen in the polar ice caps. Fig. 1 shows two maps (1992 and 2018) of the renewable freshwater resources per capita in thousands of cubic meters by country, displaying a decreasing trend that is mostly the result of population increase.
Given the vast reserve of saltwater, desalination technologies that can separate the dissolved salts from the fresh water have become essential. This importance is evidenced by the increasing number of water desalination plants across the globe, estimated in 2012 to be ~ 15,000.4 Such plants capture seawater that then goes through a series of pretreatment steps to disinfect and remove large objects and microscopic particles that eventually results in a clear,
yet salty, water. Almost all the desalination plants in the world use a non-electrochemical method called reverse osmosis (RO) which comprises pressure-driven flow through porous membranes (~1nm pore size) that captures dissolved species such as ionic salts. Other common methods include multi-stage flash distillation (MSF), and mechanical vapor compression (MVC). These traditional desalination methods use either membrane or thermal-based technologies to separate solutes from saltwater and brackish water that have typical concentrations of 35,000 and 1,000 mg/L, respectively. However, they can be unnecessarily energy-intensive for lower salinity conditions.5
Electrochemical desalination is the removal of salts from water using electrochemical principles. Such methods include electrodialysis (ED), electro-deionization (EDI), and capacitive deionization (CDI). While many other electrochemical desalination methods exist, CDI seems to be the most researched approach. CDI, also referred to as electrosorption, was first conceptualized in 1960 and operates according to the principles of electrochemical double layer theory.6 When an electronic conductor is placed within an ionically conductive media, the excess surface charge of the metal imposes a redistribution of charged solutes such that the entirety of the interface may be related to the charge separation of a parallel plate capacitor. Under applied potential, the excess charge is increased, thereby more heavily attracting oppositely charged ions until the double layer region is fully occupied.
As shown in Fig. 2, polarization of two parallel electrodes with flowing saltwater in between will force the migration of anions and cations to the electrode surfaces, therefore separating the salt from the water. However, to do this efficiently, a suitable electrode material is required that can be polarized without degradative faradaic reactions while also maximizing the electrosorption capability. The electrodes should therefore have a large surface area with high wettability
Fig. 1. Renewable freshwater resources per capita: left 1992; right 2018 (Source: WorldData.org).
and be durable enough to withstand many charging cycles without performance degradation. Conventional materials often used as CDI electrodes include porous activated carbon and carbon aerogels. Research progress since the 1960s has advanced the technology by improvements to electrode material, developments in theoretical understanding, and alternative cell configurations. Advances in electrode material have been related to the idea that if the pore structure is appropriately designed to allow uniform access to the water within the pores while also allowing for ion mobility by ensuring the double layers of the pore walls do not overlap, the efficiency of CDI is greatly improved. Advanced electrode materials include hierarchal porosity systems such as carbon nanotubes, graphene, and flowing carbon slurries. Advances in the theoretical understanding of the electrosorption process have led to membrane capacitive deionization (MCDI) in which membranes placed near each electrode surface ensure that either cations or anions are specifically adsorbed, thereby improving the efficiency of the ion removal. Alternative geometries have also been tested, including the flow-through method in which the feedwater is pumped through porous carbon electrodes.6,7
The energy consumption of CDI depends greatly on the initial salinity of the feedwater and the desired level of salt removal, whereas the energy consumed during the RO process is only mildly dependent on these parameters. CDI therefore consumes less energy than RO for brackish water desalination but underperforms in comparison to RO for high salinity waters. For this reason, commercialization of CDI has been limited.6
Desalination fuel cells have the capability to simultaneously desalinate water and produce energy. A fuel cell comprises an anode and a cathode compartment separated by an ion-selective membrane or electrolyte. On the anode side, a catalytic material (e.g., platinum) is used to convert hydrogen gas to protons and electrons. The protons go through the membrane and enter the cathode compartment and react with oxygen to form water. The flow of electrons from anode to cathode is used to supply electricity.
A desalination fuel cell (example schematic shown in Fig. 3), combines electrochemical desalination principles and fuel-cell energy production to simultaneously produce electrons while extracting ions from saltwater. The cell description presented here is based on the 2020 JES article by Atlas et al.8 The cell comprises an anolyte (NaOH/NaCl) and a catholyte (HCl/NaCl) compartment that sandwiches a flowing water compartment and ion exchange membranes. A platinum catalyst located on the anolyte side facilitates the reaction of hydrogen gas and hydroxyl ions from the anolyte to form water. Electrons released in the process are transferred to the cathode side of the cell through an external connection. Oxygen supplied to the cathode reacts with protons in the catholyte and the supplied electrons from the anode to produce water. The flow of electrons drives an ionic current that is used to pull ions from the feedwater stream. Anions migrate from the feedwater stream through an anion-exchange membrane and into the anolyte, replacing the consumed hydroxyl ions. Cations from the feedwater migrate through a cation-exchange membrane and into the catholyte, replacing the consumed protons.
Although they are a relatively new concept, DFCs may have the potential to address both energy and water scarcity issues simultaneously and therefore merit further exploration and development. Atlas et al. provide a method to estimate the thermodynamic energy efficiency of DFCs considering redox chemistry as an input.
© The Electrochemical Society. DOI:10.1149/2.F11224IF
Christopher L. Alexander, Assistant Professor of Civil & Environmental Engineering, affiliate of the Chemical, Biological, and Materials Engineering Department and Susan and William Bracken Junior Faculty Fellow, University of South Florida
Education: BS and ME in Civil Engineering and PhD in Chemical Engineering (University of Florida).
Research Interests: Directs the corrosion research laboratory, which aspires to conquer corrosion while increasing the sustainability and resilience of critical infrastructure. Current projects include the corrosion performance of anodized aluminum formed with novel additives, susceptibility of prestressing steels placed within galvanized steel ducts to hydrogen embrittlement, reinforcement corrosion control for sustainable concrete formulations, and pitting corrosion models for steel in concrete.
Work Experience: Postdoctoral fellow at Sandia National Laboratories within the Materials Reliability Center where he studied atmospheric stress corrosion cracking as it relates to the aging and lifetime of nuclear waste interim storage containers.
Honors & Awards: McKnight Dissertation Fellowship (2016–2017), DOW BEST Symposium (2016), University of Michigan NextProf (2016).
Website: https://usfcorrosion.wixsite.com/mysite https://orcid.org/0000-0002-9978-1913
1. S. Neuman, “The crisis in Jackson shows how climate change is threatening water supplies.” NPR: Climate, September 7, 2022.
2. M. Thomas, “Disease warning as Pakistan flood death toll rises.” BBC News, September 3, 2022
3. “Europe’s drought the worst in 500 years – report.” BBC News, August 23, 2022.
4. B. Valdez Salas and M. Schorr Wiener, Desalin Water Treat, 42, 347 (2012).
5. P. M. Biesheuvel et al., J Membr Sci, 120221 (2022).
6. S. Porada et al., Prog Mat Sci, 58, 1388 (2013).
7. S. Ntakirutimana, W. Tan, M. A. Anderson, and Y. Wang, J Electrochem Soc, 167, 143501 (2020)
8. I. Atlas, S. Abu Khalla, and M. E. Suss, J Electrochem Soc, 167, 134517 (2020).
Lithium plating is a typical degradation process during fast charging of a lithiumion battery (LiB), and it occurs when the overpotential of the negative electrode (NE) is greater than its open-circuitpotential (OCP). However, the OCP value and NE potential are dependent on several factors (electrode surface properties, stateof-charge, temperature); hence, accurate and timely determination of these values is challenging. In this study, the authors propose the use of a simple electrochemical method to determine lithium plating threshold voltage operando for any given 2-electrode cell system. This method neglects the overpotential of the positive electrode; thus, the NE overpotential is equal to cell overvoltage once the cell opencircuit-voltage (OCV) is known. As a result, the lithium plating threshold voltage can be calculated by summing cell OCV and NE OCP, which are measured from a 3-electrode cell test at different states-of-charge. To compare the method’s accuracy, a fastcharge 2-electrode setup at the calculated threshold voltages was monitored. Under this method, there was no clear lithium plating for 2 types of cells (NMC622 and NMC111) at threshold voltages during >1C fast charging. It also implies that the plating can be deliberately controlled once the threshold voltages are known.
From: P. Münster, M. Winter, and P. Niehoff, J Electrochem Soc, 169, 070525 (2022)
The Underpotential Deposition of Lead and its Effect on Carbon Monoxide Adsorption on Pt(111) Electrode Underpotential deposition (UPD) occurs when a metal can electrodeposit more easily on an electrode substrate than on itself. A thin layer around a monolayer is formed at potentials more positive than the equilibrium potential. Modification of Pt by Pb UPD improves the catalytic oxidation of formic acid (HCOOH). A team from National Central University in Taiwan have reported a high resolution in situ STM study revealing how this Pb adlayer improves catalytic activity by lessening the wellknown poisoning effect of carbon monoxide (CO). When CO is formed as an adsorbed intermediate during oxidation of organic molecules, it binds tightly to Pt. In the presence of Pb, the researchers found that Pb and CO were coadsorbed in an adlattice and could displace one another depending on the conditions. They used measurements of the atomic structure of the adlattice to devise a hypothesis that Pb not only reduces the total amount of adsorbed CO, but also shifts CO binding to less favorable sites. This explains how Pb modification of the electrode
increases the oxidation of HCOOH by 20x—by reducing the interaction between CO and Pt.
From: J. W. Huang, S.-Y. Huang, and S. Yau, J Electrochem Soc, 169, 082505 (2022).
Several different desalination technologies are being developed to create potable water. One such class of technologies involves the use of electrochemical couples to produce clean electricity and clean water simultaneously. Researchers from Technion-Israel Institute of Technology utilized hydrogen/oxygen couple and their work on desalination fuel cells (DFC) is part of the JES Focus Issue on Electrochemical Separations and Sustainability. Building on their previous work, the research focused on additional membrane pairs as part of the DFC. The team developed a theoretical framework to estimate the thermodynamic energy efficiency of the DFC with multiple membrane pairs. With additional membrane pairs enabling the separation of anolyte/ catholyte from the desalination feed, the group was able to optimize the pH levels of anolyte and catholyte. They studied the impact of additional membrane pairs on generated power density and desalination. The work also included the identification of an optimum concentration of anolyte and catholyte and the right acid type to minimize activation losses. Finally, the researchers investigated the impact of saline water feed rate on the performance and the overall efficiency of the system. The researchers showed that additional membrane pairs would help improve DFC performance.
From: S. Abdalla, S. A. Khalla, and M. E. Suss, J Electrochem Soc, 169, 063508 (2022)
Detection of Marginally Discriminated Ions with Differential Solid Contact Ion-Selective Electrodes
Measuring ammonium concentration in the presence of one order of magnitude or higher potassium ion concentration is impossible with conventional ammonium ion selective electrodes because of the similarities between the two ions. To tackle this challenge, researchers from the Budapest University of Technology and Economics of Hungary first demonstrated a setup of a three-compartment cell with two identical reference electrodes in the two outer compartments separated by two ion-selective membranes (Anal Chem 2016, 88, 9850), only one of which contained the ammonium ionophore. Potassium interference to ammonium was significantly reduced by measuring the current between the two electrodes without applying any external power to the system. In this recent report, the authors
further improved the setup by replacing the two outer compartments and electrodes with two solid contact ion-selective electrodes coated with plasticized PVC membranes, only one of which contained the ammonium ionophore. By measuring the difference between the two electrodes amperometrically or potentiometrically, both digital simulation and experimental results showed significant response of a few µM ammonium in the presence of 0.1 mM potassium. The significantly reduced size of the sensor made it potentially applicable to droplet-size samples.
From: M. M. Kovács, M. Kis, and L. Höfler, J Electrochem Soc, 169, 087515 (2022)
Fossil fuels, including coal and peat, are commonly used as precursors to produce commercial activated carbon. However, with increasing costs of fossil fuels and concerns over their contribution to climate change, there has been a shift in focus toward more renewable resources as well as carbon-rich waste materials, including tires and plastics. It is estimated that ~1.5 billion waste tires are produced annually worldwide which are either dumped in landfills or incinerated, which has a detrimental effect on global climate. To address this issue, a group of researchers based in China has reported on the conversion of waste tires into activated carbon and its application as a supercapacitor electrode material. Waste tires were shredded and pyrolyzed before treatment with mixed acids followed by KOH activation. Electrodes containing activated carbon derived from waste tires demonstrated a relatively high charge storage capacity of 408 F g−1 at a specific current of 0.25 A g−1 in a KOH electrolyte. This work highlights the opportunities that are available for using carbon-rich waste as precursors for value-added carbon materials.
From: D. Zhang, Z. Ma, Y. Zhang, et al., J Solid State Sci Technol, 11, 061004 (2022)
Tech Highlights was prepared by David McNulty of University of Limerick, Zenghe Liu of Abbott Diabetes Care, Chao (Gilbert) Liu of Shell, Chock Karuppaiah of Vetri Labs, Joshua Gallaway of Northeastern University, and Donald Pile of EnPower, Inc. Each article highlighted here is available free online. Go to the online version of Tech Highlights in each issue of Interface, and click on the article summary to take you to the full-text version of the article.
Internet use and streaming soared during the pandemic. As people searched for meaningful online education sources, many turned to the ECS newsfeed and YouTube to find the best in science-themed online sources.
As the world searches for solutions to the climate crisis, inquiring minds want to know who the brightest minds in batteries are. Wouldn’t you know it: 8 of the top 10 are long-term ECS members!
Winter is coming, and not only in Game of Thrones! Car and cell phone batteries drop in capacity in freezing weather. Find out why, and how to keep from being left out in the cold!
The ECS Webinar Series showcases distinguished speakers and ECS community members presenting their latest research. Dr. Jeevarajan presented critical research on thermal runaway in Li-ion cells and batteries.
The focus was on critical factors in localized corrosion in this issue honoring Gerald Frankel, long-term ECS member and leading researcher in the area. ECS News keeps readers on top of publishing opportunities.
The science-minded wanting to make their mark at post-pandemic Halloween events turned to this and to another top-10 evergreen post for costume ideas celebrating their science idols and scientific concepts.
ECS members chose 15 new ECS Fellows in 2022 based on their scientific achievements, leadership, and active participation in the Society. Learn about these leading lights of electrochemistry.
People read ECS News to learn something new and to stay on top of news and trends in their industry. They turned to ECS News for trusted advice on where to find the best in science and technology.
perish, right?
At 100 years old, amazing Nobel laureate and long-term ECS member John Goodenough has co-developed a rapidcharging, nonflammable glass battery. ECS News is the community’s portal to noteworthy news like this.
Seventy-five years ago, on December 16, 1947, the transistor was invented by William Shockley, John Bardeen, and Walter Brattain at Bell Telephone Laboratories. This was a big milestone in human history as it was the origin of the field of micro/ nano electronics, which is now resulting in a super-intelligent society. The technological revolution that followed the invention of the transistor is astounding and has moved mankind forward in countless ways. This paper describes the process of the technological development behind the invention of the transistor, and discusses the meaning and impact of its invention on human history.
Innovations in communication and information processing have been a driving force in the evolution of human culture and society, and this advancement can be divided into three waves of seminal achievements. The first wave of innovation was the invention of language long ago in human prehistory. Through language, we could communicate and think deeply and widely. The second wave was the invention of characters/letters 5000 to 10,000 years ago. Through characters/letters, we could communicate accurately with people who lived in faraway places and over many generations in time scale. The third wave was the invention of electric information, which started about 175 years ago. With electric information, we could communicate over long distances instantaneously, and make machines that can process huge amounts of information in a short time.
There have also been three stages of technological leaps during the electric information technology wave. The first leap was the instantaneous and long-distance transportation of information (and energy) through electric wires This was the beginning of electrical engineering. Information transportation was accomplished
by the “practical electric telegraph” technology invented by Samuel Morse and others in the late 1830s to the middle of the 1840s.1 Through the electric telegraph, we could communicate long-distance instantaneously in long sentences with accuracy. By the 1870s, the world became connected by the electric telegraph through submarine cables. Because of the decay of the electric signal on the long electric wire, amplification of the signal in the middle of the wire was necessary. This amplification was accomplished by a relay which was invented in the middle of the 1840s (shown in Fig. 1). The relay is a kind of three-terminal mechanical-switching device, operating by the electromagnetic force controlled by the input current flowing in the magnetic coil. The relay was the first amplifier for an electric signal, but it was only a digital amplifier operating at a very low frequency because of the mechanical switching operation.
The second leap was the invention of triode vacuum tubes in 1906 by Lee de Forest.2 This was the first three-terminal electron device. The thermo-electron beam in vacuum emitted from the heated cathode to the anode was modulated by the grid potential that was inserted in the beam, as shown in Fig. 2. The triode vacuum tube was the first analog and digital amplifier which could operate at a very high frequency, almost near the GHz range. The triode can be used to manipulate the movement of electrons by the grid bias as we wish, and this was the beginning of electronics or electronic engineering Through electronics, we were able to treat information electrically, and not only perform telecommunication by wireless radio/TV broadcast, but also information/data processing, automatic machinecontrol, and computer operations. Later artificial intelligence (AI) was also realized.
The third leap was the invention of the transistor (or the solidstate amplifier) in 1947 and the resulting micro/nano electronics with the invention of photolithography 3, 4 Micro/nano electronics or continuous miniaturization of electronic devices was a totally new concept in electronics. As shown in Fig. 3, the reduction of the dimension of electronic devices by 105 times over the past 115 years (since the invention of triode vacuum tubes), has brought us tremendous performance increases of billions to trillion times in operation speed, power consumption, volume, weight, and cost. The invention of the transistor in 1947 was the origin of the device miniaturization that has made possible the revolution we are now experiencing: a super-intelligent society with nanoelectronics.
By the mid-1940s, electronics— which started with the invention of the triode vacuum tube in 1906— had brought great benefits to human society. However, the relatively long electron transit time—due to the difficulty in decreasing the vacuumtube size—made it impossible to operate at the microwave frequency range. Also, the need to heat the cathode electrode above 1000℃ for
emitting the electrons hampered power saving and heat insulation of the systems. Many people dreamed of replacing the triode vacuum tube with some kind of a three-terminal solid-state amplifier to solve these problems
Control of an electron current in a vacuum using the grid potential was easy. However, control of the electron current in a solidstate conductor (or metal) was almost impossible because of the huge density of the free carriers, as shown in Fig. 4. On the other hand, in the case of insulators, there is no current flow at all. Thus, semiconductors that have lower electron density were thought to be candidates.
The use of semiconductors had already started for commercial point-contact rectifiers (or crystal detectors) from the beginning of the 20th century for demodulating wireless radio waves to the audio signal using their nonlinear characteristics.5–7 Semiconductor rectifiers were used popularly for low-priced radio receivers and very high-frequency microwave receivers for which triode vacuum tubes were not suitable. However, the rectifiers had very limited ability to treat the signals (e.g., they had no signal amplification ability), which was a result of the nature of two-terminal devices. Thus, a demand for three-terminal semiconductor rectifiers was created
A seemingly easy idea for creation of three-terminal solid-state amplifiers could be to control the electron (or hole) current by
the potential of the grid electrode inserted in the depletion region where there are much fewer free carriers, as shown in Fig. 5 (a) and (b). The concept of the depletion region became recognized through the rectifying theories developed by Walter Schottky,8, 9 Nevil F. Mott,10 and Boris Davydov in 1938.11 These types of devices were proposed by a German company, AGE, in 192912 and W. Shockley in 1939,13 but no successful results were reported, except for a case by Rudolf Hilsch and Robert Wichard Pohl of Göttingen Universität in 1938,14 as shown in Fig. 6.
Hilsch and Pohl used a KBr crystal. KBr is an ionic crystal with a wide bandgap (7 ~ 8 eV) and is an insulator rather than a semiconductor. Electrons were injected from the point-contact cathode electrode into the KBr crystal (2 x 5 mm cross-section and 10 mm length) at a high temperature of 490℃. There were almost no carriers other than the injected electrons and the ions of KBr, the latter of which amount to about 3% of the injected electrons at 490℃. The injected current was modulated by the potential of a Pt grid electrode inserted into KBr at 2 mm distance from the cathode. The Pt-to-KBr contact was a Schottky contact and there was a very small leakage of current at the grid voltage (VG ) of 0 ~ 13 V. The maximum current amplitude (IA/IG) of 20 was observed by the experiment, where IA is the anode current. The mobility of electrons in KBr was very low and the transit time of electrons in the KBr crystal of 10 mm was 10 seconds. Thus, the operating frequency was estimated to be only sub-Hz.
This result was the first experimental record of a solidstate amplifier and a type of MESFET (Metal-Semiconductor Field Effect Transistor), although the performance was not satisfactory at all, basically because KBr is an insulator. They also investigated the possibility of a Cu-Cu2O (semiconductor) rectifier in order to operate at a higher frequency at room temperature, but the depletion layer width was found to be 0.1 μm, and they reached the conclusion that it was not possible to make the solidstate amplifier by inserting the grid into the semiconductor
Another approach for the semiconductor amplifier was to use the ultra-thin semiconductor film and control the lateral current in the film by a vertical electric field. The
remotely by the gate electrode voltage with capacitive coupling, as shown in Fig. 5 (c). This is also a kind of FET, although the name of the transistor did not exist until 1948.
Even before the ideas of the grid-inserted structures, the idea of ultra-thin semiconductor film MESFET in 192515 and MISFET (Metal Insulator Semiconductor FET)/MOSFET (Metal Oxide Semiconductor FET)16 in 1928 were filed as patents by Julius E. Lilienfeld. Figure 7 shows the case of his MOSFET. There are no records of experimental results of Lilienfeld’s MESFET and MOSFET. In the early 1960s, J. B. Johnson fabricated Lilienfeld’s MOSFET, but observed no amplification or even modulation.17 The reason was probably the very low mobility of holes in Cu2S (~ 1 cm2V-1sec-1), and the effect of surface states on the surface of the film. Later, the ideas of MISFETs/MOSFETs were proposed by several people—Oskar Heil in 1934,18 Heinrich Welker in 1945,19 and even by W. Shockley in 194513 and others. But none of them showed successful amplification results until 1960, because of immature semiconductor quality and poor understanding of and technology to control the semiconductor surface.
Shockley’s group at Bell Laboratories started the development of the solid-state amplifier after World War II and continued to research surface states for the purpose of developing FETs. Shockley’s first attempted MISFET with an air gap as a gate insulator in 194513 did
not work because the surface state charges shielded the electric field from the gate.20 Based on Walter Brattain’s and others’ research on surface state charge compensation,21,22 John Bardeen proposed to modulate the injected surface current—from the point-contact source electrode—by the gate electrode immersed in a drop of distilled water on November 21, 194723–25 as shown in Fig. 8.26, 27 The highresistivity distilled water was used as a gate insulator and the water molecule dipoles at the semiconductor interface enhanced the local electric field to control the channel current. 21, 22, 28, 29 This is a kind of a vertical MISFET using distilled water as the gate insulator. The dielectric constant of the water is about 80, and the electric flux density in the water is about 80 times larger than that of air. Current and power gains were observed for the first time at Bell Laboratories, but there was no voltage gain.27
Eventually the distilled water was replaced by glycol borate in order to solve the problem of water evaporation and disappearance during the measurements.21, 22, 24, 25 On December 8, 1947, Bardeen proposed using a Ge semiconductor used for “high back voltage” rectifiers with high breakdown voltage, which was supposed to have low defect and impurity densities.24, 25 They obtained a power gain of 330 and a voltage gain of 2,24, 25 but the maximum operation frequency was only 8 Hz21, 22 because of low reaction time of the
gate insulator—instead of the glycol borate—in order to improve the frequency dependence. Ge oxides are dielectrics and the dipole effects were expected.
Figure 9 shows the planned/executed sample structures (prepared by Robert B. Gibney) and circuit configurations. It happened that the Ge oxides disappeared through the water treatment to wash off the glycol borate before the gate electrode metal deposition. 21, 22
At the initial measurement, Brattain ruined one of the gate electrodes by accidentally shorting it with the point-contact source electrode when he placed the point-contact electrode inside of the ring-shaped gate electrode.25 Then, he put the point-contact electrode near the peripheral of the gate electrode and obtained a voltage amplification effect when he changed the polarity of the DC bias of the “gate” electrode (or actually, it was named “emitter” immediately after) at 1 kHz on December 12, 1947, but still no power amplification was observed.25,27
Bardeen proposed to put the source and gate electrode within a distance of 50 µm in order to increase the amplification27 and Brattain made the structure using gold foils as the electrodes separated by 20 µm, 21, 22, 31, 32 as shown in Fig. 10. With this structure, on December 16, 1947, both the voltage and the power gains were confirmed at 1 kHz.31,32 Under the condition of grid bias = +1 V, plate bias = -15 V, and output load resistance = 10 kΩ, a voltage gain of 15 and a power gain of 1.3 were measured.31 AC input and output voltages were 0.6 and 10 V, respectively, and input
and output AC powers were 7.5 and 10 mW, respectively.31 This represents the discovery of the point-contact transistor effect and the invention of the point-contact transistor.
Although gold foils were used as the electrodes in the experiment, two metal tungsten needles were used as the emitter and collector electrodes later in commercial devices. The patent32 describes a thin p-type Ge layer at the surface of the n-type Ge substrate. Bardeen initially thought that the surface p-type layer was necessary for the injected hole current to reach the collector effectively. However, it was soon found, on February 13, 1948,31 by John N. Shive, that the thin p-type surface layer is not necessary for the transistor action because the minority carriers (holes in this case) can migrate in the n-Ge base for a sufficiently long distance 33 The name “transistor” was proposed by John R. Pierce as an “abbreviated combination of the words ‘transconductance’ or ‘transfer’ and ‘varistor,’” and determined by a ballot among several candidates on May 28, 1948.34
In December 1947, Brattain and Bardeen happened to discover the point-contact transistor operation during experimental work aimed at realizing FET operation. This device is not the FET that modulates the surface lateral current by the gate vertical field. It took some time to understand the mechanism of the amplification (or transistor action)
Fig. 9. The first point-contact transistor operated at 1 kHz with power gain on December 12, 1947.25, 27 They planned to fabricate a MOSFET with anodic Ge oxide as the gate insulator, but the Ge oxide happened to be washed away by the water treatment. Electrode names “source,” “gate,” “drain,” “emitter,” “base,” and “collector” were labeled by the authors to aid in understanding.
of the point-contact transistor.32,35 The point-contact transistor is a device that modulates the vertical electron current injected from the collector metal by the hole current injected from the emitter metal, as shown in Fig. 11.35, 36 The injected hole current finally flows into the collector metal (the most negative electrode), resulting in the elevation of the local potential and carrier concentrations in the Ge near the collector contact. The collector contact is the Schottky reverse-mode-junction that is sensitive to the Ge potential and carrier concentration,9, 36 resulting in the enhancement of the electron injection from the collector metal in this case. This scheme is the mechanism of the collector electron current amplification by the emitter hole current injection.
Shockley felt frustrated by the fact that he was not an inventor of the transistor.13 He worked to “invent new semiconductor amplifier principles” from December 31, 1947, and “completed conception of junction transistor” on January 23, 1948, 13, 37 as shown in Fig. 12. The junction transistor is a device that modulates the n-Ge emitter electron-current to the n-Ge collector via the p-Ge base due to p-Ge base potential applied through the base electrode
Elevation of the p-Ge potential increases the emitter electron current at the forward-biased emitter-base pn junction. Because collector potential is set to the most positive potential, the injected emitter electron current into the p-Ge base flows finally into the n-collector. This process is the mechanism of the bipolar junction transistor operation. The ratio of the base hole current to the emitter electron current at the pn junction is related to the ratio of emitter donor concentration to the base accepter concentration that is typically set to 100. Thus, there is emitter current amplification by the base current.
Because of the mechanical instability of the point-contact transistor, it disappeared from the market by the middle of the 1950s, and junction transistors started to be widely used for many intelligent applications, including the NASA Apollo Project, and eventually replaced vacuum tubes by the middle of the 1960s. During this period, various key semiconductor process technologies were developed, resulting in the invention and successful operation of the MOSFET in 1960, 39, 40 as shown in Fig. 13. One of the
the MOSFET was high-temperature thermally grown gate SiO2 in order to stabilize the semiconductor surface with small surface state concentration.40, 41 MOSFETs are now the mainstream devices for the recent nano-CMOS integrated circuits. In the near future, a super-intelligent society will realize new technologies such as AI constructed
nanoelectronics, which originated with the transistor’s invention 75 years ago.
The process through which the transistor was developed was described. The first transistor, the point-contact transistor, happened to be developed unintentionally in the course of FET development in December 1947. The operation mechanism (i.e., the bipolar transistor action) of the point-contact transistor was beyond the inventors’ original contemplation. In January 1948, immediately after the invention of the point-contact transistor, the idea of the bipolar junction transistor was inspired by the invention of the point-contact transistor. Because of the mechanical instability of the point contact, the bipolar junction transistor replaced the point-contact transistor in the market in the early 1950s. Bipolar junction transistors were the main solid-state devices in the 1950s and 1960s and contributed
Fig. 13. The first successful operation of MOSFETs by Kahng and Atalla in 1960.38, 39 One of the key technologies for the realization of the MOSFET was high-temperature thermally grown gate SiO2 to stabilize the semiconductor surface with small surface state concentration.40 Kahng and Attala used steam oxidation at 650℃ under high pressure of 40 ~ 50 atm.41
greatly to the electronic society in that period. During that period, various semiconductor process technologies were developed, resulting in the invention and successful operation of the MOSFET in 1960, which is now the mainstream device for the recent nanoCMOS integrated circuits.
The authors are grateful for the kind support of the library of Tokyo Institute of Technology for obtaining old published documents.
© The Electrochemical Society. DOI: 10.1149/2.F13224IF
Hiroshi Iwai, Professor Emeritus of Tokyo Institute of Technology, Vice Dean and Distinguished Chair Professor, National Yang Ming Chiao Tung University Education: BE and Doctorate in Engineering (University of Tokyo). Research Interests: Semiconductor device engineering.
Work Experience: Worked at Toshiba, Tokyo Institute of Technology, and National Yang Ming Chiao Tung University for 50 years. Prof. Iwai is a semiconductor device engineer who contributed to the development of integrated circuits technologies and products. In particular, he has contributed to the miniaturization of LSIs since the 8 μm PMOS.
Honors & Awards: Include ECS Fellow and Awarded Life Member. Recipient of the ECS Gordon E. Moore Medal and the ECS Thomas Callinan Award.
Work with ECS: Member, ECS EPD Executive Committee and organized many ECS Symposia for the past 25 years. Served as Chair of the ECS Japan Section.
Durga Misra, Professor and Department Chair, Electrical and Computer Engineering, New Jersey Institute of Technology
Education: MS and PhD in Electrical Engineering (University of Waterloo, Canada).
Research Interests: Has been working on nanoscale semiconductor devices, especially on gate dielectrics and particularly in high-k gate stack engineering for nanoscale devices and
device reliability for the last 35 years. His current research interest is in the design and processing of low power devices using high-k dielectrics for in-memory computing.
Honors & Awards: Include Fellow of ECS and Awarded Life Member. Recipient of the ECS Thomas Callinan Award and the Electronic and Photonic Division Award.
Work with ECS: He has been the Chair of Dielectric Science and Technology Division of ECS and has been organizing technical symposia at ECS for the last 30 years.
1. S. F. B. Morse, “Improvement in the Mode of Communication by Signals by the Application of Electro-Magnetism,” US Patent 1647, filed April 7, 1938, issued June 20, 1840; Reissue No. 79, January 15, 1846; Reissue No. 117, dated June 13, 1847.
2. L. de Forest, “Space Telegraphy,” U.S. Patent 879,532, filed January 29, 1907.
3. J. W. Lathrop and J. R. Nall, “Semiconductor Construction,” US Patent 2,890,395, filed October 31, 1957.
4. J. Andrus, “Fabrication of Semiconductor Devices,” US Patent 3,122,817, filed August 15, 1957.
5. J. C. Bose, “Detector for Electrical Disturbances,” US Patent 755,840, filed September 30, 1901.
6. G. W. Pickard, “Means for Receiving Intelligence Communicated by Electric Waves,” US Patent 836,531, filed August 30, 1906.
7. H. H. C. Dunwoody, “Wireless telegraphy system,” US Patent 837,616, filed March 23, 1906.
8. W. Schottky, Naturwissenschaften, 26, 843 (1938).
9. W. Schottky and E. Spenke, Wiss. Veroff. Siemens-Werken, 18, 225 (1939).
10. N. F. Mott, Mathematical Proceedings of the Cambridge Philosophical Society 34, 4, 568 (1938).
11. B. Davydov, Comptes Rendns (Doklady) de l’Académie des Sciences de I’URSS, 20, 4, 279 (1938).
12. Allgemeine Elektrizitäts-Gesellschaft, “Kontaktgleichrichter mit zwei durch eine Sperrschicht getrennten Metallelektroden.,” (Contact rectifier with two metal electrodes separated by a barrier layer), Ӧsterreichisches Patentamt, AT13102, filed July 11, 1930, priority July 11, 1929.
13. W. Shockley, IEEE Trans Electron Devices, 23, 7, 597 (1976).
14. R. Hilseh and R. W. Pohl, Zeitschrift für Physik, 111, 399, (1938).
15. J. E. Lilienfeld, “Method and apparatus for controlling electric current,” US Patent 1745175A, filed October 8, 1926. (Priority CA272437TA·1925-10-22).
16. J. E. Lilienfeld, “Device for controlling electric current,” US Patent 1900018, filed March 28, 1928.
17. J. B. Johnson, Physics Today, 17, 5, 60 (1964)
18. O. Heil, “Improvements in or relating to Electrical Amplifiers and other Control Arrangements and Devices,” British Patent GB439457 (A), Convention date March 2, 1934, Application date March 4, 1935, Complete specification accepted December 6, 1935.
19. H. J. Welker, “Halbleiteranordnung zur kapazitiven Steuerung von Strömen in einem Halbleiterkristall,” (Semiconductor arrangement for capacitive control of currents in a semiconductor crystal) Deutshe Patentamt DE 980084, filed June 4, 1945, priority April 7, 1945.
20. J. Bardeen, Phys Rev, 71, 10, 717 (1947).
21. W. H. Brattain, The Physics Teacher, 6, 109 (1968).
22. W. H. Brattain, Adventures in Experimental Physics, 5, 1 (1976).
23. W. S. Gorton, Proc IEEE, 86, 50 (1998)
24. L. Hoddeson, Hist Stud Phys Sci, 12, 41 (1981).
25. M. Riordan and L. Hoddeson, Crystal Fire: The Invention of the Transistor and the Birth of the Information Age, W. W. Norton & Company (1997).
26. J. Bardeen, “Three-Electrode Circuit Element Utilizing Semiconductive Materials,” US Patent 2524033, filed on February 26, 1948.
27. J. Bardeen, Science, 126, 3264, 105 (1957).
28. P. Brauer, Annalen der Physik, Series 1, 24, 609 (1936).
29. H. J. Welker, Ann Rev Mat Res, 9, 1 (1979).
30. R. B. Gibney, “Electrolytic surface treatment of germanium,” US Patent 2560792, filed on February 26, 1948.
31. J. A. Hornbeck, in A History of Engineering and Science in the Bell System, Electronics Technologies 1925 – 1975, F. M. Smith, Editor, p. 1, AT & T Bell Laboratories, International Standard Book, (1985).
32. J. Bardeen and W. H. Brattain, “Three-electrode circuit element utilizing semiconductive materials,” US Patent 2524034, filed on June 17, 1948 (a continuation-in-part of application Serial No. 11,165, filed February 26, 1948).
33. J. N. Shive, “Semiconductor amplifier,” US Patent 2,691,750, filed Aug. l4, 1948.
34. J. R. Pierce, Proc IEEE, 86, 37 (1998).
35. J. Bardeen and W. H. Brattain, Phys. Rev., 75, 1208 (1949).
36. W. Shockley, Electrons and Holes in Semiconductors: With Applications to Transistor Electronics, van Nostrand (1950).
37. W. Shockley, “Circuit Elements Utilizing Semiconductor Material,” US Patent, 2,569,347, filed June 26, 1948.
38. D. Kahng, “Electric Field Controlled Semiconductor Device,” US Patent 3,102,230, filed May 31, 1960.
39. M. M. Attala,” Semiconductor Triode,” US Patent 3,056,888, filed August 17, 1960.
40. M. M. Atala, and E. Tannenbaum, “Fabrication of Semiconductor Devices having Stable Surface Characteristics,” US patent 2,899,344, filed April 30, 1958.
41. J. R. Ligenza, “Method of Treating Silicon,” US patent 2930722, filed February 3, 1959.
This Interface article is based on presentations at the 242nd ECS Meeting in Atlanta, in the centennial symposium “E04 - 100 Years of the Electrodeposition Division: Past, Present, and Future,” given on Wednesday October 12, 2022.
This presentation celebrates the Centennial meeting of the Electrodeposition Division, which was founded in 1922 to “advance the theory and practice of Electrodeposition.” Brief retrospectives such as this are by nature incomplete and idiosyncratic. We apologize in advance for the omissions that characterize individual recollections of past events. Today, in our 60 minutes to comment on 100 years, we wish to offer our perspectives as though they are Pieces of a Frame. There are many others here, at the centennial symposium, who could contribute additional pieces and perspectives.
Our heroes of two centuries ago are the pillars of electrochemistry whose curiosity established its early scientific foundations: A. Volta, C. Coulomb, A. Ampère, G. Ohm, H. Davy, and M. Faraday, among others. Standing on their shoulders came the scientists who established methods of measuring and tabulating fundamental electrochemical phenomena: W. Nernst, L. Hittdorf, A. Fick, F. Kohlrausch, J. W. Gibbs.
Then came the DC electric generator, which changed everything. It created opportunities for large-scale industrial electrochemical operations, including electroplating, electrowinning, and electrorefining, as well as the manufacture of aluminum, copper, chlorine/caustic, and other essential industrial commodities. Their early development and commercial use took place before the recognition of many fundamental scientific and engineering principles. As a result, these industries came to be characterized by slow evolutionary change based on experience and informed intuitive insight.
Interest in electrodeposition was clearly evident from the inaugural meeting of the Society in 1902. Scanning those papers and published comments, it is abundantly clear that The Electrochemical Society played a key role in bringing together industrial practitioners and research scientists to discuss fundamental principles and their use to guide practical improvements in products and processes … a good recipe even today.
In 1913, a groundbreaking symposium on electrodeposition was carried out in close collaboration with the American Electroplaters Society. It served to apply systematic academic effort to the art of the plater, and thus to promote cooperation between science and technology. The published proceedings were recorded in the 1913 volume of the Transactions. These could arguably be called the first edition of Modern Electroplating, the pioneering publication that first appeared in 1940 and is now in its fifth edition.
These and other successful endeavors led to the formation of The Electrodeposition Division at the 41st Meeting of the Electrochemistry Society in the Spring of 1922. During the subsequent several decades, many scientific papers appeared on such topics as overvoltage, addition agents, and the stress, structure, composition, and properties of the electrodeposit. During this period, scientific advances were made in development of experimental tools (e.g., polarography), measurement of data (e.g., thermodynamic) and theory (e.g., non-ideal electrolytic solutions). Meanwhile, however, the commercial products of electrodeposition technology grew rapidly and more-or-less independently of electrochemical science. As a consequence, electrodeposition technology remained largely empirical. One of the obstacles to progress recognized at that time
was the lack of people with both sufficient knowledge of the physical chemistry of solutions, and sufficient interest in electrodeposition to bridge the gap between science and industrial practice. I will leave you to decide whether history is repeating itself.
During those early years, the means for achieving quality control were established through extensive development of specifications carried out through cooperation with the American Electroplaters Society (ASTM), and the US National Bureau of Standards (NBS). These activities promoted the association of people with different but related interests. Their committee activities stimulated advances that could be made only by concerted effort. These organizations had scientific and technological interests that were for the most part complementary and not competitive. This opened the door to discussions around intellectual property which continue today.
The principal applications for electrodeposition at that time (1920s–1940s) were the electrowinning of Zn; the electrorefining of Cu, Pb, and Ni; the electroplating of bright Ni and Cr, driven by the automobile industry; electroforming for high precision production, such as electrotyping and phonograph records; and various applications in electroanalysis
Beginning in the mid-twentieth century, there was a tremendous expansion in the electrochemical and electroprocess industries that moved from the laboratory bench to widespread commercial use. These new products and processes, along with enormous increases in the size of cells and new sources of raw materials, made necessary an almost complete rearrangement of the “rules of thumb” that dominated the technology of the day. The sense was emerging that progress based on empirical experimentation was rapidly coming to a close, and that further significant advances could be made only when the fundamentals of the plating processes were more completely understood.
Significant experimental advances made at that time led to recognition of the transport processes associated with the potential field between electrodes, and with the concentration variations near electrode surfaces. Indeed, the distribution of electrodeposited metal on a cathode provided the starting point for several decades of investigations on current and potential distribution phenomena. These concepts expanded rapidly to a wide variety of electrochemical systems that were well beyond electrodeposition. In most of these cases, it was the close connection with quality control for technological processes that elevated the importance of the topic. It is worth pointing out that it was the practical interest in the current and potential distribution phenomena that sparked the enormous growth of underlying mathematical tools.
The technologies that drove the field included porous battery and fuel cell electrodes, corrosion protection, inorganic and organic synthesis, and metal refining, among many others. Especially significant was the general recognition that most large-scale electrochemical processes are driven to their transport limit and thus constrained either by ohmic resistance or convective diffusion. For this reason, the electrochemical engineering research literature of the past 75 years has focused strongly on understanding ohmic and mass transport processes, including the effect of hydrodynamic flow.
In complex systems that include multiple phenomena, the question arises, “Which is the most important?” Many engineering fields use dimensionless ratios to decide between the relative importance of two concurrent phenomena. Many of the transport-centric electrochemical engineering analyses of that time can trace their roots to those non-
electrochemical treatments in fields such as heat conduction, diffusion, and fluid convection processes. Their first use in electrochemical systems was reported independently by C. Wagner and T. P. Hoar (called the Wagner Number, or “polarization parameter”). The value indicated the relative importance of the transport rate through the volume versus the reaction rate at the surface. Progress was initially made with use of simplified limiting cases that cleaved a complex system into smaller pieces that were more easily addressed. These came to be code-named primary, secondary, and tertiary current distributions according to which phenomena dominated the system.
While many contributed, the efforts of Wagner and V. G. Levich influenced scientists and engineers because their work established a new level of mathematical rigor. Significantly, their approach was re-usable by others. It was simple. It began with a dimensionless number. Several researchers established electrochemical science and engineering training centers, including M. Fleischmann, A. N. Frumkin, H. Gerischer, F. Hine, N. Ibl, C. W. Tobias, E. B. Yeager, and others. They and their colleagues developed important experimental and theoretical methods of study. The number of individuals who were products of these and other pioneering efforts quickly grows too numerous to mention by name.
Electrodeposition applications drove many of the foregoing science and engineering studies. But, significantly, there were many systems outside of the electrochemical field that made an impact on the electrodeposition field. The value of reading the literature to identify developments seemingly outside of one’s specialty became important. This trend continues today as the electrodeposition field expands to the molecular scale.
During the 1960s, digital computers came into use for calculating current distribution. During this period, experimental studies benefitted from development of model systems, which made possible studies under well-characterized transport conditions. Two of the more popular model electrolytes were the basic ferri/ferro-cyanide, and the acid copper sulfate system. These enabled development of the limiting current method for measuring the electrochemical reaction rate under mass transfer control.
Experimental advances in the 1960s and 1970s saw introduction of the potentiostatic power supply that enabled a wide range of experiments for probing dynamic behavior. Control of other important variables was accomplished by purification of the electrolyte, and the fabrication of well-defined electrode materials, including use of single crystals. In addition, powerful instrumental techniques came from physics-based investigations of surfaces under high vacuum and ultra-clean surface preparations. Surface spectroscopies and scanning methods eventually reached the atomic scale. Each of these developments fueled, directly or indirectly, the continued expansion of electrodeposition science. Thus began the on-ramp to electrochemical research at the molecular scale, which continues to this day.
Meanwhile, mathematical modeling advanced from algebra to differential equations. A particularly significant theoretical
breakthrough was achieved by coupling laminar boundary layer transport at an electrode with the potential field in the adjacent volume. The use of this technique for treating the rotating disk electrode was a particularly significant development that energized experimental methods for several decades to come.
During the 1970s and 1980s, the introduction of new materials (DSA, Nafion, Teflon) revolutionized electrodeposition science and engineering at virtually every step between the lab and the plant floor. At the same time, electrodeposition technology saw significant new demands arising from the changed availability of energy, feedstock, and capital as well as increased attention to waste treatment.
In the 1980s, microelectronic applications began to energize the electrodeposition field. For example, the fabrication of copper multilayer printed wiring boards was carried out with quality control of crystal structure and online monitors to achieve uniform current distribution over the large panels. As these and other applications were perfected, the microelectronics industry began to build confidence in electrodeposition process steps.
The invention and electrochemical fabrication of thin film magnetic recording heads provided dramatic densification of disk drive data storage density and reduced costs. These advances made possible the extraordinary growth of low-cost data storage. Also during this period, fundamental studies on single crystal electrodes using surface scanning microscopies and spectroscopies provided spectacular new capabilities for investigation at time scales, molecular specificity, and spatial resolution that were orders of magnitude superior to those of only a decade earlier. In addition, during this period, increases in digital computing power enabled computational methods that have become steadily more sophisticated and more user-friendly, eventually moving from the realm of programming specialists to commercial software used in undergraduate engineering programs. Each of these scientific and engineering developments fueled, directly or indirectly, the continued expansion of electrodeposition technology.
By the 1990s, the revolutionary impact of electrodeposition processes on the fabrication of transistors expanded rapidly. The first such production process implemented on Si wafer product was solder electrodeposition used for joining silicon chips to packages. This process, which is now the industry gold standard, demonstrated for the first time that electrochemical processing was feasible in a semiconductor-manufacturing environment. Electrodeposition of copper wire interconnections between transistors on chips was arguably the most revolutionary change in material (from Al to Cu) in the semiconductor industry in three decades. The success of these two pioneering and highly disruptive accomplishments paved the way for widespread adoption of electrodeposition as a key processing technology for other microelectronic devices. These events served, moreover, to attract researchers to the field and thus had a significant impact on electrochemical education.
Scientific advances were made during the 1990s in understanding small-scale phenomena associated with defects, nucleation, additive and solvent effects, surface films, and mechanisms of lattice and
dendrite formation, among many others. Powerful experimental methods continued to be developed for control and direct observation of the surface. Noteworthy among these was the invention and rapid development of scanning tunneling and atomic force microscopies for the observation of electrochemically active surfaces. These methods paved the way for several future decades by probing the electrochemical interface at unprecedented levels of resolution, from the wavelength of light down to the molecular scale. These events led to molecular-scale discoveries and potentially new devices and process inventions where control of events at the small scale was critical to product quality. These advances served to inspire the engineering community to develop multi-scale simulation tools that bridge both continuum and non-continuum phenomena.
By 2000, a strong base of continuum engineering methods was available for the design, scale-up, and optimization associated with building cells and production facilities, and controlling them during operation. Generally, however, the continuum approach was based on empirical characterization of the interfacial processes that appeared as “boundary conditions” in the transport analyses. But the previous several paragraphs indicate that electrochemical science had been moving downscale since the 1980s and, by this time, had generated much knowledge for future engineering and technology developments. In addition, it was recognized that events at the molecular scale play a significant role in determining product quality in many technological processes. This served to inspire electrochemical mathematical modeling efforts to include non-continuum stochastic methods, especially kinetic Monte Carlo, molecular dynamics, and quantum chemistry. The unique feature of these new capabilities is that they are intimately coupled in both old and new technologies that are widespread and that possess high monetary and energy value.
But there still remains a gap between our current scientific ability to understand small-scale phenomena, and our ability to incorporate it into routine engineering methods taught to
undergraduates. While new technological advances are today routinely envisioned, one might even say “glibly hyped,” the manufacturability of such devices requires precise, quantitative understanding at a magnitude, sophistication, and completeness that is extraordinarily difficult to assemble.
As this narrative approaches the most recent decade, we will abandon the historical perspective, and turn to some overarching themes that have emerged which, in our opinion, will continue to be highly relevant as we look to the future. The perspectives here are those of an engineer who uses science to deliver insights to technology.
Several high-level needs are clear:
1. Find the Thermodynamic Limit for the Entire System
• Today, candidates include new green electrodeposition processes, electrolytic syntheses based on H2 or CO2 for producing energy or chemicals, and new electrochemical separation technologies, all which require careful consideration of systems-level thermodynamics.
2. Focus on What Limits the Path Forward
• Over the past century, there have been periods when significant gaps existed between scientific understanding of electrochemical phenomena and our ability to incorporate it into engineering practice.
• These gaps tend to close when applications that are worth solving emerge and provide focus on what advances are needed.
• Today we have gaps. Today we have important applications. Today the system is primed making for significant advances. Our suggestion is to think not about the chemical process industry, but about its replacement: the ELECTROchemical process industry.
3.
• There are many electrochemical systems that have been studied over the course of many years, but that remain “near-miss” ideas. It is important to be alert to situations where new science or engineering materials and methods can provide fresh opportunities.
• Historically, many advances come from outside the electrochemical field. Virtually every field of engineering is currently engaged in responding to societal needs with new products and processes in energy, environment, medicine and health care, and information technology. Many of these advances involve understanding of molecular mechanisms, tailoring of new materials, and dealing with massive data repositories—all topics that are essential activities in the electrodeposition field. Many of these activities are producing results that could conceivably be re-directed to electrochemical applications. It is therefore important to read the literature widely, and with enough curiosity and informed judgment to recognize analogies between seemingly different situations
4.
• Today it is difficult to compare sophisticated experiments with sophisticated theory to test proposed hypotheses of mechanism.
• Mathematical models may be used to express the hypothesis by including underlying assumptions, chemical specifications, experimental protocols, operating conditions, system geometry, etc. This enables quantification of uncertainties in the hypothesis as well as the feasibility of linking experimental data with error bars, sensitivity analysis, parameter estimation, etc.
• Following this process will help make it possible for others to verify (and modify) your results to make it easy to test multiple reasonable hypotheses … and improve them.
5. Follow the Engineering Method
• Two essential tasks in the evaluation of new technological opportunities are (1) to determine the return on investment, and (2) to identify the technological barriers where improved scientific knowledge and/or invention is needed.
• Recognize that a system-wide engineering view involves uncertainty. This motivates the need for numerical efficiency associated with massively iterative simulations associated with error bars, sensitivity analysis, parameter estimation, optimization, etc.
• In addition, molecular manipulation will increasingly be treated as an engineering design focus, to exploit selfassembly of molecular-scale units during processing.
• It has become increasingly important to develop reusable electrochemical engineering methods that extended teams can use to align tight integration of discovery science, application design, research prototyping, and manufacturing collaboration.
Now let us end where we began. The Electrodeposition Division was founded on bringing together industrial practitioners and research scientists to discuss principles of chemistry, electricity, and metallurgy, and their use to guide practical improvements in products and processes. Since 1922, the integration of electrochemical science, engineering, and industrial practice has moved upscale as well as downscale to span 12 orders of magnitude. These activities have spawned the field of electrodeposition that today is as vibrant as those pioneers could ever have imagined when they gathered at that meeting in the spring of 1922 to form The Electrodeposition Division of The Electrochemical Society.
© The Electrochemical Society. DOI: 10.1149/2.F14224IF
Richard Alkire, Charles J. and Dorothy G. Prizer Chair Emeritus, University of Illinois Urbana-Champaign
Research Interests: Electrochemical Engineering.
Work Experience: Dr. Alkire has held a faculty position in the Department of Chemical Engineering since 1969.
Honors: Elected to the National Academy of Engineering in 1988.
Work with ECS: ECS President from 1986 to 1987.
Xiao Su, Assistant Professor of Chemical and Biomolecular Engineering, University of Illinois Urbana-Champaign
Education: Bachelor’s degree in Applied Sciences in Chemical Engineering (University of Waterloo), PhD in Chemical Engineering (MIT).
Research Interests: Development of electrochemical technologies for selective separations and process intensification.
Honors & Awards: NSF CAREER Award (2019), the Victor K. LaMer Award from the American Chemical Society (2020), and the ISE-Elsevier Prize for Green Electrochemistry (2021).
Website: https://xiaosugroup.web.illinois.edu/ https://orcid.org/0000-0001-7794-290X
Tetsuya Osaka, Professor of Applied Chemistry and Director of the Institute for Nanoscience and Nanotechnology, Waseda University Education: PhD in Engineering (Waseda University), post-doctoral fellowship at Georgetown University, Visiting Professor at the University of Minnesota.
Research Interests: Electrochemical
nanotechnology, including electro- and electroless-deposition/ surface finishing, electronic packaging materials, magnetic storage and energy storage devices, and chemical- and bio-sensors.
Work Experience: Dr. Osaka’s past appointments include Director, Department of Applied Chemistry; Dean, Graduate School of Science and Engineering; Provost, Research Promotion Division; Director, Research Institute for Science and Engineering; and Deputy Dean, Faculty of Research and Engineering, all at Waseda University.
Pubs + Patents: Author and/or editor >70 books and >890 original and review papers. Highly Cited Researcher, Materials Science, ISIHighlyCited.com
Work with ECS: A member of ECS since 1979, Dr. Osaka has served as a leading organizer and a co-editor of many ECS proceedings volumes. He has received the Research Award of the Electrodeposition Division of ECS, and was elected Fellow of ECS in 2002. He has served as President of the Electrochemical Society of Japan, Chair of the ECS Japan Section, and was ECS President from 2013 to 2014.
Honors & Awards: Medal with Purple Ribbon bestowed by the Decoration Bureau of the Cabinet Office; Japan Prizes for Science and Technology in the Development Category of the Commendation for Science and Technology; Society Award of the Magnetics Society of Japan; Chemical Society of Japan Award; Pergamon Electrochimica Acta Gold Medal; Society Award of the Surface Finishing Society of Japan; and Simon Wernic International Award of the International Union for Surface Finishing.
The ECS Japan Section launched its official website this past spring. The site at https://ecsjapansection.jp/ includes information on the section’s events as well as reports from branches and membership news. Some branches have already posted their news there.
The 34th ECS Japan Tohoku branch held its Young Scientists Meeting as a joint meeting with the ECSJ Tokai Branch online via Zoom on November 22–23, 2021. The meeting, supported by ECS, brought together 80 young students and researchers to discuss a wide range of electrochemistry topics (bioelectrochemistry, batteries, organic electrochemistry, and more). Students and postdocs made 35 oral presentations, and prizes for oral presentations were awarded to 4 students from the Tohoku Branch and 4 from the Tokai Branch. The SEMI Conference, organized by the ECSJ Tohoku and Tokai branches, took place at the same time as the Young Scientists Meeting. Senior participants from the SEMI Conference joined the Young Scientists Meeting and provided inspiration and guidance to young researchers.
The 3rd Kansai Denki-Kagaku Kenkyukai webinar was held online via Remo and Zoom on December 4, 2021. The event attracted 230 participants and included an awards ceremony where the KansaiDenki-Kagaku-Shourei-Shou Award was presented to 12 authors.
The 29th International Workshop on Active-Matrix Flat Panel Displays and Devices: TFT Technologies and FPD Materials (AMFPD ʼ22) took place in Kyoto from July 5 to 8, 2022. The hybrid event was held live and on Zoom. The workshop had 111 participants from 14 countries. Of the 64 papers presented, 3 were keynote speeches and 5 were invited talks. In addition, the Japan Section held an award ceremony online on February 2, 2022, in which Dr. Juan Paolo Bermundo of the Nara Institute of Science and Technology received the AMFPD-ECS Japan Section Young Researcher Award
Arizona Section Candace Kay Chan, Chair
Brazil Section Luis F. P. Dick, Chair
Canada Section Heather Andreas, Chair
Chile Section José H. Zagal, Chair
China Section Yongyao Xia, Chair
Detroit Section Eric Anderson, Chair
Europe Section Philippe Marcus, Chair
Georgia Section Open
India Section Sinthai A. Ilangovan, Chair
Israel Section Daniel Mandler, Chair
Japan Section Seiichi Mayazaki, Chair
Korea Section Won-Sub Yoon, Chair
Mexico Section Carlos E. Frontana-Vázquez, Chair
Mid-America Section Andrew J. Wilson, Chair
National Capital Section Chunsheng Wang, Chair
New England Section Sanjeev Mukerjee, Chair
Pacific Northwest Section Jie Xiao, Chair
Pittsburgh Section Open
San Francisco Section Gao Liu, Chair
Singapore Section Zichuan J. Xu, Chair
Taiwan Section Chi-Chang Hu, Chair
Texas Section Jeremy P. Meyers, Chair
Twin Cities Section Victoria (Vicki) Gelling, Chair
Visit https://www.electrochem.org/sections to learn more about ECS sections.
The Honors & Awards Program recognizes outstanding technical achievements in electrochemistry and solid state science and technology, and exceptional service to the Society, through ECS Society, Division, Section, and Student Awards.
Highlights follow. Visit www.electrochem.org/awards for more information.
The ECS Toyota Young Investigator Fellowship, established in 2015 in partnership with the Toyota Research Institute of North America, encourages young professionals and scholars to pursue research into batteries, fuel cells, and hydrogen, and future sustainable technologies. Each year, at least one candidate receives the fellowship restricted grant of no less than US $50,000 to conduct the proposed research within one year, and a one-year complimentary ECS membership. Recipients must present at a Society biannual meeting and publish their research in a relevant ECS journal within 24 months of receiving the award. Materials are due January 31 annually.
Fellow of the Electrochemical Society, established in 1989 as the Society’s highest honor, recognizes advanced individual technological contributions in the field of electrochemistry and solid state science and technology, and active ECS membership. The award consists of a framed certificate and lapel pin. Materials are due February 1, 2023.
The Henry B. Linford Award for Distinguished Teaching was established in 1981 for excellence in teaching in subject areas of interest to the Society. The award consists of a silver medal and plaque containing a bronze replica thereof; $2,500 prize; Society Life Membership; and complimentary meeting registration. Materials are due April 15, 2023.
The Vittorio de Nora Award, established in 1971, recognizes distinguished contributions to the field of electrochemical engineering and technology. The award consists of a gold medal and plaque that contains a bronze replica thereof; $7,500; Society Life Membership; and complimentary meeting registration. Materials are due April 15, 2023.
Leadership Circle Awards, established in 2002 to honor and thank our electrochemistry and solid state science partners, are granted in the anniversary year that an institutional member reaches a milestone level. Awardees receive a commemorative plaque and recognition on the ECS website and in Interface Nominations are not accepted.
The Organic and Biological Electrochemistry Division Manuel M. Baizer Award, established in 1992, recognizes outstanding scientific achievements in the electrochemistry of organics and organometallic compounds, carbon-based polymers and biomass, whether fundamental or applied, and including but not limited to synthesis, mechanistic studies, engineering of processes, electrocatalysis, devices such as sensors, pollution control, and separation/recovery. The award consists of a framed certificate and US $1,000 prize. Materials are due by January 15, 2023.
The High-Temperature Division J. Bruce Wagner, Jr. Young Investigator Award, established in 1998, recognizes a young Society member who demonstrates exceptional promise for a successful career in science and/ or technology in the field of high-temperature materials. The award consists of a scroll and US $1,000. The recipient may receive complimentary meeting registration and up to US $1,000 in travel expenses. Materials are due by February 1, 2023.
Biannual Meeting Travel Grants are awarded for each Society biannual meeting. Many ECS divisions and sections offer travel grants to undergraduates, graduate students, postdoctoral researchers, and young professionals and faculty presenting papers at ECS biannual meetings. The awards consist of financial support ranging from complimentary meeting registration to luncheon/ reception tickets, travel support, and more. Divisions and sections maintain their own application requirements. 243rd ECS Meeting Travel Grant applications are due by February 27, 2023.
The Physical and Analytical Electrochemistry Division Max Bredig Award in Molten Salt and Ionic Liquid Chemistry was founded in 1984 to recognize excellence in the field and to stimulate publication of high quality research papers in this area in the Journal of The Electrochemical Society. The award consists of a scroll and US $1,500 prize. Materials are due by March 1, 2023.
The Sensor Division Early Career Award, established in 2021, recognizes promising early career engineers’ and scientists’ research contributions to the field of sensors. The award consists of a framed certificate; US $500 prize; complimentary meeting registration; and Sensor Division business luncheon ticket. Materials are due by March 1, 2023.
The Sensor Division Outstanding Achievement Award was created in 1989 to recognize outstanding achievement in research and/or technical contributions to the field of sensors and to encourage work excellence in the field. The award consists of a framed certificate and US $1,000 prize. Materials are due by March 1, 2023.
The Battery Division Early Career Award Sponsored by Neware Technology Limited was launched in 2020 to encourage excellence among postdoctoral researchers in battery and fuel cell research with the primary purpose of recognizing and supporting the development of talent and future leaders in battery and fuel cell science and technology. The award consists of a framed scroll; US $2,000; and complimentary meeting registration. Materials are due by March 15, 2023.
The Battery Division Postdoctoral Associate Research Award Sponsored by MTI Corporation and the Jiang Family Foundation, established in 2016, encourages excellence among postdoctoral researchers in battery and fuel cell research. The award consists of a framed scroll; US $2,000; and complimentary meeting registration. Two awards are granted each year. Materials are due by March 15, 2023.
The Battery Division Research Award was established in 1958 to recognize excellence in battery and fuel cell research and encourages publication in ECS journals. The award of a framed certificate and US $2,000 recognizes outstanding contributions to the science of primary and secondary cells, batteries, and fuel cells. Materials are due by March 15, 2023.
The Battery Division Technology Award, established in 1993, encourages the development of battery and fuel cell technology, and recognizes significant achievements in this area. The award’s field of interest is “that area of electrochemical technology which deals with the design, fabrication, scale-up, performance, lifetime, operation, control, and application of devices (i.e., primary and secondary cells and batteries, and fuel cells) in which chemical energy can be converted into usable electrical energy by an electrochemical process.” The award consists of a scroll; US $2,000; and Battery Division membership while the recipient is an ECS member. Materials are due by March 15, 2023.
The Electrodeposition Division Early Career Investigator Award, was established in 2015 to recognize an outstanding young researcher in the field of electrochemical deposition science and technology. The award consists of a framed certificate and US $1,000. Materials are due by April 1, 2023
The Electrodeposition Division Research Award recognizes outstanding research contributions to the field of electrodeposition and encourages the publication of high-quality papers in the Journal of The Electrochemical Society. The award is based on recent outstanding achievement in, or contributions to, the field of electrodeposition, and is given to an author/co-author of a paper published in JES or another ECS publication. The award consists of a framed certificate and US $2,000. Materials are due by April 1, 2023.
The Energy Technology Division Walter van Schalkwijk Award in Sustainable Energy Technology was established in 2021 to recognize and reward researcher scientists, academicians, and entrepreneurs who make innovative and transformative contributions to sustainable energy technologies (devices, materials, and/or processes). The award consists of a framed certificate and monetary prize equal to 1/25th of the endowment with a maximum of US $2,500. Materials are due by April 15, 2023.
The Canada Section W. Lash Miller Award, established in 1967, recognizes publications and/or excellence in the field of electrochemical science and technology and/or solid state science and technology. Recipients must be Canadian residents who completed their last advanced education degree no more than 15 years before the award year. The award is a framed certificate and CAD $1,500 . Materials are due December 31, 2022.
The ECS Korea Section Student Award, established in 2005, recognizes academic accomplishments in any area of science or engineering in which electrochemical and/or solid state science and technology is the central consideration. The US $500 prize is presented at a designated Korea Section meeting. The recipient may be asked to speak at that meeting on a subject of major interest to him/her in the field of electrochemical and/or solid state science and technology. Materials are due by December 31, 2022.
The Colin Garfield Fink Fellowship, first awarded in 1962, assists a postdoctoral scientist/researcher pursue research from June through August in a field of interest to the Society. The award consists of US $5,000 and publication of a summary report in the award year’s Interface winter issue. Materials are due by January 15, 2023.
ECS Summer Fellowships, established in 1928, assist students pursuing research from June through August in a field of interest to ECS. Up to four summer fellowships are awarded each year: the Edward G. Weston Fellowship, Joseph W. Richards Fellowship, F. M. Becket Fellowship, and H. H. Uhlig Fellowship. Recipients receive US $5,000 to support their research and publication of a summary report in the award year’s Interface winter issue. Materials are due by January 15, 2023.
The San Francisco Section Daniel Cubicciotti Student Award, was established in 1994 to assist a deserving student in Northern California pursue a career in the physical sciences or engineering. The award consists of an etched metal plaque and US $2,000 prize. Up to two honorable mentions are also extended, each receiving a framed certificate and US $500 prize. Materials are due by February 15, 2023.
The Canada Section Student Award was created in 1987 to recognize promising young engineers and scientists in the field of electrochemical power sources and encourage them to initiate or continue careers in the field. The award consists of a US $1,500 prize. Materials are due February 28, 2023.
The Pacific Northwest Section Electrochemistry Student Award Sponsored by Thermo Fisher Scientific was established in 2021 to recognize promising young engineers and scientists in Washington, Oregon, and Idaho pursuing PhDs in the fields of electrochemical engineering and applied electrochemistry. The award consists of a US $1,000 prize. Materials are due by February 28, 2023.
The Sensor Division Student Research Award was established in 2021 to recognize promising students in their graduate training for conducting outstanding research in the field of sensors. The award consists of a framed certificate; US $200 prize; complimentary meeting registration; and Sensor Division business luncheon ticket. Materials are due by March 1, 2023.
The Battery Division Student Research Award Sponsored by Mercedes-Benz Research & Development recognizes promising young engineers and scientists in the field of electrochemical power sources. The award of a framed certificate and US $1,000 encourages recipients to initiate or continue careers in the field. Eligible candidates must be enrolled in a college or university at the nomination deadline. Materials are due by March 15, 2023.
The ECS Outstanding Student Chapter Award (formerly The Gwendolyn B. Wood Section Excellence Award) was launched in 2012 to recognize distinguished student chapters that demonstrate active participation in the Society’s technical activities; establish community and outreach activities in the areas of electrochemical and solid state science and engineering education; and create and maintain a robust membership base. The winning Outstanding Student Chapter receives a recognition plaque; US $1,000; and award recognition and chapter group photo in Interface or other electronic communications. Up to two Chapters of Excellence are named and receive recognition certificates and acknowledgement in Interface Materials are due by April 15, 2023.
Chi Won Ahn, Daejeon, Chungcheongnamdo, South Korea
Lazbourne Allie, Atlanta, GA, USA
Juveiriah M. Ashraf, Masdar City, Abu Dhabi, UAE
Kobra Azizi, Kvistgard, Hovedstaden, Denmark
Youngjoon Bae, Suwon, Gyeonggi-do, South Korea
Andrea Ballabio, Dolzago, Lombardia, Italy
Andrea Barzaghi, Nova Milanese, Lombardia, Italy
Rajesh Bashyam, Bolingbrook, IL, USA
Elena Belyanovskaya, Dnipro, Dnipropetrovsk, Ukraine
Sriparna Bhattacharya, Clemson, SC, USA
Bhaskar Bhuyan, Sunnyvale, CA, USA
Wengang Bi, Santa Clara, CA, USA
David Bilby, Royal Oak, MI, USA
Nicholas Boruta, Palmdale, CA, USA
Helen Cativo, Newark, DE, USA
Yen-Hung Chen, Fremont, CA, USA
Kangwoo Cho, Pohang, Gyeongsangbuk-do (north), South Korea
Younghyun Cho, Asan, Chungcheongnam-do (South), South Korea
Pyoungho Choi, Huntsville, AL, USA
Yoon-Seok Choi, Athens, OH, USA
James Coates, Prosser, WA, USA
Omar Concepcion Diaz, Juelich, North Rhine-Westphalia, Germany
Perceval Coudrain, Grenoble, AuvergneRhone-Alpes, France
Mitul Dalal, South Grafton, MA, USA
Timothy Davenport, Cumberland, RI, USA
Fabien Deprat, Crolles Cedex, AuvergneRhone-Alpes, France
Joseph DuChene, Amherst, MA, USA
Peter Ercius, Berkeley, CA, USA
Claudio Fontanesi, Modena, EmiliaRomagna, Italy
Mounesha Garaga, New York, NY, USA
Yannick Garsany, Washington, DC, USA
Marco Giorgetti, Bologna, Emilia-Romagna, Italy
Mikaela Gorlin, Cambridge, MA, USA
Andrew Greytak, Columbia, SC, USA
Anke Hagen, Kongens Lyngby, Hovedstaden, Denmark
Hyung Chul Ham, Incheon, Gyeonggi-do, South Korea
Hee Han, Daejeon, Chungcheongnam-do, South Korea
Hyun Soo Han, Stanford, CA, USA Nurul Hasan, Melbourne, Victoria, Australia
Tadao Hashimoto, Buellton, CA, USA
Hideo Hosono, Yamato, Kanagawa, Japan Boxun Hu, Moraga, CA, USA
Joshua Hudgens, Knoxville, TN, USA Craig Hughes, Charlotte, NC, USA
Giovanni Isella, Como, Lombardia, Italy
Seonah Jin, Capitola, CA, USA
Nathaniel Jones, Pendergrass, GA, USA
Abhijeet Joshi, Scotts Valley, CA, USA
Sang-Mun Jung, Pohang, Gyeongsangbukdo, South Korea
Ali Kamali, Shenyang, Liaoning, China
Joël Kanyandekwe, Grenoble, AuvergneRhone-Alpes, France
Matthew Kastelic, Cambridge, MA, USA
Stephen Kelly, San Francisco, CA, USA
Rami Khazaka, Leuven, Leuven, Belgium
Byunghyuk Kim, Daejeon, Chungcheongnam-do, South Korea
Hoon Kim, Painted Post, NY, USA
Mokwon Kim, Suwon, Gyeonggi-do, South Korea
Sewon Kim, Suwon, Gyeonggi-do, South Korea
Younsoo Kim, Suwon-si, Gyeonggi-do, South Korea
Senthilkumar Krishnan, Faridabad, HR, India
Krzysztof Kruczala, Krakow, Małopolska, Poland
Ashok Kumar, Columbus, IN, USA
Balasubramanian Lakshmanan, Newark, DE, USA
Adam Lange, Franksville, WI, USA
John Lau, Mountain View, CA, USA
Quoc Bao Le, Zlín, Zlín, Czech Republic
Connor Leach, Beltsville, MD, USA
James LeBeau, Cambridge, MA, USA
Jang-Sik Lee, Pohang, Gyeongsangbuk-do, South Korea
Keonhag Lee, Berkeley, CA, USA
Myeong-Hee Lee, Ulsan, Gyeonggi-do, South Korea
Yonghee Lee, Daejeon, Chungcheongnamdo, South Korea
Jichao Li, Plymouth, MI, USA
Mengya Li, Knoxville, TN, USA
Jin-hyung Lim, Austin, TX, USA
Sung Lim, Alameda, CA, USA
Hansan Liu, Historic New Castle, DE, USA
Xiangquan Liu, Beijing, Beijing, China
Patrick Lomenzo, Whiting, NJ, USA
Sunil Lonkar, Masdar City, Abu Dahbi, UAE
Eduardo Magdaluyo Jr., Quezon City, Metro Manila, Philippines
Joe Margetis, Tempe, AZ, USA
Ivan Marri, Carpi, Emilia-Romagna, Italy
James Martin, Raleigh, NC, USA
Thangaraj Mathuraiveeran, Columbus, IN, USA
Jesse McDaniel, Atlanta, GA, USA
Naoki Mitsuta, Hagamachi, Tochigi, Japan
Shogo Mochizuki, Albany, NY, USA
Eun Ju Moon, Painted Post, NY, USA
Geon Dae Moon, Yangsan-si, Gyeongsangnam-do, South Korea
Anja-Verena Mudring, Aarhus C., Midtjyllan, Denmark
Wayne Mullinax, Boiling Springs, SC, USA
Nicie Murphy, Knoxville, TN, USA
Thomas Nunan, Marlborough, MA, USA
Michael Oehme, Stuttgart, BadenWuerttemberg, Germany
Anatoliy Onanko, Кyiv, Kyiv Oblast, Ukraine
Erin Ortiz, Fayetteville, NC, USA
Seijung Park, Yongin-si, Gyeonggi-do, South Korea
Kavitha Parvataneni, Historic New Castle, DE, USA
Anna Peczek, Frankfurt Oder, Brandenburg, Germany
Jack Pekarik, Essex Junction, VT, USA
Denis Phares, Reno, NV, USA
Raphael Prentki, Montreal, QC, Canada
Hanna Qin, Maple Ridge, BC, Canada
Uppili Raghunathan, Essex Junction, VT, USA
ECS is proud to announce the following new members for July, August, & September 2022 (Members are listed alphabetically by family/last name.)V. S. Raja, Mumbai, MH, India
Siddharth Rajan, Columbus, OH, USA
Apparao Rao, Clemson, SC, USA
William Rauch, Atlanta, GA, USA
Danielle Reifsnyder Hickey, University Park, PA, USA
Taylor Reynolds, Idaho Falls, ID, USA
Fernando Rhen, Limerick, Munster, Ireland
Won Ryoo, Seoul, Gyeonggi-do, South Korea
Morteza Sabet, Greenville, SC, USA
Gudrun Saevarsdottir, Reykjavik, RVK, Iceland
Giordano Scappucci, Delft, South Holland, Netherlands
Daniel Schwarz, Stuttgart, BadenWuerttemberg, Germany
Tamar Segal-Peretz, Haifa, Haifa, Israel
Lee Seokhee, Daejeon, Chungcheongnam-do, South Korea
Nedjeljko Seselj, Kvistgård, Hovedstaden, Denmark
Abdul Shakoor, Doha, Qatar, Qatar
Partha Sheet, Ann Arbor, MI, USA
Glenn Sklar, San Diego, CA, USA
Malgorzata Sojka, Houston, TX, USA
Sreeprasad Sreenivasan, El Paso, TX, USA
Viranjay Srivastava, Durban, Kwazulu Natal, South Africa
Lorenzo Stievano, Montpellier, Occitanie, France
Dmitry Suyatin, Lund, Skane, Sweden
Takaoki Takeshita, Kariya-shi, Aichi, Japan
Andrew Teplyakov, Newark, DE, USA
John Texter, Rochester, NY, USA
Susanna Thon, Baltimore, MD, USA
Mara Tolksdorf, Silver Spring, MD, USA
Suguru Uemura, Sapporo, Hokkaido, Japan
Vinod Upadhyay, Tyrone, GA, USA
Mohamed Shahid Usen Nazreen, San Antonio, TX, USA
J. van Ommen, Delft, South Holland, Netherlands
Lieven Vandersypen, Delft, South Holland, Netherlands
Justin Virgili, Berkeley, CA, USA
Anil Virkar, Salt Lake City, UT, USA
Franceska Waggett, Billingham, North East, UK Shanshan Wang, Acworth, GA, USA Wei-Chen Wen, Frankfurt, Brandenburg, Germany
Feina Xu, Vandoeuvre-les-Nancy, Grand Est, France
Sachin Yadav, Leuven, Flemish Brabant, Belgium
Yuji Yamamoto, Frankfurt, Brandenburg, Germany
Ran Yi, Richland, WA, USA
Ryo Yokogawa, Kawasaki, Kanagawa, Japan
Donghwan Yoon, Yongin-si, Gyeonggi-do, South Korea
Kazutoshi Yoshioka, Kawasaki, Kanagawa, Japan
Brian Zappella, Walpole, MA, USA
Li Zhang, Tinley Park, IL, USA
Junjie Zhao, Hangzhou, Zhejiang, China
Qing-Tai Zhao, Juelich, North RhineWestphalia, Germany
Sichen Zhong, Auburndale, MA, USA
Muhammad Abbas, Richardson, TX, USA
Rose Anne Acedera, Quezon City, Metro Manila, Philippines
Rudy Acosta, Fayetteville, AR, USA
Priyanka Adapala, Lancaster, OH, USA
Christabel Adjah-Tetteh, Lafayette, LA, USA
Liezle Agasino, General Trias, Cavite, Philippines
Sheikh Imran Uddin Ahmed, San Antonio, TX, USA
Yoojin Ahn, Algonquin, IL, USA
Omer Aksoy, Istanbul, Istanbul, Turkey
Rayan Alaufey, Philadelphia, PA, USA
Aljawharah Alsharif, Thuwal, Makkah Province, Saudi Arabia
Shuichiro Amatsuka, Sendai, Miyagi, Japan Ketan Anand, Frankfurt, Brandenburg, Germany
Grace Anderson, Berkeley, CA, USA
Tanvir Alam Arman, Albuquerque, NM, USA
Airon Rae Asuncion, Quezon City, Metro Manila, Philippines
Abhinand Ayyaswamy, Lafayette, IN, USA
Mahsa Bagi, Columbia, SC, USA
Elnaz Bahmani, Hamilton, ON, Canada
Jaira Neibel Bamba, San Fernando, Pampanga, Philippines
Sourim Banerjee, West Lafayette, IN, USA
Robert Barber, Belfast, Northern Ireland, UK
Sarmistha Baruah, North Guwahati, AS, India
Igor Bezsonov, Lansing, MI, USA
Rashmi Bhaskaran, Chennai, TN, India
Md Sajibul Alam Bhuyan, Indianapolis, IN, USA
Yanhong Bian, Princeton, NJ, USA Aidan Billings, Columbia, SC, USA
Markus Binder, Ulm, Baden-Wuerttemberg, Germany
Ashley Bird, Richmond, CA, USA
Rowan Blake, Bellshill, Scotland, UK
Martina Borges, East Lansing, MI, USA
Jenny Borjesson Axen, Stockholm, Sodermanland and Uppland, Sweden
Michael Borrello, Berkeley, CA, USA
Scott Bowers, Bloomington, IN, USA
Victor Brandao, Atlanta, GA, USA
Sonja Brankovic, Atlanta, GA, USA
Grace Buckey, Cincinnati, OH, USA
Meng Cao, Wuhan, Hubei, China
John Carbo, Baltimore, MD, USA
Neal Cardoza, Philadelphia, PA, USA
Cole Carpenter, Arvada, CO, USA
Celine Chen, Lake Forest, CA, USA
Chen Chen, Xi’an, Shaanxi Province, China
Xunkai Chen, Princeton, NJ, USA
Zhitao Chen, Atlanta, GA, USA
Yoshihiro Chida, Sendai, Miyagi, Japan
Jinwon Cho, Atlanta, GA, USA
Gihun Choe, Atlanta, GA, USA
Jung-goo Choi, Gwangju, Jeollanam-do, South Korea
Minjun Choi, Gwangju, Jeollanam-do, South Korea
Pilsoo Choi, Gwangju, Jeollanam-do, South Korea
Jens Christensen, Bagsværd, Hovedstaden, Denmark
Woowon Chung, Ansan, Gyeonggi-do, South Korea
Natalie Click, Chandler, AZ, USA
Matthew Coats, Golden, CO, USA
Kailyn Cohen, Cherry Hill, NJ, USA
Patrick Combs, Stillwater, OK, USA
John Larry Corpuz, Cuenca, Batangas, Philippines
Stephen Cotty, Champaign, IL, USA
Mohana Priya D. B., Chennai, TN, India
Suman Das, Auburn, AL, USA
Ashley David, Tallahassee, FL, USA
Wedage Dayaratne, South Bend, IN, USA
Marcel Roy Domalanta, Quezon City, Quezon Ci, Philippines
Stephanie Dulovic, Plainsboro, NJ, USA
Alicia Theresse Dumlao, Quezon City, Metro Manila, Philippines
Mohammed Eldeeb, Richardson, TX, USA
Casey Elliott, Reno, NV, USA
Shengqi Fan, Boston, MA, USA
Yuge Feng, Xi’an, Shaanxi Province, China
Giovanni Ferro, Irvine, CA, USA
Marvin Frauenrath, Grenoble, AuvergneRhone-Alpes, France
Sven Friedrich, Munich, Bavaria, Germany
Yu Fu, Buffalo, NY, USA
Bala Krishnan Ganesan, Gwangju, Jeollabuk-do, South Korea
Jiaxin Gao, Baltimore, MD, USA
Jayson Garcia, Quezon City, Metro Manila, Philippines
ThomasJae Garcia, Los Angeles, CA, USA
Jon Nyner Gavan, Quezon City, Metro Manila, Philippines
Nithya George, Mavelikkara, KL, India
Basanta Ghimire, Clemson, SC, USA
Suvash Ghimire, Orlando, FL, USA
Rupayan Ghosh, Blacksburg, VA, USA
Sayan Ghosh, Chennai, TN, India
Tianyao Gong, Baltimore, MD, USA
Nikhil Govindarajan, Atlanta, GA, USA
Cameron Grant, Greenwood, IN, USA
Joshua Grant, Fayetteville, AR, USA
Adam Grosvirt-Dramen, Irvine, CA, USA
Baichuan Guo, Baltimore, MD, USA
Jinyu Guo, Stanford, CA, USA
Pramod Gupta, Sultanpur, UP, India
Asanka Gurukandure, Auburn, AL, USA
Sangmun Han, Seoul, Gyeonggi-do, South Korea
Yingjie Hang, Amherst, MA, USA
Shomaz Ul Haq, Knoxville, TN, USA
Zenifar Haque, Lubbock, TX, USA
Rong He, Las Cruces, NM, USA
Simon Helmer, Garching, Bavaria, Germany
Alma Hernandez Gonzalez, Princeton, NJ, USA
Dabeen Hong, Gwangju, Jeollanam-do, South Korea
Seung-Bo Hong, Seoul, Gyeonggi-do, South Korea
Mahmudul Hoq, Indianapolis, IN, USA
Jie Hou, Marietta, GA, USA
Xueyu Hu, Atlanta, GA, USA
Xun Huang, Xian, Shaanxi Province, China
Shineui Hwang, Atlanta, GA, USA
MD Mahdi Ul Ishtiaque, Ames, IA, USA
Rohit Jadhav, Salt Lake City, UT, USA
Nicole James, Provo, UT, USA
Dahye Jeong, Cheonan, Chungcheongnamdo, South Korea
Jinhyeon Jo, Gwangju, Jeollanam-do, South Korea
Hyo-Jun Joo, Singapore, Singapore, Singapore
Skylar Jordan, Marietta, GA, USA
Lolly Jose, Kallumala, KL, India
Sreerag Kaaliveetil, Newark, NJ, USA
Amrit Kafle, Silver Spring, MD, USA
Naisargi Kanabar, Albany, NY, USA
Ganapathi Rao Kandregula, Chennai, TN, India
Donghyeon Kang, Lemont, IL, USA
Sinwoo Kang, Gwangju, Gyeonggi-do, South Korea
Peshal Karki, Clemson, SC, USA
Avijit Karmakar, Lafayette, IN, USA
Niroshan Karunarathne, Vienna, Upper Austria, Austria
Amin Kazem Ghamsari, Tampa, FL, USA Bethany Kersten, Cleveland Heights, OH, USA
Harun Khan, Chennai, TN, India
Kishwar Khan, Atlanta, GA, USA Chanho Kim, Atlanta, GA, USA
Doyeub Kim, Atlanta, GA, USA Jae-Min Kim, Seoul, Gyeonggi-do, South Korea
Jung Tae Kim, Maple Ridge, BC, Canada Kyu-Su Kim, Pohang, Gyeongsangbuk-do, South Korea
Min-Sung Kim, Gwangju, Jeollanam-do, South Korea
Myoung Chan Kim, Seoul, Gyeonggi-do, South Korea Nayeong Kim, Champaign, IL, USA
Youngmin Kim, Singapore, Singapore, Singapore
Trevor Kramer, Springboro, OH, USA
Nitish Kumar, Surrey, BC, Canada Chao Kun, Xi’an, Shaanxi Province, China Tzu-Wen Kuo, Hsinchu, Hsinchu County, Taiwan
Kevin Lester Lobo, Mandaluyong, Metro Manila, Philippines
Quentin Lomonaco, Grenoble, AuvergneRhone-Alpes, France
Mary Long, Thomasville, AL, USA
Daniel Eldrei Loresca, Quezon City, Metro Manila, Philippines
William Lowery, Nashville, TN, USA
Xinlin Lu, Atlanta, GA, USA
Sophia Bernadette Lunor, Bacnotan, La Union, Philippines
K. A. U. Madhushani, Pittsburg, KS, USA
Megan Magness, Farmington, AR, USA
Casey Mahony, Altona, NY, USA
Fares Maimani, Redondo Beach, CA, USA
Akmal Irfan Majid, Eindhoven, North Brabant, Netherlands
Meysam Maleki, Tehran, Tehran, Iran
Anne-Flore Mallet, Crolles, AuvergneRhone-Alpes, France
Sukdeb Mandal, Durgapur, WB, India
Refiloe Maphoto, Polokwane, Limpopo, South Africa
Raul Marquez-Montes, Austin, TX, USA
Anmol Mathur, Baltimore, MD, USA
D. J. Donn Matienzo, Quezon City, Metro Manila, Philippines
Kyle Matthews, Philadelphia, PA, USA
Calla McCulley, Huntsville, AL, USA
LHunter McRay, Mechanicsville, VA, USA
Marc Francis Labata, Quezon City, Metro Manila, Philippines
Nathaniel Larm, Annapolis, MD, USA
Saheed Lateef, Columbia, SC, USA
Josef Lawrence, Princeton, NJ, USA
Christian Lechtenfeld, Muenster, North Rhine-Westphalia, Germany
Jin Wook Lee, Seoul, Gyeonggi-do, South Korea
Minyoung Lee, Cheonan, Chungcheongnamdo, South Korea
Seojun Lee, Gwangju, Jeollanam-do, South Korea
Soobeen Lee, Seoul, Gyeonggi-do, South Korea
Young-Jun Lee, Seoul, Gyeonggi-do, South Korea
Brian Lenhart, Columbia, SC, USA
Justine Lespiaux, Crolles, Auvergne-RhoneAlpes, France
Haixia Li, Columbia, SC, USA
Jiawei Li, Wuhan, Hubei, China
Mohan Li, Darmstadt, Hesse H, Germany
Qiang Li, Xi’an, Shaanxi Province, China
Yaqi Li, Aalborg East, Aalborg, Nordjylland, Denmark
Humphrey Lin, Atlanta, GA, USA
Federico Lissandrello, Lodi, Lombardia, Italy
Hang Liu, Wuhan, Hebei, China
Quanhong Liu, Tuscaloosa, AL, USA
Tong Liu, Pittsburgh, PA, USA
Yujia Liu, Berlin, Brandenburg, Germany
William Liyayi, Sheffield, South Yorkshire, England, UK
Emil Lobachev, Tallahassee, FL, USA
Anoop Meleri, Thrissur, KL, India
John Miller, Lafayette, LA, USA
Nikhil Mohan, Chennai, TN, India
Craig Moore, Reno, NV, USA
James Moore, Amherst, MA, USA
Megala Moorthy, Gwangju, Jeollanam-do, South Korea
Lincoln Mtemeri, Lansing, MI, USA
Maxwell Myers, Erie, PA, USA
Santiago Naranjo, Richmond, VA, USA
Elina Nazmutdinova, Muenster, North Rhine-Westphalia, Germany
Zachary Nguyen, Bluffdale, UT, USA
Xiu Niu, Xi’an, Shaanxi Province, China
Hyunjun Oh, Gwangju, Jeollanam-do, South Korea
Afolabi Olayiwola, Blacksburg, VA, USA
Alexandra Oliveira, Newark, DE, USA
Darrell Omo-Lamai, Philadelphia, PA, USA
Maura Andrea Ortega, Quezon City, Metro Manila, Philippines
Olivia Owens, Panama Ciy, FL, USA
Solomon Oyakhire, Stanford, CA, USA
Mecaelah Palaganas, Taguig, Metro Manila, Philippines
Krishnaveni Palanisamy, Ulm, BadenWuerttemberg, Germany
Hui Pan, Heverlee, Vlaams Brabant, Belgium
Swati Panigrahi, Chennai, TN, India
Andrew Park, Bordentown, NJ, USA
Mark Joseph Pasciolco, Clearwater Bay, Sai Kung, Hong Kong
Daniel Patosa, Tanza, Cavite, Philippines
Michael Patron, Pasig City, National Capital Region, Philippines
Rogelio Paulino Jr., City of Dasmarinas, Cavite, Philippines
Mahender Peddi, Chennai, TN, India
Jakub Pepas, Atlanta, GA, USA
A. A. P. R. Perera, Pittsburg, KS, USA
Tram Phung, Palaiseau, Ile-de-France, France
Sofia Pinzon, Santa Fe, NM, USA
Jan Pleie, Muenster, North Rhine-Westphalia, Germany
Christopher Poches, Rapid City, SD, USA
Sravani Potham, Chennai, TN, India
Vineeth Pothanamkandathil, State College, PA, USA
Darlene Pudolin, Manila, Metro Manila, Philippines
Lady Quispe Garrido, Lima, Lima, Peru
Marta Ragu, Pontypridd, Wales, UK
Sabari Rajendran, Manhattan, KS, USA
Rhoda Lyn Ramos, Trece Martires City, Cavite, Philippines
Austin Reese, Ithaca, NY, USA
Chloe Renfro, Neosho, MO, USA
Gianluca Rengo, Leuven, Leuven, Belgium
Thomas Roth, Munich, Bavaria, Germany
Christian Ruiz, Santa Fe, NM, USA
Maria Rybalchenko, Shannon, Munster, Ireland
Je-gwang Ryu, Gwang-ju, Gyeonggi-do, South Korea
Nicole Sagui, Uppsala, Uplandia, Sweden
Keita Sahara, Kawasaki City, Kanagawa, Japan
Abhilipsa Sahoo, Chennai, TN, India
Arizeo Salac, Mariveles, Bataan, Philippines
Rodney Salazar, Turku, Southwest Finland, Finland
Bibek Samanta, Muenster, North RhineWestphalia, Germany
Giancarlo Sanglay, Dumaguete City, Negros Oriental, Philippines
Nawraj Sapkota, Clemson, SC, USA
Joachim Schaeffer, Berlin, Berlin, Germany
Vaidik Shah, Ithaca, NY, USA
Jensheer Shamsudeen Seenath, Montréal, QC, Canada
Parul Sharma, Stillwater, OK, USA
Yuki Shibayama, Chiyoda, Kanagawa, Japan
Jinha Shim, Anyang, Gyeonggi, South Korea
Qingdian Shu, Leeuwarden, Friesland, Netherlands
Tana Siboonruang, Long Island City, NY, USA
Aditya Singla, West Lafayette, IN, USA
Jairus Dameanne Somera, Las Pinas City, Metro Manila, Philippines
Uirim Son, Gwangju, Jeollanam-do, South Korea
Katherine Steinberg, Cambridge, MA, USA
Dominik Steinle, Ulm, Baden-Wuerttemberg, Germany
Dominik Stepien, Ulm, BadenWuerttemberg, Germany
Kevin Stojanovski, Erlangen, Bavaria, Germany
Moritz Streb, Stockholm, Sodermanland and Uppland, Sweden
Luiza Streck, Munich, Bavaria, Germany
Po-Hua Su, Ulm, Baden-Wuerttemberg, Germany
Sho Sugawa, Kawasaki-shi, Kanagawa, Japan
Sylwia Sulikowski, North Barrington, IL, USA
Congcong Sun, Xi’an, Shaanxi Province, China
Fei Sun, Provo, UT, USA
Dola Sundeep, Kurnool, AP, India Gabor Szabo, South Bend, IN, USA
Hyun Jong Tak, Seoul, Gyeonggi-do, South Korea
Riku Takayama, Sendai, Miyagi, Japan
James Tan, Singapore, Singapore, Singapore
Weirui Tan, Amherst, MA, USA
Chaoyun Tang, Sunderland, MA, USA
Hunter Teel, Columbia, SC, USA
Prichard Tembo, Reno, NV, USA
Wai Than, Stillwater, OK, USA
Phung Thien, Jeonju, North Jeolla, South Korea
Susmi Thomas, Kallumala, KL, India
Tam Tran, Lafayette, LA, USA
Brandon Truong, North York, ON, Canada
Honesto Ovid Tubalinal, Quezon City, Metro Manila, Philippines
Rik van Gorp, Amsterdam, North Holland, Netherlands
Pierpaolo Vecchi, San Giovanni in Persiceto, Emilia-Romagna, Italy
Lorenzo Vergari, Berkeley, CA, USA
Ekta Verma, Bloomington, IN, USA
Vivienne Angelica Viernes, Quezon City, Metro Manila, Philippines
Jeremy Vives, Saint Martin d’Heres, Auvergne-Rhone-Alpes, France
Anyang Wang, Amherst, MA, USA
Cliffton Wang, Irvine, CA, USA
Dingtai Wang, Xi’an, Shaanxi Province, China
Han Wang, Blacksburg, VA, USA
Peng Wang, Tallahassee, FL, USA
Wanhua Wang, Columbia, SC, USA
Weining Wang, Atlanta, GA, USA
Yuxin Wang, Falcon Heights, MN, USA
Mohd Shaharyar Wani, Princeton, NJ, USA
Hugh Warkentin, Kingston, ON, Canada
Lingze Wei, Stanford, CA, USA
Di Wen, Wu Han, Hubei, China
Olivia Westhead, London, Greater London, UK
Enggar Wibowo, Berlin, Berlin, Germany
Haley Williams, Berkeley, CA, USA
Xinsheng Wu, Pittsburgh, PA, USA
Ao Xie, Auburn, AL, USA
Sijie Xie, Leuven, Flemish Brabant, Belgium
Kaixuan Xu, Nashville, TN, USA
Shi Xuncheng, Singapore, Singapore, Singapore
Siva Ram Yadavalli, Chennai, TN, India
Jian Yang, Kalamazoo, MI, USA
Minji Yang, Gwangju, Jeollanam-do, South Korea
Tzu Yi Yang, Hsinchu, Hsinchu County, Taiwan
Zicheng Yang, Quincy, MA, USA
David Yapell, Miami, FL, USA
Humaira Yeasmin, Auburn, AL, USA
Yijie Yin, Burlington, MA, USA
Eun Seong Yu, Asan, Chungcheongnam-do, South Korea
Mengjie Yu, Ann Arbor, MI, USA
Aseel Zeinati, Flemington, NJ, USA
Anni Zhang, Auburn, AL, USA
Liwen Zhang, Tullahoma, TN, USA
Meng Zhang, Xi’an, Shaanxi Province, China
Qi Zhang, Indianapolis, IN, USA
Yuxuan Zhang, West Lafayette, IN, USA
Fengyi Zhao, Atlanta, GA, USA
Bingyao Zhou, Portage, MI, USA
Guangqi Zhu, Indianapolis, IN, USA
On September 15, 2022, members of the Gebze Technical University (GTU) chapter welcomed ECS President Turgut M. Gür to the GTU campus for a full day visit. The GTU student chapter was established only recently in 2021 under the supervision and guidance of faculty advisor Associate Professor Aligül Büyükaksoy of the Department of Materials Science and Engineering at GTU. It enjoys a large membership of more than 15 students. Despite its short history, the chapter has been quite active, having organized two webinars by outside speakers and a face-to-face meeting with members during the pandemic.
During his visit, Prof. Gür met with members of the ECS student chapter, and had extensive conversations about the chapter’s goals and accomplishments, previous and future activities, and ways the chapter can work with ECS to improve the chapter’s activities and broaden impact. Prof. Gür encouraged students to engage with ECS more closely and to take on leadership roles in the Society. He also answered chapter members’ questions about ECS activities and educational opportunities overseas, and provided insights and advice on career planning and academic and professional life.
Prof. Gür gave a technical talk, “Pathways to Decarbonizing Electric Power Generation: Challenges and Opportunities for Energy Materials and Carbon Capture.’’ The seminar was well attended and attracted wide interest among faculty and students from many departments and fields. In his talk, Prof. Gür gave an outlook on the global energy landscape in the context of CO2 emissions, emphasized the importance of energy storage for wider deployment of renewables, and highlighted the magnitude of global challenges in mitigating climate change. The ECS Gebze Technical University Student Chapter gratefully thanks Prof. Gür for an inspiring day.
The ECS Indiana University Student Chapter continues recruiting new members and promoting The Electrochemical Society. This year, our members received multiple fellowships and awards. They attended national conferences to present their research, including the 2022 Inorganic Chemistry and Noble Metal Nanoparticles Gordon Conferences (GRC) and ACS Fall 2022. Chapter Secretary Krista M. Kulesa gave a talk at the Inorganic Chemistry GRC. Member Megan Knobloch presented a poster at the Noble Metal Nanoparticles GRC. Chapter President Sheyda Partovi and member Moumita Ghosh presented their current research at ACS Fall 2022. Moumita Ghosh was invited to attend the ACS Green Chemistry Summer School in Golden, CO, where she presented a poster about her research and learned about the importance of green and sustainability chemistry.
Chapter Advisor Prof. Jeremy Smith hosted Prof. Caroline Saouma from the University of Utah. Chapter members met with her to discuss their research and career aspirations. On September 26, 2022, the student chapter–selected speaker, Prof. Jillian Dempsey, presented “How Molecular Catalysts Mediate the Electrochemical
Generation of Fuels.” Members also discussed their research and future career directions with Prof. Dempsey. The ECS Indiana University Student Chapter is grateful for the opportunity to meet with several accomplished and knowledgeable electrochemists.
In August, the chapter met with incoming chemistry graduate students to present the chapter’s mission, describe past and future chapter activities, and recruit new members. New student chapter officers will be elected by year-end.
The ECS Jawaharlal Nehru University (JNU) Student Chapter at the Special Centre for Nanoscience held its second membership meeting. The chapter’s mission is to promote interest in and the advancement of the design and application of cutting-edge nanotechnology and nanoscience-based biosensors with interest in diagnostics among JNU students. Supportive faculty advisor Dr. Partima Solanki is head of the Nano-Bio Laboratory, Special Centre for Nanoscience, JNU, for biosensors and point-of-care devices. With enthusiastic efforts from President Amit K. Yadav, Vice President Reena Sajwan, Secretary Damini Verma, and Treasurer Navneet Chaudhary, the chapter will undoubtedly succeed in achieving the tremendous yet attainable vision it has for the organization. The chapter looks forward to finally building a coherent community of electrochemists at JNU, new relationships with other ECS Student Chapters, and partners outside the JNU community.
The founding chapter members demonstrated enthusiasm by hosting the 2nd Sensors for Society Symposium on October 12, 2022. Although the chapter has been active for only a few months, it was honored to host another speaker. Prof. P. K. Bhatnagar, formerly Head Electronic Scientist, Dean FIAS, UGC BSR Faculty Fellow, University of Delhi, presented “Typical Polymer-based Sensors and Nanosensors for VOC and UV Detection.” He received his PhD in 1980 from Delhi University. Before his retirement in February 2014, he was Professor and Head of the Department of Electronic Science twice at the University of Delhi South Campus. His research areas are optical memories, photovoltaics, application of nano materials in electronic devices, quantum dots, conducting polymers, ZnO nanorods, and DNA sequence detection using conducting polymers and carbon nanotubes. Prof. Bhatnagar introduced his work on the development and characterization of carbon nanotube and polymerbased composite volatile organic compound sensors. He also discussed mutation detection for medical diagnostics using FRET between cationic conjugated polymer and labeled PNA probes. He reviewed current technologies for sensors/biosensors in diagnostics
and future possibilities as well as limitations. A question-and-answer session facilitated the formulation of a general meeting summary.
Students attended from different JNU departments, including Physics, Materials Science, Chemistry, Biology, Nanoscience, and Nanoengineering. Students at JNU show great interest in electrochemistry, biosensors, biomarkers, diagnostics, and optical and point-of-care devices, and in The Electrochemical Society as a whole. The ECS Student Chapter support was the primary funding for the symposium, which was free for all attendees. Through the symposium, the chapter gained new members. Further, the chapter hopes to plan events for the upcoming academic school year and organize more symposia and seminars.
More information about the Nano-Bio Laboratory, SCNS, and JNU is available on our webpage
Hosting numerous academic, social, and informative events made 2022 one of the ECS University of Notre Dame Student Chapter’s most active years yet. On April 26, the chapter hosted its first-ever in-person seminar since it was founded in 2019, notably at the beginning of the global pandemic. The chapter had the pleasure of hosting Prof. Jordi Cabana from the University of Illinois Chicago, whose talk addressed the functionality of oxides in Mg batteries. After the seminar, Prof. Cabana continued interacting with the chapter community, visiting students’ laboratories, and sharing invaluable expertise and ideas on the design and structure of electrochemical cells for energy storage.
On August 25, the chapter provided the next highlight by hosting Prof. Csaba Janáky from the University of Szeged (Hungary) as our first in-person international speaker. His seminar talk focused on electrolyzer development and process optimization for industrial CO2 electroreduction. The chapter acknowledges Prof. Prashant Kamat for helping the chapter host this event, which not only extended members’ scientific horizons but also enriched cultural exchange.
On October 12, the chapter virtually hosted Prof. Andrew Wilson from the University of Louisville. An expert in the field of electrocatalysis and Chair of the ECS Mid-America Section, his talk addressed the role of plasmons in electrocatalysis. This topic resonated strongly with the research interests of the chapter’s community.
The chapter hosted several social events in June and September, interacting with new and existing members. While playing various games and enjoying free food and drinks, these events strengthened the network among chapter members. Students from the Department of Chemistry and Biochemistry and the Department of Chemical and Biochemical Engineering especially connected further and explored interdepartmental areas of science.
An officer transition occurred at the beginning of June.
The community officially welcomed President Julius Reitemeier, Vice-President Bo-An Chen, Secretary Lingyu Yang, and Treasurer Jishnudas Chakkamalayath as board members. The chapter welcomes Prof. Kaiyu Fu as a new Assistant Professor at the University of Notre Dame and the chapter’s new faculty advisor. The addition of his expertise underscores the steep growth of the electrochemical community at Notre Dame.
Fueled by the number of distinguished speakers and stimulating discussions at the seminars, the student chapter recorded a strong increase in members, counting 48 students to date, almost triple the number since the chapter was established. In the future, the chapter plans to strengthen the community further by hosting electrochemical workshops and outreach events, aiming to exchange valuable electrochemical skillsets, and to provide a suitable platform for students to build relationships and interactions with leaders in electrochemical science.
• Indian Institute of Technology Madras, India
• Johns Hopkins University, US
• University of Buffalo, US
• University of Colorado Boulder, US
• University of the Philippines, Philippines
• Xidian University, China
ECS Student Chapter membership provides many benefits, including:
• Engaging with students and peers
• Organizing technical meeting programs and scholarly activities
• Collaborating with members to present posters at ECS biannual meetings
• Networking with 8,000+ international ECS members
• Accessing career resources
• Adding impressive extracurricular activities to resumes
• Funding to support chapter activities
• Partnering with local ECS sections on activities and technical programs
• Receiving recognition in Interface and the ECS website
Visit the ECS Student Center for more information about student chapters. For the global scope of the Society’s student chapter network, view the Student Chapter Directory
Interested in establishing an ECS Student Chapter at your academic institution? Review the guidelines for starting a chapter and submit a new student chapter application today!
Please join us in celebrating the accomplishments of our community’s graduates. The list below announces ECS members who graduated from January 1 through December 31, 2022.* Join us in congratulating them on their academic success—and best wishes for their next career steps!
Dhilip Kanna A.
Pedram Abbasi
George Paa Kwesi AffaduDanful
Brahim Ahammou
Amir Alihosseinzadeh
Eva Michelle Allen
Eleni Andreou
Tanvir Alam Arman
Fabusuyi Akindele Aroge
Arezoo Avid
Yasuhiro Ayauchi
Irfan Aydogdu
Jenny Börjesson Axén
Behrouz Bahadormanesh
Aaron Thomas Bain
Anne Berger
Amruth Bhargav
Sahana Bhattacharyya
Md Sajibul Alam Bhuyan
Thomas Boulanger
David Emory Brown
Silje Nornes Bryntesen
Cailin Buchanan
Derrick James Butler
Selma Nur Buyukgoz
Kiana Cahue
Lea Caradant
Adam Timothy Caridi
Ezer Castillo
Utkarsh Chadha
Nora Chelfouh
Yuhan Chen
Darren Chow
Charlotte Clegg
Keith Cleland
Jan-Willem Clerix
Estefania Godoy Colin
Monica Susana Campos
Covarrubias
Kenneth Crossley
Suman Das
Vikram Narayanan Dhamu
Jan-Carlo Díaz
Nataša Diklić
Prashant Dubey
Austin Duncan
Erica Eggleton
Eugene Tettey Engmann
Matthias Felix Ernst
Vanessa Briana Espinoza
Selma Fairach
Conner Fear
Alexis Maguin Fenton Jr.
Margaret Anne Fitzgerald
Sean Foradori
Juan David Forero-Saboya
Julie C. Fornaciari
Marvin Frauenrath
Mathias Fritz
Mayur Mahendra Gaikwad
Anindita Ganguly
Stephanie Gao
Tanja Geng
Adjmal Ghaur
Ali Ghazanfar
Touraj Ghaznavi
Gerald Gilligan
Ana Maria Ulloa Gomez
Matt Gordon Navneet Goswami
Maximilian Graf
Raymond Guan Disni Gunasekera
Youngjin Ham
Heather Hamilton
Louis Hartmann
Gillian Hawes
Maren-Kathrin Heubach
Degenhart Hochfilzer
Pia Hoenicke
Christin Hogrefe
Singyuk Hou Zakaria Hsain
Jake Huang
Keyvan Jodeiri Iran
Samuel T. Jacobs
Cristian Jaimes
Alexander Jameson
Vijay Kumar Jayswal
Rajesh Bharat Jethwa
Matthew Jordan Kätlin Kaare
Gautham Kumar Kabbinahithlu
Venkatesh Kabra
Magdalena Kaliszczak
Rumasha Nipuni Thiruwana Kankanamage
Masaki Kataoka
Kenta Kawashime
Bahman Khaki
Abdelrahman Mohamed Mokhtar Khaled
Kishwar Khan
Chanho Kim
David Kitsche
Bennet Kleinhans
Aswathi Koorikkat
Martin Kosicek
Isaac A. Kretzmer
Krista M. Kulesa
Deepak Kumar
Steven Lam
Egle Latvyte
Michael J. Lee
Michelle Lehmann
Yuting Lei
Selma Leonardi
Justine Lespiaux
Tyra Lewis Feng Li
Jiawei Li Katja Li Xin Li
Yuanjiao Li Allen Yu-Lun Liang
Jiri Libich
Jeonghoon Lim
Shuyu Lin
Chang Liu
Christopher Pantayatiwong Liu
Alice Victoria Llewellyn
Joseph Steven Lopata Xinlin Lu
Kyle Ludwig Samantha Luong
Diana Marie Lutz
Norman Luu
John Alexander H. MacDonald Shaimaa Maher
Vaishali Maheshkar
Tanushree Mandal
Refiloe Innocencia Maphoto
Gilberto Josué Martínez-Blanco
Eric McKenzie Hunter McRay Rohit Mehta
Nathaniel Metzger
Alexandra Meyer
Harry Michael
Philip Minnmann
Janhavi Mishra
Jun-yeob Moon Carolina Vicente Moraes
James Thomas Moulton Zamaan Mukadam
James Murawski
Jessica Murdzek
Paranjothi Murugan Deeksha N. V. N. Nomaan Nabi
Tan Nguyen
Robert Nissler
Cassandra Nunez
Tsubasa Okamura
Mohamed Okasha
Tonny Ipael Okedi
Ryota Okuno
Renee Olney
Adekunle James Omoboye
Gerrit Overhoff
Maedeh Pahlevaninezhad
Dhananjai Pangotra
Daniel Lee Parr, IV
Nigel Patterson
Emmanuel Peprah-Yamoah
Vanesa Muñoz Perales
Verena Perner
Saran Pidaparthy
Sofia Pinzon
Yves Preibisch
Simon Qian
Yitao Qiu
Haozheng Qu
Sonja Radloff
Syed Rafiquddin
Sundararajan Ramakrishnan
Ruchiranga Ranaweera
Philip Rapp
Nallayagari Ashwini Reddy
Saketh Srikar Reddy
Davide Ripepi
Nuwanthi Dilhari Rodrigo Chushan Ru
Matthew Sadd
Tamoghna Saha
Sadman Sakib
Susmita Sarkar
Rodrigo Elizalde Segovia Asmita Shah
Nora Shaheen
Shailendra Kumar Sharma
Katey Sheets
Sarah Sheffield
Pranay Shrestha
Qingdian Shu
Humera Khatoon Siddiqui Mayura Sankalpa Silva
Shashwat Singh
Rebecca Joan Skelton
Arthur Douglas Sloan
Piper Smith
Dhaivat Jayendrabhai Solanki
Marta Cazorla Soult
Stephanie Spence
Michael Spencer
Thomas Stephen Stracensky
Benjamin Strehle
Yunkai Sun
Yoshimasa Suzuki
Li Tao
Kevin Tenny
Daisy Thornton
Anna Iwona Tomaszewska
Ouardia Touag
Tam Thi Thanh Tran
Zarina Turtayeva
Veenasri Vallem
Anjaliben Harsukhbhai
Vanpariya
Julia Fernández Vidal
Niraj Ashutosh Vidwans
Huiru Wang
Konosuke Watanabe
Julia Wellmann
Zachary Xavier William Widel
Lea R. Winter
Zhixiao Xu
Jian Yang
Chentian Yuan
Zahid Ali Zafar
Shumin Zhang
Yirui Zhang
Yiwei Zheng
Yu Zheng
Bingyao Zhou
* Graduation information as of October 1, 2022. Members must list their graduation date in their ECS My Account to be included in the list.
•
•
•
•
•
•
•
•
•
•
•
•
•
•
2022
BioLogic USA/BioLogic SAS (14*)
Duracell (65)
Gamry Instruments (15)
Gelest, Inc. (13)
Hydro-Québec (15)
Pine Research Instrumentation (16)
BASi (7)
Central Electrochemical Research Institute (29)
Center for Synthetic Organic Electrochemistry, University of Utah (1)
DLR-Institut für Vernetzte Energiesysteme e.V. (14)
EL-CELL GmbH (8)
Electrosynthesis Company, Inc. (26)
Ford Motor Corporation (8)
GS Yuasa International Ltd. (42)
Honda R&D Co., Ltd. (15)
Medtronic Inc. (42)
Nissan Motor Co., Ltd. (15)
Pacific Northwest National Laboratory (PNNL) (3)
Panasonic Energy Corporation (27)
Permascand AB (19)
Plug Power, Inc. (1)
Teledyne Energy Systems, Inc. (23)
UL Research Institutes (1)
Center for Solar Energy and Hydrogen Research Baden-Württemberg (ZSW) (18)
Energizer (77)
Faraday Technology, Inc. (16)
GE Global Research Center (70)
Lawrence Berkeley National Laboratory (18)
Scribner Associates, Inc. (26)
Toyota Research Institute of North America (14)
Advanced Cell Engineering (1) Cummins, Inc (4)
General Motors Holdings LLC (70)
Giner, Inc./GES (36)
Ion Power Inc. (8)
Kanto Chemical Co., Inc. (10)
Los Alamos National Laboratory (14)
Metrohm USA, Inc. (9)
Microsoft Corporation (5)
Occidental Chemical Corporation (80)
Sandia National Laboratories (46)
Sherwin-Williams (1)
Technic, Inc. (26)
United Mineral & Chemical Corporation (1)
Western Digital GK (8) Westlake (27)
Yeager Center for Electrochemical Sciences (24)
ECS, a prestigious nonprofit professional society, has led the world in electrochemistry, solid state science and technology and allied subjects since 1902, providing a rigorous and high-quality home for the whole community.
ECS is dedicated to moving science forward by empowering researchers globally to leave their mark on science. The Society connects a diverse and representative constituency of members and nonmembers to accelerate scientific discovery, facilitate the engagement of an inclusive network, and champion the dissemination of research to support a sustainable future.
For more information on becoming a member, or publishing in ECS publications, visit electrochem.org