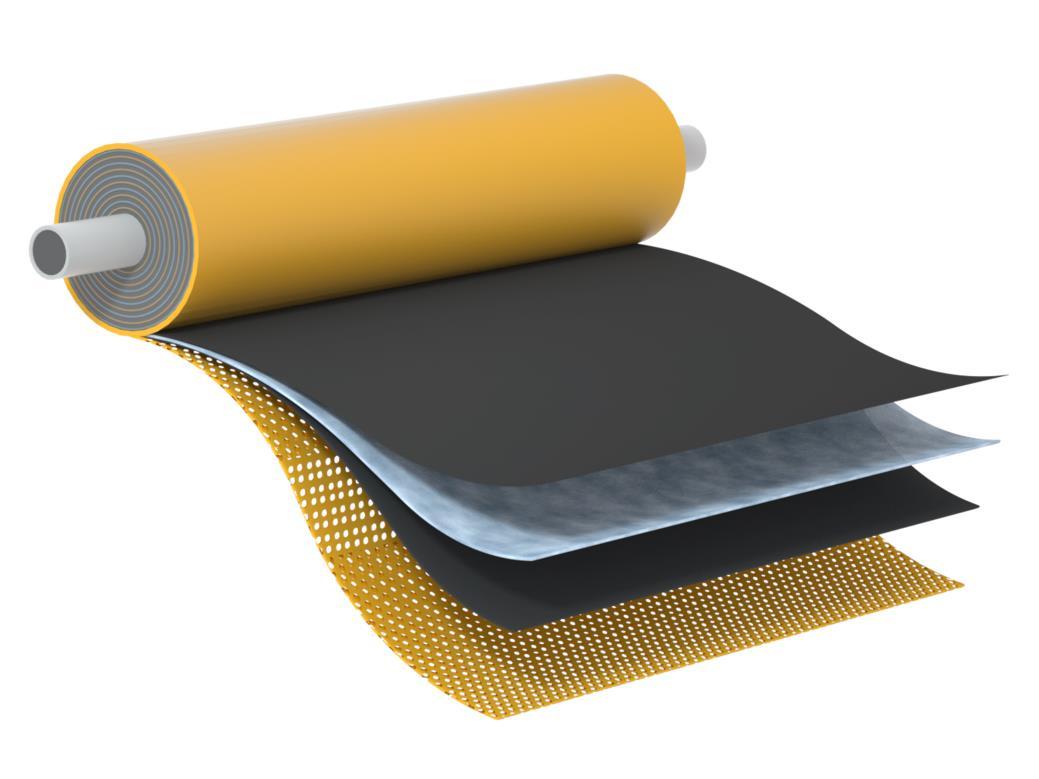
14 A Sneak Peek at the 245th Meeting
26 Heavy-Duty Transportation in 10 Charts
49 Electrolysis in Chloride Molten Salts for Sustainable Critical Metals Production and Recovery
55 Electrochemical Gas Separation for Green Energy Integration
14 A Sneak Peek at the 245th Meeting
26 Heavy-Duty Transportation in 10 Charts
49 Electrolysis in Chloride Molten Salts for Sustainable Critical Metals Production and Recovery
55 Electrochemical Gas Separation for Green Energy Integration
ECS, a prestigious nonprofit professional society, has led the world in electrochemistry, solid state science and technology and allied subjects since 1902, providing a rigorous and high-quality home for the whole community.
ECS is dedicated to moving science forward by empowering researchers globally to leave their mark on science. The Society connects a diverse and representative constituency of members and nonmembers to accelerate scientific discovery, facilitate the engagement of an inclusive network, and champion the dissemination of research to support a sustainable future.
For more information on becoming a member, or publishing in ECS publications, visit electrochem.org
We’ll see you at the 245th ECS Meeting May 26th through May 30th and ECS PRiME October 6th through October 11th.
LEADING ENERGY CONVERSION AND STORAGE TECHNOLOGIES SINCE 1980
Fuel Cell Test | Electrolyzer Test | Membrane Conductivity Test | Redox Flow Cell Test
Continuity is a goal for all organizations. Successful organizations are built in such a way that their excellence is maintained over the long term, from generation to generation. ECS is a great example; its divisional and editorial board structures have provided robust mechanisms for continuity for over 120 years. A succession plan (or lack thereof) is so key that TV series have been made about it (one with the ingenious title Succession). Organizations that are successful over multiple generations are those that can handle such planning well, whether by luck or by science. Organizations that do not handle this aspect well may be spectacular while their founder/star is involved, but the Grim Reaper remains undefeated. At that point, the organization drifts and eventually fades away. I am sure you can think of a couple that fit the latter description.
In academia, there is another layer of intrigue which is the requirement that a department’s faculty and dean of the school agree on how to “replace” someone who is retiring. In the US, there is no mandatory retirement age, so succession planning can be a little uncomfortable for all involved. Seniority carries a lot of weight, whether it should or not, and department chairs generally avoid conversations like, “So, Mike, we have been colleagues for 25 years. Not to seem like we are putting you out to pasture, but if we did, what kind of pasture would be your favorite? And when can you pack up your office?” Faculty themselves can be grumpy about the topic as well (grumpy faculty, shocking, I know). We are in the enviable position of being paid to do something we love (generally). Why should we leave? In most cases, faculty want the department to continue to have active research in their area as it is the most important topic anyone could work on (obviously). But faculty positions are like gold. As I have noted in previous columns, in the research arena, departments are like groups of independent small businesses. Thus, when a faculty position opens there is often intense jockeying to either maintain an area of research, strengthen a different area, move the department into a new research direction, or to move the position to another department at the dean’s fancy.
My department has been thinking about this topic a lot because we have faculty who make up our Silver Seven, as I lovingly call my six colleagues and me. We are faculty that (trying to be polite here) are a lot closer to the end than the beginning of our careers to put it mildly. Our corrosion/electrochemistry group is well represented in that seven. The ongoing importance of our fields, the established infrastructure, and the health of the research enterprise here were compelling arguments for hiring replacements in the same general area, although the $50 bribes paid to some faculty probably helped. Glenn Stoner established our group 50 years ago, and he implemented a succession plan, one that would have been successful if only it hadn’t included John Scully and me. Part of that plan was to expand the research focus from only applied electrochemistry to corrosion. Now, 30 years later, we need to consider what’s next, although we have the help of Beth Opila and her outstanding high-temperature oxidation work and reputation. It is a daunting task, but an exciting one as well. The position is using a broad definition of “harsh environments” which will complicate the selection, but also provide the most opportunities for the next generation of research in our group.
By the time you read this missive, we should be well into the process, I expect/hope. One tenet of any hiring is that one should “hire better than you are.” The cruel among you (looking at you, E. J. Taylor) are already thinking how easy that would be in my case. We also believe that some overlap between when the new faculty member starts and when the mature faculty exits has tremendous benefits to the new faculty member’s academic career. Overlap requires good luck and a supportive department chair, faculty, and dean. Those seem to be in place here. That said, we are keeping our fingers crossed (which makes typing a lot harder), because as the great philosopher and heavyweight boxer Mike Tyson said, “Everybody has a plan until you get punched in the face.” Wish us luck.
Until next time, be safe and happy.
Published by:
The Electrochemical Society (ECS) 65 South Main Street Pennington, NJ 08534-2839, USA Tel 609.737.1902, Fax 609.737.2743 www.electrochem.org
Editor: Rob Kelly
Guest Editors: Christopher G. Arges and Hui Xu
Contributing Editors: Christopher L. Alexander, Christopher G. Arges, Scott Cushing, Ahmet Kusolgu, Donald Pile, Alice Suroviec
Director of Publications: Adrian Plummer
Director of Community Engagement: Shannon Reed
Production Editor: Kara McArthur
Graphic Design & Print Production Manager: Dinia Agrawala
Staff Contributors: Frances Chaves, Genevieve Goldy, Mary Hojlo, Christopher J. Jannuzzi, John Lewis, Anna Olsen, Jennifer Ortiz, Francesca Di Palo, Jennifer Tarantino, JaneAnn Wormann, Fern Oram
Advisory Board: Brett Lucht (Battery Division)
Dev Chidambaram (Corrosion Division) Uroš Cvelbar (Dielectric Science and Technology Division)
Luca Magagnin (Electrodeposition Division)
Qiliang Li (Electronics and Photonics Division) Katherine Ayers (Energy Technology Division)
Cortney Kreller (High-Temperature Energy, Materials, & Processes Division)
Maria Inman (Industrial Electrochemistry and Electrochemical Engineering Division)
Eugeniusz Zych (Luminescence and Display Materials Division)
Jeff Blackburn (Nanocarbons Division)
Shelley Minteer (Organic and Biological Electrochemistry Division)
Stephen Paddison (Physical and Analytical Electrochemistry Division)
Larry Nagahara (Sensor Division)
Publications Subcommittee Chair: James Fenton
Society Officers: Gerardine Botte, President; Colm O’Dwyer, Senior Vice President; James (Jim) Fenton, 2nd Vice President; Francis D’Souza, 3rd Vice President; Marca Doeff, Secretary; Elizabeth J. Podlaha-Murphy, Treasurer; Christopher J. Jannuzzi, Executive Director & CEO
Statements and opinions given in The Electrochemical Society Interface are those of the contributors, and ECS assumes no responsibility for them.
Authorization to photocopy any article for internal or personal use beyond the fair use provisions of the Copyright Act of 1976 is granted by The Electrochemical Society to libraries and other users registered with the Copyright Clearance Center (CCC). Copying for other than internal or personal use without express permission of ECS is prohibited. The CCC Code for The Electrochemical Society Interface is 1064-8208/92.
ISSN : Print: 1064-8208 Online: 1944-8783
The Electrochemical Society Interface is published quarterly by The Electrochemical Society (ECS), at 65 South Main Street, Pennington, NJ 08534-2839 USA. Subscription to members is part of membership service. © Copyright 2024 by The Electrochemical Society. *“Save as otherwise expressly stated.”
The Electrochemical Society is an educational, nonprofit 501(c)(3) organization with more than 8,500 scientists and engineers in over 75 countries worldwide who hold individual membership. Founded in 1902, the Society has a long tradition in advancing the theory and practice of electrochemical and solid state science by dissemination of information through its publications and international meetings.
https://orcid.org/0000-0002-7354-0978
41 26
by Ahmet Kusoglu42
by Christopher G. Arges and Hui Xu
The
Christopher49
G. Arges Electrolysis
55
by Ben Holcombe, Nicholas Sinclair, Alexander Baker, Eunjeong Kim, Scott K. McCall, and Rohan Akolkar
Electrochemical
byThomas Stracensky and Hui Xu
3
7
10
14
17
33
ello friends! Welcome to the first Interface edition of the new year.
As I write, we have just finished reviewing the initial round of 245th ECS Meeting abstract submissions. As we have seen with every meeting since the COVID “blip,” the ECS community continues to grow, attracting new and more diverse members, reaching new constituents, and expanding the products, programs, and services we offer in support of ECS’s mission. With more than 3,000 manuscripts accepted already (even before accepting late submissions!), the 245th ECS Meeting from May 26th to 30th in San Francisco will be our largest spring meeting, breaking the record set in Boston last spring.
This is an incredibly encouraging and inspiring indication of the value the larger technical community places on engaging with ECS, especially considering the tumultuous times and myriad disruptions we navigated in recent years. It is wonderful to see that ECS is stronger for what we have endured together.
We have great momentum behind us, and I would like to discuss efforts we have launched to build on that momentum and advance two initiatives ECS President Gerardine Botte outlined in the previous edition of Interface—Sustainability and Diversity.
On the Sustainability front, we are helping advance green transportation technologies and address a critical labor shortage in the battery industry. To that end, I am excited to announce that later this year we will launch our new distance learning platform and release our first suite of long-form online courses to provide workforce development training for the battery industry. The courses are:
• Components for Lithium Ion Batteries: Electrolytes, Anodes, and Cathodes: Participants develop a general understanding of the properties and challenges associated with different commercial electrode materials and electrolytes and learn about materials characterization techniques for electrode materials and electrolytes.
• Electrochemical Techniques and Diagnostics for Batteries: Basic electrochemical testing techniques and diagnostic methods for commercial lithium ion batteries are covered.
• Fabrication of Batteries and Pack Design: General methods for the preparation of laminate electrodes, electrode stacking/winding, and pack construction are reviewed. Participants also develop a general understanding of commercial laminate electrode preparation and the challenges associated with electrode stacking and winding processes.
Each course is a self-directed, online learning experience, featuring 20–25 hours of instruction, with various levels of assessment given throughout the course. The initial set of courses is geared to those with existing technical expertise in an adjacent technology (materials science, physics, chemistry,… etc.) who want to re-skill for work in the burgeoning battery industry.
The teams developing these courses have worked hard since early last summer to create the content and assessments. Concurrently, ECS staff partnered with instructional design experts to launch ECS’s first-ever Learning Management System (LMS), which will integrate seamlessly with the ECS website and the accounts of ECS members and constituents enrolled in these courses. Stay on the lookout for more information on the platform’s beta launch later this spring.
To help meet our Diversity goals, the “Redefining ECS Membership” project was launched last year. The ECS Board of Directors is working with the Individual Membership Committee chaired by E. J. Taylor to develop new, lowercost options for joining the ECS community. The team’s final recommendations will be submitted for review by the Board in advance of the May meeting. In the meantime, some great news: ECS membership has now grown beyond pre-pandemic levels! “Redefining ECS Membership” will extend that growth trajectory, finding new audiences by lowering barriers to engagement with the Society.
An important aspect of our Diversity effort is establishing new ECS Student Chapters to help develop and nurture the new generation of scientists and researchers in our field. We have already experienced phenomenal results through this effort. A new chapter recently launched at the Universidad Autónoma de Nuevo León in Mexico, joining new chapters at universities in India, Singapore, Thailand, and the Republic of Korea. With these new additions, ECS has more than 130 student chapters around the globe!
And of course, we look forward to seeing you at our meetings in 2024. The spring meeting in San Francisco is already shaping up to be an amazing event. In October, we convene PRiME 2024 with our partners, The Electrochemical Society of Japan (ECSJ) and The Korean Electrochemical Society (KECS), in person in Hawaii for the first time in eight years! Since we last met there in person in 2016, our field and respective societies have experienced incredible growth. The 2024 conference promises to be the largest single gathering in our 122-year history. Don’t miss being part of this historic event! Click here to learn more and submit your work before the April 12th PRiME 2024 abstract submission deadline.
Last, I want to extend my thanks and gratitude to the entire ECS community. The ECS HQ team and I are incredibly honored to serve this organization. We look forward to joining with you in 2024 and beyond to advance the ECS mission around the world!
See you soon!
For data-driven decisions you can trust
OBSERVATION EXPLANATION SOLUTION
The system is not linear
Decrease the perturbation amplitude of the input signal
The system is not stationary
Increase the rest period before the EIS measurement
The output signal is too small
Increase the perturbation amplitude of the input signal
Electrochemical Impedance Spectroscopy provides valuable data on redox processes, which can lead to significant advances in science and industry.
However, acquiring this data can be complex, even for seasoned experts. EIS QI helps you to find the delicate balance between linearity (THD) and noise (NSR) and ensures you know exactly how long to wait for a stable system (NSD).
Biologic’s quality indicators streamline and validate impedance experiments, ensuring you get the data you need.
Only BioLogic allows you to get quantitative feedback for your Electrochemical Impedance Spectroscopy data. QI
Our members’ crucial role in addressing global challenges such as climate change, renewable energy, connectivity, and pandemics became increasingly clear in 2023. As the leading society in solid state and electrochemical science, we take pride in fulfilling our urgent mission and in empowering members at the forefront of discovery amid ongoing global uncertainties. Key 2023 achievements include:
• Convening scientists at all career stages— from academia, industry, and governmental organizations—at ECS meetings designed to drive research, discovery, and collaboration;
• Promoting global awareness of our science and technology;
• Supporting and nurturing future leaders and recognizing rising stars in electrochemical and solid state science via ECS Student Chapters and awards;
• Expanding ECS’s global membership;
• Expanding traditional journal offerings and fostering open access for faster scientific progress;
• Strengthening diversity and inclusion through the ECS Presidential Ad Hoc Committee on Diversity, Equity, Inclusion, and Engagement;
• Increasing our international presence post-pandemic with meetings in Europe and leadership visits to Asia and Mexico; and
• Achieving strong financial results to sustain these initiatives.
We invite you to join us in 2024 for the 245th ECS Meeting in San Francisco (May 26–30), and PRiME 2024 in Honolulu (October 6–11), and submit your work to the ECS Family of Journals, which have been delivering peer-reviewed excellence since 1902.
• More than 8,000 members strong and a return to pre-pandemic membership levels.
• The 2,690 student members is the largest number of student members ever!
• Representing 13 new Student Chapters in India, Mexico, Republic of Korea, Singapore, Thailand, and the United States, these students now bring their passion for the Society and electrochemistry and solid state science to their local student bodies.
+12.2%
• The 2023 ECS Class of Fellows welcomed 15 new members: Martin Bazant, Jeffrey Blackburn, Teruhisa Horita, Ajit Khosla, Po-Tsun Liu, Robert Mantz, Nazario Martín, John Muldoon, Mikael Östling, Bryan Pivovar, Minhua Shao, Peter Strasser, Alice Suroviec, Chunsheng Wang, and Bilge Yildiz
• With the addition of two 2023–2024 ECS Toyota Young Investigator Fellows, the Society’s partnership with the Toyota Research Institute of North America has, since its inception, provided more than $1.5M in research funding to 30 young investigators.
• The ECS Mid-America Section reestablished the Ernest B. Yeager Electrochemistry Award (previously offered by the ECS Cleveland Section), recognizing significant contributions to fundamental electrochemical science and engineering and distinguished careers as educators/researchers in Indiana, Illinois, Ohio, and Kentucky. 2023 2023 2023 2023
• Linda L. Horton, Associate Director of Science for Basic Energy Sciences (BES), US Department of Energy (DOE) Office of Science (SC), delivered the ECS Lecture at the meeting’s plenary session.
• For the first time since 2011, the 243rd ECS Meeting was co-located with SOFC-XVIII. Their day-long plenary session featured talks by David Tew, ARPA-E; William Gibbons, DOE EERE; Shailesh Vora, DOE; Mirela Atanasiu, Clean Hydrogen Partnership; Yosuke Fujii, NEDO; and Rak-Hyun Song, KIER.
• Zhenan Bao, K. K. Lee Professor of Chemical Engineering, Stanford University, delivered the ECS Lecture at the meeting’s plenary session.
• A special seminar, Young Researchers in Europe, highlighted the work of up-and-coming scientists, provided funding information, and fostered crossborder collaboration.
• Meeting attendees showed off their poetry, singing, and performance chops at a new and unique event, It Could be Verse: An Evening of Poetry and More
• Eliminated barriers to scientific progress by doubling transformative and read-and-publish agreements globally, enabling authors to access content behind paywalls and publish open-access without fees.
• Expanded our pool of qualified peer reviewers through training and education opportunities and designed new ways to encourage authors to choose ECS as their publication home.
• Attracted new reviewers dedicated to the ECS mission, equipped with ECS and IOP Peer Review Excellence training and certification.
• Maintained a digital library with record-breaking download and readership metrics.
The ECS Continuing Education program continues to grow to meet and support the needs of the community, offering webinars, short courses, and professional development opportunities. ECS also announced efforts to launch battery workforce development coursework, focused on three initial courses set to launch in 2024:
• Components for Lithium Ion Batteries
• Electrochemical Techniques and Diagnostics for Batteries
• Fabrication of Batteries and Pack Design
Articles and abstracts downloaded from the ECS Digital Library: 9,015,249
Journal articles published in the 2023 volume year: 1,763
Total Short Course registrants: 79
Percentage of articles published open access in 2023: 35%
# of open access papers published: 614
Webinars: 10
Participants: 5,274
Speakers: 10
Short Courses: 4
Professional development workshops: 7
Professional development workshop registrants: 325
oin your peers at this international conference where scientists, engineers, and researchers from academia, industry, and government laboratories gather at this unique interdisciplinary forum to share electrochemical and solid state science and technology research and issues. Absorb and exchange information on the latest scientific developments and related topics through oral presentations, poster sessions, panel discussions, tutorial sessions, short courses, professional development workshops, exhibits, and more!
Start planning now for these technical and networking opportunities:
• Five days of technical programming across 48 symposia;
• More than 3,000 abstracts;
• More than 2,250 oral presentations, including 575 invited talks by the world’s leading experts;
• More than 700 posters over three evening poster sessions;
• 14 hours of exhibit hall time over three days;
• Daily morning and afternoon coffee breaks;
• Complimentary WiFi in meeting rooms;
• Special program for nontechnical registrants.
San Francisco captivates visitors with its unique blend of iconic landmarks, cultural richness, and natural beauty. This vibrant and diverse city is renowned for its distinct neighborhoods, each with its own charm and character. From Chinatown’s historic streets to Union Square’s trendy boutiques, the city provides a wide range of experiences. Visitors can wander through Golden Gate Park’s scenic landscapes or explore notorious Alcatraz Island. San Fran’s culinary scene is also a major draw, with a plethora of world-class restaurants showcasing a variety of cuisines. Whether it’s the iconic cable cars, vibrant arts scene, or welcoming atmosphere, San Francisco promises travelers memorable and enriching experiences.
Monday, May 27
“What MXenes Can Do for Efficient Generation, Storage, and Conversion of Electrical Energy”
Yury Gogotsi, A. J. Drexel Nanomaterials Institute and Department of Materials Science and Engineering, Drexel University
Yury Gogotsi is Distinguished University Professor and Charles T. and Ruth M. Bach Endowed Chair in the Department of Materials Science and Engineering at Drexel University. Together with his students and colleagues, he has made principal contributions to the development of materials for electrochemical capacitors and other energy storage devices, discovered MXenes and polygonal nanotubes (graphite polyhedral crystals), demonstrated the tuning of structure and porosity of carbide-derived carbons, and developed new processes for the synthesis, surface modification, and purification of nanotubes and nanodiamonds. He also serves as Director of the A. J. Drexel Nanomaterials Institute. He received his MS (1984) and PhD (1986) from Kyiv Polytechnic and a DSc degree from the National Academy of Sciences of Ukraine in 1995. Prof. Gogotsi is a Fellow of the Electrochemical Society. With an h-index of over 200, he has been recognized as a Highly Cited Researcher in Materials Science and Chemistry, and a Citations Laureate in Physics by Clarivate Analytics (Web of Science). His lecture will describe the synthesis, structure, and chemistry of 2D carbides and nitrides known as MXenes, as well as their electrochemical applications, with a focus on the generation, storage, and conversion of electrical energy.
(Consult the meeting app for times.)
Society Award-Winning Speakers
Uziel Landau, Case Western Reserve University
Henry B. Linford Award for Distinguished Teaching
Daniel Lincot, Centre national de la recherche scientifique (CNRS)
Vittorio de Nora Award
Division Award-Winning Speakers
Xiang Gao, University of North Carolina at Charlotte Battery Division Postdoctoral Associate Research Award sponsored by MTI Corporation and the Jiang Family Foundation
Peter Mascher, McMaster University Dielectric Science & Technology Thomas Callinan Award
Travis Anderson, US Naval Research Laboratory Electronics and Photonics Division Award
Noor Ul Hassan, National Renewable Energy Laboratory
Energy Technology Division Graduate Student Award sponsored by BioLogic
Maha Yusuf, Princeton University
Energy Technology Division Graduate Student Award sponsored by BioLogic
William Mustain, University of South Carolina Energy Technology Division Research Award
Nikolay Kornienko, Institut für Anorganische Chemie, Universität Bonn Energy Technology Division Supramaniam Srinivasan Young Investigator Award
Yirui Zhang, Stanford University
Industrial Electrochemistry and Electrochemical Engineering
Division H. H. Dow Memorial Student Achievement Award
Deepra Bhattacharya, GE Vernova
Industrial Electrochemistry and Electrochemical Engineering
Division Student Achievement Award
R. Bruce Weisman, Rice University
Nanocarbons Division Richard E. Smalley Research Award
Delphine Bouilly, Université de Montréal Nanocarbons Division SES Research Young Investigator Award
Stefano Cinti, Università degli Studi di Napoli Federico II Sensor Division Early Career Award
Yaoli Zhao, University at Buffalo Sensor Division Student Research Award
Sunday, May 26
ECS Short Courses are all-day classes designed to provide students and seasoned professionals with in-depth education on a wide range of topics. These small classes taught by academic and industry experts offer personalized instruction and help novices and experts advance their technical expertise and knowledge.
An Integrated Approach to Chemical Mechanical Planarization Technology Advances
Basim Bahar and Jason Keleher, Instructors
Basic Impedance Spectroscopy
Mark Orazem, Instructor
Fundamental and Modern Technological Aspects of Electrodeposition for Material Processing Sudipta Roy, Instructor
Introduction to Lithium Ion Batteries
David Hall and Chock Karuppaiah, Instructors
(Check the meeting app for times.)
ECS provides professional development opportunities at its biannual meetings—including workshops and professional panels as well as career resources. They are targeted to students, early career researchers, and experienced professionals.
Mentoring Session
Facilitator: Alice Suroviec, Berry College
Peer Reviewer Excellence Certification Workshop
Instructors: Paul Cooper, The Electrochemical Society, and Jessica MacDonald, IOP Publishing
Essential Elements for Employment Success
Instructor: Michel Fouré, Berkeley Grant Writing, LLC
Resume Review
Instructor: Michel Fouré, Berkeley Grant Writing, LLC
Strategic Tools for a Successful Career
Instructor: Michel Fouré, Berkeley Grant Writing, LLC
Win Funding: How to Write a Competitive Proposal
Instructor: Michel Fouré, Berkeley Grant Writing, LLC
(Consult the meeting app for times.)
Opening Reception
Get a taste of San Francisco and kick off an exciting week! All attendees are welcome to enjoy light snacks, open bar, ample networking time, and a chance to meet with ECS division members.
ECS members are invited to join us for food, drinks, giveaways, and light entertainment. Jump-start the meeting with ECS community members before the Opening Reception. Register now, as only 400 tickets are available!
Wrap up the meeting’s first full day with friends and peers. Students and early-career professionals mingle in a relaxed setting and enjoy light hors d’oeuvres and refreshments.
Annual Society Business Meeting and Luncheon*
Join us as to celebrate 2023’s many successes and look ahead to an even brighter future!
With hundreds of posters to explore, don’t miss a minute of these sessions. Grab a snack, wander the aisles, review presentations, talk to authors, and get to know our exhibitors. These sessions are a great way to end the day!
Take time to explore electrochemistry and solid science’s leading vendors’ exhibits. Then stop by the exhibit hall for Poster Sessions, Networking Breaks, complimentary Professional Portraits, and the ECS Booth.
Many ECS divisions and meeting symposia host social events (receptions, banquets, luncheons, and more!) throughout the week. Be sure to check the online program for these opportunities to socialize and network with your peers!
*These events require pre-registration or purchase of a separate ticket.
A Batteries and Energy Storage
A01 New Approaches and Advances in Electrochemical Energy Systems: In Memory of Sri Narayan
Ayyakkannu Manivannan, Brett L. Lucht, Loraine Torres Castro, Chockkalingam Karuppaiah, Ratnakumar V. Bugga Energy Technology Division; Battery Division; Industrial Electrochemistry and Electrochemical Engineering Division
A02 Lithium Ion Batteries
Kang Xu, Jie Xiao, Shirley Meng
Battery Division
A03 Large Scale Energy Storage 15
Daniel Steingart, Joshua Gallaway, Nian Liu, Jacob Spendelow, Ertan Agar
Battery Division; Energy Technology Division
A04 Battery Material Scale-up and Manufacturing
Dongping Lu, Neil Dasgupta, Jagjit Nanda, Fang Dai Battery Division
A05 Battery Student Slam 8
Feng Lin, Chixia Tian Battery Division
B Carbon Nanostructures and Devices
B01 Carbon Nanostructures for Energy Conversion and Storage
Andrew Ferguson, Kyu-Young Park, Uroš Cvelbar, Jeff L. Blackburn, Avetik Harutyunyan, Chunsheng Wang
Nanocarbons Division; Battery Division; Dielectric Science and Technology Division
B02 Carbon Nanostructures in Medicine and Biology
Delphine Bouilly, Ardemis Boghossian, Tatiana Da Ros, Markita Landry, Jeffrey Halpern, Mekki Bayachou, Jessica Koehne, Anton Naumov, Nicole Iverson, Noe Alvarez, Daniel Heller
Nanocarbons Division; Organic and Biological Electrochemistry Division
B03 Carbon Nanotubes - From Fundamentals to Devices
Ming Zheng, R. Bruce Weisman, Slava Rotkin, Shigeo Maruyama, Yan Li, Benjamin Scott Flavel, Yutaka Ohno, Emilio Perez, YuHuang Wang
Nanocarbons Division
B04 NANO in India
Slava Rotkin, Francis D’Souza, Anunay Samanta, K. George Thomas, Angshuman Nag
Nanocarbons Division
B05 Fullerenes - Endohedral Fullerenes and Molecular Carbon, in Memory of Bob Curl
Yoko Yamakoshi, Alan Balch, Francis D’Souza, Luis Echegoyen, Dirk Guldi, Nazario Martín, Steven Stevenson, Shangfeng Yang, Akimitsu Narita, Uroš Cvelbar
Nanocarbons Division
B06 2D Layered Materials from Fundamental Science to Applications
Michael Scott Arnold, Uroš Cvelbar, Slava Rotkin, Yaw Obeng, Stefan De Gendt, Zia Karim, Stephen E. Creager, Elisa Miller-Link, Richard Martel
Nanocarbons Division; Dielectric Science and Technology Division
B07 Light Energy Conversion with Metal Halide Perovskites, Inorganic/Organic Hybrid Materials, and Dynamic Exciton
Hiroshi Imahori, Kei Murakoshi, Tsukasa Torimoto, Mahesh
Hariharan, Zhiqun Lin, Prashant Kamat
Nanocarbons Division
B08 Porphyrins, Phthalocyanines, and Supramolecular Assemblies
Nathalie Solladie, Karl Kadish, Tomas Torres, Roberto Paolesse, Norbert Jux, Ãngela Sastre-Santos
Nanocarbons Division
B09 Nano for Industry
R. Bruce Weisman, Dan Wang, Thorsten Lill, Oana Leonte, David Estrada, Mark Hersam, Slava Rotkin
Nanocarbons Division; Dielectric Science and Technology Division; Industrial Electrochemistry and Electrochemical Engineering Division
B10 On-Surface Synthesis of Carbon Nanomaterials
Nazario Martín, Hiroshi Imahori, David Ecija, Wilhelm Auwarter
Nanocarbons Division
C Corrosion Science and Technology
C01 Corrosion General Session
Dev Chidambaram, Eiji Tada
Corrosion Division
D Dielectric Science and Materials
D01 Chemical Mechanical Polishing 17
Bahar Basim, Yaw Obeng, Vimal Chaitanya, Robert Rhoades, Gautam Banerjee, Jason Keleher
Dielectric Science and Technology Division
D02 Dielectrics for Nanosystems 10: Materials Science, Processing, Reliability, and Manufacturing
Durgamadhab Misra, Toyohiro Chikyow, Dong-Kyun Ko, Yaw Obeng, Zhi Chen, Daniel Bauza, Kay Song
Dielectric Science and Technology Division
D03 Nanoscale Luminescent Materials 8
Peter Mascher, Dong-Kyun Ko, David Lockwood, Federico Rosei
Dielectric Science and Technology Division; Electronics and Photonics Division
D04 Quantum Dot Science and Technology 3
Dong-Kyun Ko, Vladimir Svrcek, Soong Ju Oh, Preston Todd Snee, Ivan Marri, Qiliang Li, Danielle Reifsnyder Hickey, Andrew Greytak
Dielectric Science and Technology Division; Electronics and Photonics Division; Nanocarbons Division
D05 Plasma Electrochemistry and Catalysis 3
Davide Mariotti, Uroš Cvelbar, Mohan Sankaran, Mahendra
Sunkara
Dielectric Science and Technology Division; Energy Technology Division
E Electrochemical/Electroless Deposition
E01 Magnetic Materials Processes and Devices 16
Stanko Brankovic, Adriana Ispas, Luca Magagnin Electrodeposition Division
F Electrochemical Engineering
F01 Advances in Industrial Electrochemistry and Electrochemical Engineering
Maria Inman, Elizabeth Biddinger, Paul Kenis
Industrial Electrochemistry and Electrochemical Engineering Division; Electrodeposition Division
F02 Highlighting Startups Pursuing Electrochemical Manufacturing
William Tarpeh, Juan Lopez-Ruiz
Industrial Electrochemistry and Electrochemical Engineering Division; Electrodeposition Division
G Electronic Materials and Processing
G01 G01—Silicon Compatible Emerging Materials, Processes, and Technologies for Advanced CMOS and Post-CMOS Applications
14
Hemanth Jagannathan, Zia Karim, Kuniyuki Kakushima, Paul J. Timans, Evgeni Gousev, Stefan De Gendt, Durgamadhab Misra, Yaw Obeng, Fred Roozeboom
Electronics and Photonics Division; Dielectric Science and Technology Division
G02 Organic Semiconductor Materials, Devices, and Processing 9
Benjamin Iniguez, Jamal Deen, Hagen Klauk, David Gundlach, Zhi Chen, Sunghwan Lee Electronics and Photonics Division
H Electronic and Photonic Devices and Systems
H01 Wide-Bandgap Semiconductor Materials and Devices 25
Qiliang Li, Steve Kilgore, Jennifer Hite, Vidhya Chakrapani, Travis Anderson, Marko Tadjer
Electronics and Photonics Division; Dielectric Science and Technology Division
H02 Solid State Electronics and Photonics in Biology and Medicine 10
Zong-Hong Lin, Yu-Lin Wang, Wenzhuo Wu, Chih-Ting Lin, Toshiya Sakata, Mark Ming-Cheng Cheng, Lluís Marsal, Revathy Padmanabhan
Electronics and Photonics Division
I Fuel Cells, Electrolyzers, and Energy Conversion
I01 Low Temperature Water Electrolysis (LT-WE) for H2
Production 2
Hui Xu, Karen Swider-Lyons, William Mustain, Marcelo Carmo, Svitlana Pylypenko, Shaun Alia, Peter Strasser, Sara Cavaliere, Shigenori Mitsushima, Karel Bouzek, Fan Yang
Energy Technology Division; Industrial Electrochemistry and Electrochemical Engineering Division; Physical and Analytical Electrochemistry Division
I02 Renewable Fuels via Artificial Photosynthesis or Heterocatalysis
10
Nianqiang Nick Wu, Vaidyanathan Subramanian, Ayyakkannu Manivannan, Pawel Kulesza, Frank Osterloh, Bunsho Ohtani, Eric Miller, Gary Wiederrecht, Tianquan Lian, Heli Wang Energy Technology Division; Physical and Analytical Electrochemistry Division; Sensor Division
I03 Materials for Low Temperature Electrochemical Systems 10
Minhua Shao, Gang Wu, Enyuan Hu Energy Technology Division; Battery Division; Physical and Analytical Electrochemistry Division
I04 Electrosynthesis of Fuels 8
Xiao-Dong Zhou, Hui Xu, Huyen Dinh, Gessie Brisard, Dong Ding, Jianhua Tong, Damilola Daramola
High-Temperature Energy, Materials, & Processes Division; Energy Technology Division; Industrial Electrochemistry and Electrochemical Engineering Division
I05 Mechano-Electro-Chemical Coupling in Energy Related Materials and Devices 5
Jason Nicholas, Nicola Perry, Chengcheng Fang
High-Temperature Energy, Materials, & Processes Division; Battery Division
I06 Energy Conversion Based on N, P, and Other Nutrients 3 Lea Winter, Marta Hatzell, William Tarpeh, Gang Wu, Julie Renner, Pawel Kulesza, Damilola Daramola Energy Technology Division; Industrial Electrochemistry and Electrochemical Engineering Division; Physical and Analytical Electrochemistry Division
K Organic and Bioelectrochemistry
K01 Advances in Organic and Biological Electrochemistry: In Memory of Diane Smith
Kevin Moeller, Dan Little, Shelley Minteer, Mekki Bayachou
Organic and Biological Electrochemistry Division
K02 Bioelectrochemistry – From Ions to Proteins to Living Organisms
Sabine Kuss, Lior Sepunaru, Sanela Martic, Sadagopan Krishnan, Alice Suroviec
Organic and Biological Electrochemistry Division; Physical and Analytical Electrochemistry Division
L Physical and Analytical Electrochemistry, Electrocatalysis, and Photoelectrochemistry
L01 Physical and Analytical Electrochemistry, Electrocatalysis, and Photoelectrochemistry General Session
Anne Co, Stephen Paddison
Physical and Analytical Electrochemistry Division
L02 Computational Electrochemistry 9
Stephen Paddison, Yue Qi, Scott Calabrese Barton, Steven DeCaluwe
Physical and Analytical Electrochemistry Division; Energy Technology Division
L03 Electrochemical Studies by Synchrotron Techniques 3
Svitlana Pylypenko, Anne Co, Iryna Zenyuk, Kelsey Stoerzinger
Physical and Analytical Electrochemistry Division; Battery Division; Electrodeposition Division; Energy Technology Division
L05 L05—Electrochemistry at the Nanoscale 2
Plamen Atanassov, Vito Di Noto
Physical and Analytical Electrochemistry Division; Electrodeposition Division; Energy Technology Division
M Sensors
M01 Recent Advances in Sensors Systems 5
Praveen Kumar Sekhar, Jessica Koehne, Aida Ebrahimi, Dongmei Dong, Thiagarajan Soundappan, Larry Nagahara Sensor Division
M02 Printed and Wearable Sensors and Systems
Jessica Koehne, Milton Cordeiro, Lok-kun Tsui, Leyla Soleymani, Milad Navaei, Aida Ebrahimi, Yaw Obeng, Uroš Cvelbar Sensor Division; Dielectric Science and Technology Division; Nanocarbons Division
M03 Sensors for Energy Production, Conversion, and Storage
Dongmei Dong, Rangachary Mukundan, Gary W. Hunter, Praveen Kumar Sekhar, A. Robert Hillman, Kannan Ramaiyan, Milad Navaei Sensor Division; High-Temperature Energy, Materials, & Processes Division
Z General
Z01 General Student Poster Session Alice Suroviec All Divisions
Z02 Education in Electrochemistry 4
Alice Suroviec, Plamen Atanassov, Thomas Fuller, Christopher Arges, Praveen Kumar Sekhar
Physical and Analytical Electrochemistry Division; Battery Division; Corrosion Division; Energy Technology Division; Industrial Electrochemistry and Electrochemical Engineering Division; Sensor Division
Z03 Electrochemical Recovery, Recycling, and Sustainability of Critical and Value Added Materials 2
Chockkalingam Karuppaiah, Natasa Vasiljevic, Zheng Chen, Luca Magagnin, Xiao Su, Santosh Vijapur, Shiqiang Zou, Luis Diaz Aldana, Shuang Andersen, Hojong Kim
Industrial Electrochemistry and Electrochemical Engineering Division; Battery Division; Electrodeposition Division; Energy Technology Division; High-Temperature Energy, Materials, & Processes Division; Interdisciplinary Science and Technology Subcommittee
Z04 Electrochemistry for Chemical Manufacturing 2
Elizabeth Biddinger, Chockkalingam Karuppaiah, Wenzhen Li, René Böttcher, Paul Kenis, Scott Calabrese Barton, Martin Leimbach Industrial Electrochemistry and Electrochemical Engineering Division; Battery Division; Corrosion Division; Electrodeposition Division; Energy Technology Division; Organic and Biological Electrochemistry Division; Interdisciplinary Science and Technology Subcommittee
Z05 Materials, Devices, and Systems for Neuromorphic Computing and Artificial Intelligence Hardware
Durgamadhab Misra, Kuniyuki Kakushima, Revathy Padmanabhan, Vidhya Chakrapani, Jeff L. Blackburn, Gitanjali Kolhatkar, Bilge Yildiz
Dielectric Science and Technology Division; Electronics and Photonics Division; High-Temperature Energy, Materials, & Processes Division; Nanocarbons Division; Interdisciplinary Science and Technology Subcommittee
2023 was another amazing year for ECS Publications—another year of accelerating scientific discovery, championing the dissemination of research, and supporting authors in their goal to leave their mark on the scholarly record.
Readers of the highly cited content published by The Electrochemical Society are located in every continent of the globe, with downloads of our content already exceeding nine million, from Australia, China, the USA, Canada, New Zealand, and countless other nations; connecting their research with authors from 65 nations, including but not limited to Indonesia, Ukraine, Sweden, Germany, Mexico, France, and Canada. We saw growth in the number of citations of this impactful research and are proud to say that the content of our digital library is more than 50% open access!
To our Peer Reviewers: Thank you for taking time out of your busy schedules and lives to share your expertise and feedback
with the prospective authors of our published content. Without our reviewers’ contributions, our editors would not be equipped with the vital feedback necessary for the peer review process. We hope that you will continue to serve ECS and the scientific community in 2024 and, we hope, be among the first to be recognized in our NEW! Peer Review Excellence Recognition Program
To our Editors: Thank you not only for your commitment to the quality and integrity of the ECS publications portfolio but also to the Society’s overall mission. Through your dedicated efforts, ECS is able to further our vision to be the recognized steward of electrochemical and solid-state science and technology by advancing theory and practice. We have reappointed many of our editors in 2024 and have added new esteemed members to our editorial boards to continue to maintain our standard of excellence!
To our Authors: We know that you have options for where you publish your precious work. We are grateful that you chose the ECS family of publications as your publications home. For over 120 years, ECS publications have contributed to the scholarly record as a community publisher and a place where authors—whether they are early career or highly experienced senior scientists—come together to advance scientific research into the future.
Thanks to all of your efforts, ECS continues to be a community publisher of quality content for the members of the electrochemical and solid-state science communities.
Gang Wu
Initial Appointment as Associate Editor for the Journal of The Electrochemical Society Fuel Cells, Electrolyzers, and Energy Conversion topical interest area (TIA) for the term December 1, 2023 –November 30, 2025
Wataru Sugimoto
Initial Appointment as Associate Editor for the Journal of The Electrochemical Society Battery and Energy Storage TIA for the term January 1, 2024 –December 31, 2025
Scott Donne Associate Editor for the Journal of The Electrochemical Society January 1, 2019 –December 31, 2023
Meng Tao Associate Editor for the ECS Journal of Solid State Science and Technology May 1, 2019 – December 31, 2023
The summer issue of Interface is scheduled to hit your mailbox on June 28. It will be guest edited by the Electrodeposition Division's Natasa Vasiljevic and Rohan Akolkar. The summer issue will also feature the new
2024 ECS officers; a chance to meet the new ECS Advances editor-in-chief; and of course Society, division, student, and people news; Looking at Patent Law; and Tech Highlights.
Battery
Brett Lucht, Chair
University of Rhode Island
Jie Xiao, Vice Chair
Jagjit Nanda, Secretary
Xiaolin Li, Treasurer
Doron Aurbach, Journals Editorial Board Representative
Corrosion
Dev Chidambaram, Chair
University of Nevada Reno
Eiji Tada, Vice Chair
Rebecca Schaller, Secretary/Treasurer
Sannakaisa Virtanen, Journals Editorial Board Representative
Dielectric Science and Technology
Uroš Cvelbar, Chair
Jožef Stefan Institute
Sreeram Vaddiraju, Vice Chair
Zhi David Chen, Secretary
Thorsten Lill, Treasurer
Peter Mascher, Journals Editorial Board Representative
Electrodeposition
Luca Magagnin, Chair
Politecnico di Milano
Andreas Bund, Vice Chair
Rohan Akolkar, Secretary
Adriana Ispas, Treasurer
Takayuki Homma, Journals Editorial Board Representative
Electronics and Photonics
Qiliang Li, Chair
George Mason University
Vidhya Chakrapani, Vice Chair
Zia Karim, 2nd Vice Chair
Helmut Baumgart, Secretary
Travis Anderson, Treasurer
Khanna Aniruddh Jagdish, Journals Editorial Board Representative
Fan Ren, Journals Editorial Board Representative
Energy Technology
Katherine Ayers, Chair
Nel Hydrogen
Minhua Shao, Vice Chair
Hui Xu, Secretary
Iryna Zenyuk, Treasurer
Minhua Shao, Journals Editorial Board Representative
High-Temperature Energy, Materials, and Processes
Cortney Kreller, Chair
Los Alamos National Laboratory
Xingbo Liu, Senior Vice Chair
Teruhisa Horita, Junior Vice Chair
Dong Ding, Secretary/Treasurer
Minhua Shao, Journals Editorial Board Representative
Industrial Electrochemistry and Electrochemical Engineering
Maria Inman, Chair
Faraday Technology, Inc.
Paul Kenis, Vice Chair
Elizabeth Biddinger, Secretary/Treasurer
Paul Kenis, Journals Editorial Board Representative
Luminescence and Display Materials
Eugeniusz Zych, Chair
Uniwersytet Wrocławski
Chong-Geng Ma, Vice Chair
Marco Bettinelli, Secretary/Treasurer
Im Won Bin, Journals Editorial Board Representative
Nanocarbons
Jeff L. Blackburn, Chair
National Renewable Energy Laboratory
Ardemis Boghossian, Vice Chair
Yan Li, Secretary
Hiroshi Imahori, Treasurer
Dirk M. Guldi, Journals Editorial Board Representative
Organic and Biological Electrochemistry
Shelley Minteer, Chair
University of Utah
Jeffrey Halpern, Vice Chair
Sabine Kuss, 2nd Vice Chair
Ariel Furst, Secretary/Treasurer
Janine Mauzeroll, Journals Editorial Board Representative
Physical and Analytical Electrochemistry
Stephen Paddison, Chair
University of Tennessee, Knoxville
Anne Co, Vice Chair
Svitlana Pylypenko, Secretary
Iwona Rutkowska, Treasurer
David Cliffel, Journals Editorial Board Representative
Sensor
Larry Nagahara, Chair
Johns Hopkins University
Praveen Kumar Sekhar, Vice Chair
Dong-Joo Kim, Secretary
Leyla Soleymani, Treasurer
Netz Arroyo, Journals Editorial Board Representative
Stefano Cinti, Journals Editorial Board Representative
These divisions have nominated new officers for the spring 2024 to spring 2026 term. Elections take place from April 1 through April 30, 2024. The results are reported in the summer 2024 issue of Interface.
Chair
Sreeram Vaddiraju, Texas A&M University
Vice Chair
Eva Kovacevic, GREMI/Université d’Orléans
Secretary
Zhi David Chen, University of Kentucky
Treasurer
Thorsten Lill, Lam Research Corporation
Member at Large
Gautam Banerjee, Micron Technology Inc.
Vimal H. Chaitanya, New Mexico State University
Stefan De Gendt, IMEC
Dennis Hess, Georgia Institute of Technology
Hemanth Jagannathan, IBM Corporation Research Center
Zia Karim, Yield Engineering Systems
Steve Kilgore, NXP Semiconductor
Dong-Kyun Ko, New Jersey Institute of Technology
Paul Kohl, Georgia Institute of Technology
Hiroki Kondo, Nagoya University
Sunghwan Lee, Purdue University
Oana Leonte, Berkeley Polymer Technologies, Inc.
Durga Misra, New Jersey Institute of Technology
Yaw Obeng, National Institute of Standards and Technology
Neelakandan Santhosh Marath, Jožef Stefan Institute
Kay Song, Yield Engineering Systems
Kalpathy Sundaram, University of Central Florida
Mahendra Sunkara, University of Louisville
Chair
Paul Kenis, University of Illinois at Urbana-Champaign
Vice Chair
Elizabeth Biddinger, City College of New York
Division Secretary/Treasurer
Chockalingam Karuppaiah, Vetri Labs
Member at Large
Christopher Arges, Argonne National Laboratory
Saket Bhargava, Dow Chemical Company
Gerardine Botte, Texas Tech University
Fikile Brushett, Massachusetts Institute of Technology
Damilola Daramola, Northeastern University
Luis Diaz Aldana, Idaho National Laboratory
James Fenton, University of Central Florida
Taylor Garrick, General Motors Holdings, LLC
Matthew Graaf, Corteva Agriscience
John Harb, Brigham Young University
Marta Hatzell, Georgia Institute of Technology
Shrisudersan Jayaraman, Corning, Inc.
Wenzhen Li, Iowa State University
Juan Lopez-Ruiz, Pacific Northwest National Laboratory
Trung Van Nguyen, University of Kansas
Mark E. Orazem, University of Florida
Tyler Petek, Lubrizol Corporation
Doug Riemer, TDK Ventures
Shrihari Sankarasubramanian, University of Texas at San Antonio
Robert Savinell, Case Western Reserve University
John Staser, Ohio University
Xiao Su, University of Illinois at Urbana-Champaign
Venkat Subramanian, University of Texas at Austin
William Tarpeh, Stanford University
Santosh Vijapur, Faraday Technology, Inc.
John Weidner, University of Cincinnati
Chair
TBD
Nanocarbons
Vice Chair
Ardemis Boghossian, École Polytechnique Fédérale de Lausanne
Secretary
Yan Li, Peking University
Treasurer
Hiroshi Imahori, Kyoto University
Member at Large
Noe Alvarez, University of Cincinnati
Mike Arnold, University of Wisconsin–Madison
Delphine Bouilly, Université de Montréal
Tatiana Da Ros, Università degli Studi di Trieste
Francis D’Souza, University of North Texas
Andrew Ferguson, National Renewable Energy Laboratory
Daniel Heller, Memorial Sloan Kettering Cancer Center
Mark Hersam, Northwestern University
Nicole Iverson, University of Nebraska–Lincoln
Markita Landry, University of California, Berkeley
Fernando Langa, Universidad de Castilla–La Mancha
Richard Martel, Université de Montréal
Nazario Martín, Universidad Complutense de Madrid
Shigeo Maruyama, University of Tokyo
Elisa Miller-Link, National Renewable Energy Laboratory
Anton Naumov, Texas Christian University
Roberto Paolesse, Università di Roma Tor Vergata
Slava V. Rotkin, The Pennsylvania State University
Ángela Sastre-Santos, Universidad Miguel Hernández de Elche
Steve Stevenson, Purdue University
Tomás Torres, Universidad Autónoma de Madrid
R. Bruce Weisman, Rice University
Yoko Yamakoshi, Laboratorium für Organische Chemie –ETH Zürich
Shangfeng Yang, University of Science and Technology of China
Ming Zheng, National Institute of Standards and Technology
It is with great pleasure that we recognize the following ECS members who have reached their 30, 40, 50, and 60-year anniversaries with the Society in 2024. Congratulations to you all!
Arthur H. Graham
Donald L. Maricle
Robert A. Rapp
Brooke Schumm
Rajaram Bhat
Uri Cohen
Robert B. Comizzoli
Gary M. Crosbie
Harry O. Finklea
Dennis W. Hess
Thomas D. Kaun
Kyong-Min Kim
John A. Kosek
Samuel C. Levy
J. Ross MacDonald
James A. McIntyre
Kashmiri L. Mittal
Shohei Nakahara
Richard E. Novak
Bruce A. Parkinson
Wayne H. Smith
Ralph E. White
Gilbert M. Brown
Pietro Cavallotti
Thomas W. Chapman
Fausto Croce
Lili Deligianni
Gerald R. Dietze
Ross E. Dueber
Robert Paul Flicker
Stephen J. Fonash
Jorge G. Ibañez
Robert G. Kelly
Wayne I. Kinney
Wilbur C. Krusell
Andrzej Lasia
Jean Lessard
Stuart Licht
Jacek Lipkowski
Marcin Majda
Richard L. McCreery
Trung Van Nguyen
Mikael Östling
Miguel Mateo Paz Soldán
Ralph A. Petersen
Mark D. Pritzker
Daniel A. Scherson
César Augusto C. Sequeira
Michael Paul Setter
James J. Steppan
Jean St-Pierre
John R. Susko
Robin A. Susko
Jun-ichi Yamaki
Cammy R. Abernathy
Stuart B. Adler
Pankaj Arora
David Reed Atherton
Supriyo Bandyopadhyay
Christina Bock
Felix N. Büchi
Nieves Casañ-Pastor
Yufei Chen
Stuart M. Davis
Emory Sayre De Castro
Felice DiMascio
Huyen Ngoc Dinh
Scott W. Donne
Yasuhiro Fukunaka
Diana Golodnitsky
Srikanth Gopalan
Arthur M. Greenberg
Hiroki Habazaki
Michael T. Hicks
Bing-Joe Hwang
Tatsumi Ishihara
Radha S. Jalan
Patrick I. James
John R. Johnson
Jerry J. Kaczur
Oana M. Leonte
David J. Lockwood
Dennie T. Mah
Mani Manivannan
Robert A. Mantz
Jeremy P. Meyers
Minoru Mizuhata
Krystof J. Mocala
Masatsugu Morimitsu
Rangachary Mukundan
Evert Nagel Soepenberg
Hameed A. Naseem
Ikuzo Nishiguchi
Kazuyoshi Okuno
Jinshan Pan
Stefano Passerini
Fan Ren
Mamoru Saito
Yoji Sakurai
Kazunari Sasaki
Patrik Schmuki
Akshey Sehgal
Peter Miles Anson Sherwood
Drew Sinha
Giovanni Sotgiu
Kalpathy B. Sundaram
Yung-Eun Sung
Waltraud Taucher-Mautner
Thomas N. Tucker
Atsushi Ueda
Steven Joseph Visco
R. Bruce Weisman
MasahiroYanagida
April
Selected for you by
Alice H. SuroviecThe Journal of the Electrochemical Society is the flagship journal of the Society. With an impact factor of 3.9 and a SCOPUS score of 7.2, this journal should be everyone’s first landing spot when looking for current research in the solid state and electrochemical sciences.
While there are several focus issues every year, the focus issue on Electrochemical Separations and Sustainability is well suited to match the content of this Interface issue.
https://iopscience.iop.org/journal/1945-7111/page/focusissue-on-electrochemical-separations-and-sustainability
These open access databases contain Gibbs energy models and values for molten salt components. There is also a comprehensive list of MSTDB reference documents and publications.
https://mstdb.ornl.gov
The explainer articles are a series of informative articles that cover a wide variety of topics. The articles are written for the novice scientist looking to learn more about a topic. The articles all link to current research at PNNL as well as current literature on the topic. An example is linked below to an article on Renewable Integration.
https://www.pnnl.gov/explainer-articles/renewableintegration
Alice Suroviec is a Professor of Bioanalytical Chemistry and Dean of the School of Mathematical and Natural Sciences at Berry College. She earned a BS in Chemistry from Allegheny College in 2000. She received her PhD from Virginia Tech in 2005 under the direction of Dr. Mark R. Anderson. Her research focuses on enzymatically modified electrodes for use as biosensors. She is a Fellow of the Electrochemical Society and Associate Editor of the PAE topical interest area for the Journal of the Electrochemical Society. She welcomes feedback from the ECS community.
https://orcid.org/0000-0002-9252-2468
JES is the flagship journal of The Electrochemical Society. Published continuously from 1902 to the present, JES remains one of the most highly cited journals in electrochemistry and solid state science and technology.
JSS is a peer-reviewed journal covering fundamental and applied areas of solid state science and technology, including experimental and theoretical aspects of the chemistry and physics of materials and devices.
ECS Sensors Plus is a one-stop shop journal for sensors. This multidisciplinary, Gold Open Access journal provides an international platform for publishing high-quality impactful articles and promoting scholarly communication and interactions among scientists, engineers, and technologists whose primary interests focus on materials, structures, properties, performance, and characterization of sensing and detection devices and systems, including sensor arrays and networks.
ECS Advances is a multidisciplinary, Gold Open Access forum of peer-reviewed, high-quality content covering all technical areas supported by the Society. ECS Advances publishes full-length original work, brief communicationstyle papers, perspectives, review articles, and special issues.
SUBMIT TODAY! SUBMIT
Transportation is one of the most important and visible indicators of the mobility of people and goods, both globally and locally. A rather less visible aspect of the transportation sector is freight movement, which is considered the backbone of many sectors and of the economy overall. In the United States, for example, the freight transportation services index very closely follows the gross domestic product (GDP), which illustrates how a nation’s economic activity and output is tied to freight transportation (Fig. 1a).
While freight movement occurs in many modes—from on-road transportation to rail, aviation, and shipping—heavy-duty truckinga is responsible for most of the cargo movement in the nation. In 2021, trucks carried 72% of US freight in terms of value and almost twothirds of freight in terms of tonnage (Fig. 2).1,2 In addition, the US Department of Transportation (DOT) projects an almost 50% increase in the nation’s freight capacity by 2050, the majority of which is still expected to be handled by trucks.2 With their dominant role in freight movement, trucks contribute over $390 billion to the US economy as part of the transportation and warehouse sector, with a 5% share of the GDP.1,2 Trucking also has the most employees in transportationrelated occupations, with almost 2 million drivers for heavy-duty and tractor-trailer trucks, which includes drivers working within the transportation and warehousing sector and in non-transportation sectors (e.g., truck drivers employed by the wholesale and retail trade industry), see Fig. 1b.
Moving the majority of the nation’s cargo requires a lot of energy. Energy demand by trucks reached 5.55 quads in 2022, constituting 20% of the transportation energy use in highways.4 This is the result of a combination of longer annual miles traveled by trucks and their much higher fossil fuel consumption per mile compared to light duty vehicles (LDVs) like passenger cars. In fact, the gap between the annual fuel consumption and miles traveled per truck versus LDV has been increasing over the decades (Fig. 3). A direct consequence of this activity is the higher greenhouse gas emissions by the internal combustion engines of trucks. The transportation sector is currently the major source of carbon emissions in the United States, with
a In this article, trucks are used to describe heavy-duty trucks including, but not limited to, Class 8 and combination trucks. Due to the differences in classifications of vehicles, or their grouping, between reports cited in this article, values and facts shown in each figure reflect the designations used in the original datasets. Interested readers are encouraged to check the referenced online databases for details on reporting structures and methodologies.
energy use and resulting emissions despite constituting only 5% of the entire vehicle inventory.
While these annual emissions provide a picture painted by broad brushes, the real impact of the transportation emissions is the air pollutants associated with the combustion of the gasoline and diesel fuel, including carbon monoxide (CO), hydrocarbon (HC) and nitrogen oxide (NOₓ), and particulate matter (PM). Transportationrelated GHG emissions primarily originate from burning gasoline and diesel fuel for light and heavy-duty vehicles, with additional emissions coming from jet fuel in aviation and other fuels (Fig. 6). The share of gasoline fuel consumption by light-duty trucks is the
a share of 29% in 2021 according to the EPA (Fig. 4). Within the transportation sector, heavy-duty trucks are responsible for almost a quarter of these emissions. In fact, trucks are responsible for 7% of annual greenhouse gas (GHG) emissions, which puts the HDV sector on par with the residential and commercial sectors in terms of share of carbon emissions.
The share of the trucking sector in carbon emissions, however, can be understood much better if the vehicle inventory is considered. Of the entire US vehicle fleet (282 million as of 2021), only 5% are heavy-duty trucks, including single-unit and combination trucks (10.7 and 3.1 million, respectively).1,2 Despite their small fraction of the vehicle fleet, trucks cover ~10% of the total annual miles traveled on the highways, consume 29% of the transportation fuels annually, and contribute 28% of annual transportation emissions (Fig. 5). These numbers illustrate the disproportionally high impact of trucks on
highest, and MDV/HDV trucks and buses consume 90% of diesel fuel.2,5 A better way to assess and compare these emissions from different classes is the emissions per mile of vehicle travel (Fig. 7). HDVs emit significantly more NOx and HC per mile traveled compared to light-duty passenger cars and trucks.1-3
A major environmental and health concern is PM2.5 emissions, especially from diesel engines. PM2.5 stands for particulate matter with a diameter of less than 2.5 micrometers. While particulate matter in general refers to a mixture of solid particles and liquid droplets in the air, PM2.5 poses the greatest risk to health. PM2.5 emissions are
transportation sector are strongly intertwined. One way to accomplish this is to reduce vehicle mileage; yet, given the increasing global economic activity, there is increased freight movement projected in the United States, and heavy-duty truck mileage in the world toward 2050 (Fig. 10). IEA Net-zero scenariob projections for economic indicators show increases in the energy demand and total mileage for trucks compared to passenger cars.9,10
As is the case in the United States, on a global scale, heavy trucks also release more CO2 per distance traveled than passenger cars (Fig. 10).9 Yet, achieving net-zero emissions globally by 2050 requires decarbonization of transportation sector. Therefore, this dichotomy between the increasing freight tonnage and truck mileage vs. higher per-vehicle emissions from trucks calls for a significantly higher reduction in emissions in HDVs compared to LDVs, as illustrated in the IEA analysis (Fig. 10). The disparities in per-vehicle mileage and fuel consumption metrics for HDVs (in contrast to LDVs) results in a disproportionally higher impact of trucks on emissions
linked to many adverse health effects, such as lung and heart diseases and increased respiratory symptoms.6 HDV trucks release up to 50 PM2.5 emissions/mile more than do LDV cars. Converting these values to the total annual emission per vehicle reveals a striking difference between light and heavy-duty vehicles: a diesel truck emits over 100 times more PM2.5 than does a gasoline passenger car (Fig. 8).
In other words, in terms of eliminating total PM2.5 emissions and mitigating their adverse health impacts, decarbonizing one diesel truck would be equivalent to decarbonizing ~100 passenger cars 1,3 Such an action would not only reduce transportation-caused GHG emissions and their influence on climate change, it will also negate the harmful pollutants and their impact on communities. In fact, the effects of PM2.5 emissions on communities also change with geography and demographics.7,8 A recent study reported disproportional impact of PM2.5 emissions on underserved populations, which was found to be the case also for transportation-related emissions, including heavyduty trucks (Fig. 9a). On a global scale, a recent update by IEA on NZE7 also noted the disparities in overall PM2.5 concentration among the advanced and emerging market economies (Fig. 9b). In addition to the critical role of heavy-duty diesel trucks in contributing to PM2.5 emissions, the adverse health effects of these emissions also vary by region, economy, income, and demographics, making this an energy equity issue and environmental justice problem.
Thus, eliminating harmful pollutants from combustion of fuels in vehicles, especially heavy-duty trucks, and decarbonizing the
b The Net Zero Emissions by 2050 Scenario (NZE) is a normative IEA scenario that shows a pathway for the global energy sector to achieve net zero CO2 emissions by 2050, consistent with limiting the global temperature rise to 1.5 °C with no or limited temperature overshoot (with a 50% probability), in line with reductions assessed by the Intergovernmental Panel on Climate Change (IPCC) in its Sixth Assessment Report.
and air pollution, which makes the decarbonization of the trucking sector a distinct challenge. As such, overcoming this challenge with zero-emission truck technologies will also lead to a significantly more positive change for demographics and communities impacted most adversely by the emissions and air pollutants from the freight movement and truck activity on highways, warehouses, and ports, as well as off-road activity such as construction and mining. This impact of decarbonization, aided by the increasing share of zero-emission vehicles, on emission reduction is captured in the IEA NZE analysis which projects a global decrease in PM2.5 concentrations from current levels if the targets are achieved by 2050 (Fig. 9b).7
This article brings together disparate datasets on heavy-duty transportation to establish a visual story of key facts and figures on heavy-duty trucks and freight transportation, as well as their role in greenhouse gas emissions and associated impact on people. In light of increased emphasis on decarbonization of the transportation sector given its major share in emissions, emergence of zero-emission truck regulations to aid decarbonization efforts, and development of roadmaps and analysis on electrification of the trucking sector (with batteries and hydrogen fuel cells), this article aims to provide a brief yet coherent story on the facts and challenges regarding freight movement and the trucking sector, with a focus on numbers in the United States from various government databases (EPA, BTS, EIA) along with global projections by the IEA and US AEO As decarbonization efforts continue, there will be a growing need to analyze the transportation sector by accounting for the unique characteristics of each mode, including and especially, heavy-duty trucks and freight movement.
The views and opinions of the author expressed herein do not necessarily state or reflect those of the United States Government or any agency thereof. Neither the United States Government nor any agency thereof, nor any of their employees, makes any warranty, expressed or implied, or assumes any legal liability or responsibility for the accuracy, completeness, or usefulness of any information, apparatus, product, or process disclosed, or represents that its use would not infringe privately owned rights.
© The Electrochemical Society. DOI: 10.1149/2.F04241IF
Ahmet Kusoglu, Staff Scientist, Lawrence Berkeley National Laboratory
Education: PhD (University of Delaware), Post-doctoral Fellow at Berkeley Lab. Research Interests: Ionomers, Polymer electrolytes, Mechanochemistry, Structureproperty relationships, Hydrogen technologies, Fuel cells and electrolyzers.
Pubs + Patents: >80 peer-reviewed journal papers, 2 book chapters, >50 invited talks and tutorials at international meetings, academia, and industry forums.
Work Experience: Scientist at Berkeley Lab working on functional polymers and interfaces for clean energy systems, Communications Director of the MillionMile Fuel Cell Truck (M2FCT) Consortium, and data visualization and data-driven stories on energy and environmental topics, including hydrogen, transportation, and electrochemical clean energy technologies.
Awards: Srinivasan Young Investigator Award and ECS Toyota Young Investigator Fellowship.
Work with ECS: Contributing Editor, ECS Interface; ECS member for 10+ years; Member-at-large, Energy Technologies Division (ETD), Symposium co-organizer for Polymer Electrolyte Fuel Cells and Electrolyzers.
Website: https://kusoglulab.lbl.gov
https://orcid.org/0000-0002-2761-1050
1. P. Hu, R. R. Schmitt, R. Robinson, et al. Transportation Statistics Annual Report 2022 (2022).
2. US Department of Transportation, Bureau of Transportation Statistics, Freight Facts and Figures.
3. US Department of Transportation, Bureau of Transportation Statistics (BTS), Freight Facts and Figures.
4. US Energy Information Agency, Monthly Energy Review.
5. Transportation Energy Data Book (ORNL), Ed 40.
6. Particulate Matter (PM) Pollution, EPA.
7. International Energy Agency (IEA). Net Zero by 2050: A Global Pathway to keep the 1.5 °C Goal in Reach (2023).
8. C. W. Tessum, D. A. Paolella, S. E. Chambliss, J. S. Apte, J. D. Hill, J. D. Marshall, Sci Adv, 7(18), eabf4491 (2021).
9. International Energy Agency (IEA), Net Zero by 2050: A Roadmap for the Global Energy Sector (2021).
10. International Energy Agency (IEA), World Energy Outlook (2020).
The European Federation of Corrosion (EFC) has awarded Michael Rohwerder the European Corrosion Medal, honoring his 25 years of significant contributions to the field of corrosion science. The prize consists of a bronze medal and a certificate.
The author of more than 200 publications on corrosion science, Rohwerder leads the corrosion group at the Max-Planck Institut für Eisenforschung (MPIE). His research includes metallic, organic, and self-healing coatings; hydrogen in materials; and corrosion. Rohwerder also has a strong interest in how metallic materials and coatings can be tailored for future sustainable energy applications: “To reduce carbon emissions and achieve a climate neutral industry, resilient infrastructures are required. Green energy often relies on the use of hydrogen as a renewable energy source. Meanwhile, hydrogen often leads to materials failure due to a phenomenon called hydrogen embrittlement. Also, other severe corrosion problems are seen in the green energy sector, for example in electrolyzers. Thus, solving corrosion problems is crucial for the transition to a sustainable future. This challenge drives my passion for corrosion science. Receiving the European Corrosion Medal has been a great honor and strengthens my motivation further,” says Rohwerder.
Joan B. Berkowitz, ECS President 1979–1980, passed away on January 22, 2020, at the age of 88. A distinguished chemist, her areas of research included materials for the space program, reusable molds for spacecraft construction built from molybdenum disilicides and tungsten disilicides, and the disposal and treatment of hazardous wastes. Dr. Berkowitz was the first woman to serve as president of The Electrochemical Society. She was born in Brooklyn, New York on March 13, 1931. She received her BA in chemistry from Swarthmore in 1952. She wished to follow her high school boyfriend (and fellow Swarthmore graduate) to Princeton University, but at the time Princeton did not accept women for graduate study in chemistry. Instead, she attended the University of Illinois Urbana-Champaign, graduating with a PhD in physical chemistry in 1955. From 1955 to 1957 Dr. Berkowitz was a National Science Foundation postdoctoral fellow at Yale University studying polymeric electrolytes.
Dr. Berkowitz joined Arthur D. Little, Inc. in 1957, where she was responsible for basic and applied research programs in high temperature chemistry and environmental science. She worked on the kinetics of oxidation of molybdenum, graphite, and the refractory borides and silicides. She investigated the effect of applied electrochemical potentials on reactions associated with hot corrosion of chromium-based alloys. She explored the thermodynamics of vaporization of high temperature materials in both vacuum and reactive atmospheres. Dr. Berkowitz also synthesized single crystals of high melting point materials and directed work on the growth of gallium arsenide for solar cell applications.
For the lunar space program, Dr. Berkowitz designed experiments in metals melting and eutectic solidification in space. She also assessed the possibility of photochemical reactions among the LEM exhaust products. She co-authored a monograph on industrial utilization of NASA developments in electroplating.
Rohwerder received the medal and presented his research at the European Corrosion Congress (EUROCORR) in August 2023. The EFC brings together 45 research organizations from 25 European countries to advance corrosion science through cooperation and networking.
In her pioneering environmental work, she headed a team that wrote the two-volume handbook, Physical, Chemical and Biological Treatment Techniques for Industrial Wastes (1976), a survey of manufactured goods and their potential for causing pollution. She examined limestone scrubbers that removed sulfur dioxide, demonstrated how to reduce hard deposits which hindered their effectiveness, and improved their design. She also studied the disposal of hazardous wastes in landfills and produced the first handbook on alternative disposal methods. By the 1980s she was a vice president and later head of the Environmental Business Worldwide section at A.D. Little.
In 1986 she left A.D. Little to become CEO of Risk Science International. In 1989 she co-founded the consulting firm Farkas Berkowitz & Company. In addition, she was an adjunct professor at the University of Maryland University College.
Dr. Berkowitz joined the Society in 1961. She was active in the Electrothermics and Metallurgy Division and served as Member-atLarge, Vice-Chair, and Chair (1971–1973). She became a Division Editor of JES in 1966. She served on many Society committees: Membership, Nominating, New Technology, and Ways and Means. She organized Society symposia on high temperature oxidation, oceanography, and energy storage. She authored over 35 technical papers and review papers in the fields of high temperature chemistry and oxidation of refractory hard materials and held 2 patents.
Dr. Berkowitz was also affiliated with the American Chemical Society, American Physical Society, Sigma Xi, Phi Beta Kappa, and the National Association of Corrosion Engineers. In 1970 she was a member of the National Academy of Science/National Academy of Engineering task group on high temperature oxidation-resistant coatings. She also chaired the chemistry panel for review of NAS/NRC fellowship applications. Her many awards include the Stanley J. Drazek award for excellence in teaching, the Sylvia M. Stoesser Lecturer in Chemistry, and the Achievement Award from the Society of Women Engineers.
Dr. Berkowitz is survived by her daughter, Rosemary, who is also a chemist.
Yasuhiko Ito, emeritus ECS member, passed away on October 17, 2023 at the age of 82. He joined The Electrochemical Society in 1985. He received the Physical and Analytical Electrochemistry Division Max Bredig Award in Molten Salt and Ionic Liquid Chemistry in 1999. He awarded Fellow of The Electrochemical Society in 2000. Professor Ito was born on March 14, 1941. He entered the Department of Industrial Chemistry, Faculty of Engineering, Kyoto University in 1959, and graduated from the same department in 1963. He completed a master’s course in the Department of Industrial Chemistry at the Graduate School of Engineering, Kyoto University in 1965, and received his PhD degree in 1969. In 1968, he was employed as an assistant professor in the Faculty of Engineering, Kyoto University, and in 1971, he was promoted to an associate professor at the Faculty of Liberal Arts, Kyoto University. In 1981, he was reassigned to the Department of Nuclear Engineering, Faculty of Engineering, Kyoto University, and in 1989, he was promoted to a professor at the same department. In May 1996, with the establishment of the Graduate School of Energy Science, Prof Ito was reassigned to the Department of Fundamental Energy Science at the same graduate school. He served as Dean of the Graduate School of Energy Science of Kyoto University for two terms from May 1998 to May 2002. During his many years of teaching students and supervising researchers at Kyoto University, he fostered many talented scientists.
After retiring from Kyoto University in 2004, Prof. Ito served as a professor in the Faculty of Engineering (currently Faculty of Science and Engineering) and the Graduate School of Engineering (currently Graduate School of Science and Engineering) at Doshisha University until 2011, where he again fostered many talented individuals through his education and research.
In Memoriam ...
David A. Vermilyea
1923–2022
David A. Vermilyea, ECS President 1974–1975, passed away on January 6, 2022 at the age of 98. Dr. Vermilyea joined The Electrochemical Society in 1955 and was active in the Corrosion and Physical Electrochemistry Divisions. He served as Secretary-Treasurer, ViceChairman, and from 1967 to 1969, as Chairman of the Theoretical (now Physical Electrochemical) Division. He served on many committees: Nominating, Young Authors, Ways and Means Subcommittee on Restructuring the Society, and Chairman of the Ad Hoc Committee on Education. He organized the symposiums “Mechanical Properties of Oxide Corrosion Products” in 1967 and “Structure and Characterization of Surface Reaction Products” in 1968.
In 2006, he established the venture company I’MSEP Co., Ltd. with the aim of developing and commercializing the research results accumulated at Kyoto University over many years, in addition to those newly obtained at Doshisha University, as technology seeds. He served as Representative Director since its establishment.
He was also a long-time member of the Electrochemical Society of Japan, contributing greatly to the development of the Society, mainly by chairing the Molten Salt Committee and the Committee of the Electrolytic Science and Technology. He served as Vice President of the Electrochemical Society of Japan from February 1997 to January 1999. From 2000 to 2002, he was Vice President of the International Society of Electrochemistry (ISE). In addition, he was an elected Fellow of ISE and of the Royal Norwegian Academy of Technical Sciences.
In Prof. Ito’s research field of high-temperature molten salt electrochemistry, he was responsible for many accomplishments in areas related to the fundamentals and applications of energy chemistry, industrial physical chemistry, and materials science. Among them, the electrolytic synthesis of ammonia in molten salt at atmospheric pressure was invented when he was at Kyoto University, and he devoted himself to its practical application until shortly before his passing. His foresight in recognizing the potential of ammonia as a hydrogen carrier long before it attracted attention deserves special mention. Many other unique and practical research achievements include the formation of various rare earth alloys using molten salt electrolysis, the electrochemical surface nitriding of various metals, and the electroplating of dense carbon film. Furthermore, he developed discharge electrolysis, in which electrolysis is performed by creating an electrical discharge between an electrode and a molten salt, which is a good example of his natural talent for invention.
He is survived by his wife Nobuko and three sons.
This note was contributed by Toshiyuki Nohira.
Dr. Vermilyea was elected Vice President of the Society in 1971 and served three years before being elected President in 1974. He received many prestigious ECS awards, including the Turner Book Prize (1954), Battery Division Outstanding Achievement Award (1975), Honorary Member of the Society (1977), and Edward Goodrich Acheson Award (1978). Other important awards Dr. Vermilyea received over his career include the Coolidge Fellowship from the GE Research Laboratory and the Whitney Award from the Association of Corrosion Engineers. Dr. Vermilyea served as Editor of the Annual Review of Materials Sciences; published 100 articles in scientific journals, with an exceptionally high number of citations; and was awarded 9 patents.
His early life was included service in the United States Army in Germany during World War II, where he drove and maintained a truck for an artillery battalion and was decorated with the Purple
(continued from previous page)
Heart. After the war, he earned a PhD in metallurgy from Rensselaer Polytechnic Institute, graduating in 1953.
Dr. Vermilyea was first employed as a metallurgist. He also taught metallurgy and electrochemistry at the Rensselaer Polytechnic Institute for a short time, before taking a position in the General Electric Company Research Laboratory in Niskayuna, New York, where he remained for 33 years, retiring in 1983. His areas of specialty were metallurgy and electrochemistry. He also spent several years as a key member of the technical planning team determining the focus areas for the Center.
Dr. Vermilyea loved the outdoors. He was a proud member and chapter head of the Adirondack Mountain Club, and had climbed all 46 of the Adirondack High Peaks several times. He was also the third
recorded Winter 46er, and guided two of his children to completion of the 46 as well. Upon his retirement he purchased 60 acres of woodland in Pattersonville and “went to work” there four days a week, creating a beautiful forest with many hand-planted trees.
In addition to his parents, Dave was predeceased by his loving wife of 64 years, Jane, and by his brother, Richard Vermilyea, who was killed in action during World War II. He was also predeceased by his sister, Jeanne Vermilyea Ackerman. He is survived by his sister, Laurie Wolfe, his two daughters, Carol and her husband Roger Cherney of Newton, New Jersey and Barbara and her husband Glenn Johnson of Shelburne, Vermont, and by his son Mark and wife Kathleen of Niskayuna. He is also survived by nine grandchildren and nine great-grandchildren. His family was a source of great pride and joy to him.
James Acheson (b. 1936) member since 1990, Dielectric Science and Technology Division
Michael Gulla (b. 1940) member since 1966, Electrodeposition Division
Albert R. Landgrebe (b. 1933) member since 1975, Energy Technology Division
George W. Luckey (b. 1925) member since 1968, Luminescence and Display Materials Division
Frederick Leon Marsh (b. 1935) member since 1956, Battery Division
Carl E. Mueller (b. 1939) member since 1969, Battery Division
Alex R. Nisbet (b. 1938) member since 1967, Physical and Analytical Electrochemistry Division
John P. Olatta (b. 1928) member since 1957, Luminescence and Display Materials Division
Thomas B. Reddy (b. 1933) member since 1960, Battery Division
Aiji A. Uchiyama (b. 1923) member since 1959, Battery Division
Perry N. Yocom (b. 1930) member since 1958, Luminescence and Display Materials Division
Interface is searching for People News for upcoming issues. If you have news you would like to share with the Society about a promotion, award, retirement, or other milestone event, please email the content to: kara.mcarthur@electrochem.org.
In this installment of the ‟Looking at Patent Lawˮ articles, we present a case study of a patented invention for an electrochemical cell for the concurrent electrolytic reduction of carbon dioxide and electrolytic oxidation (dehydrogenation) of hydrocarbons. The subject invention aligns with the research interest in sustainability matters by The Electrochemical Society (ECS) and its members. The case illustrates the use of a Request for Continued Examination (RCE) to continue prosecution of a patent application after a Final rejection by the United States Patent and Trademark Office (USPTO).
Recall from our previous article,1 the prosecution history of a patent application is publicly available in the file wrapper of the USPTO Patent Center.2 With the USPTO PAIR system as the primary source of information for this case study, we illustrate the prosecution “events” encountered during the examination of US Patent No. 11,788,193; “Electrochemical Cells and Electrochemical Methods.”3 The ‘193 patent issued on October 17, 2023 with inventor Gerardine G. Botte. Dr. Botte is currently a Professor at Texas Tech University where she holds the Whitacre Endowed Chair in Sustainable Energy and she is the Founder/Director of the NSF Engineering Research Center for Advancing Sustainable and Distributed Fertilizer Production (CASFER).4 The assignee of the patent at the time of issue was Ohio University, where Dr. Botte was previously the Russ Professor and Director of the Center for Electrochemical Engineering Research.
Dr. Botte is a long-time member of ECS and is a frequent presenter at ECS biannual meetings. Dr. Botte became a Chapter Fellow of the National Academy of Inventors in 2012 and was elected ECS Fellow in 2014. Dr. Botte is past chair of the Industrial Electrochemistry and Electrochemical Engineering (IE&EE) division and currently serves as ECS President.
The ‘193 patent abstract generally describes the invention as follows:3
“An electrochemical cell and method for reducing carbon dioxide and/or dehydrogenating a hydrocarbon to an olefin are provided. The electrochemical cell includes a cathode having a first conducting component that is active toward adsorption and reduction of an oxidizing agent such as CO2; and an anode having a second conducting component that is active toward adsorption and oxidation of a reducing
agent such as a hydrocarbon. Additionally, a hydrophobic modifier is present on at least a portion of a surface of the second conducting component or both the first and second conducting components. The method includes exposing the cathode to a CO2-containing fluid; exposing the anode to a hydrocarbon-containing fluid; and applying a voltage between the cathode exposed to the CO2-containing fluid and the anode exposed to the hydrocarbon-containing fluid, wherein the voltage is sufficient to simultaneously oxidize the hydrocarbon via a dehydrogenation reaction and reduce the CO2.”
This article summarizes the prosecution history of US Patent Application No. 15/570,848 leading to U.S. Patent No. 11,788,193. In Table I, we list the patent applications related to Patent Application No. 15/570,848. US Provisional Patent Application No. 62/157,103 was filed on May 5, 2015. A claim is not required in a provisional patent application5 and a provisional patent application expires one year from its filing date.6 Patent Cooperation Treaty (PCT) Application No. PCT/US2016/0299550 was filed on April 29, 2016. The PCT patent application was filed within one year of the filing date of the provisional patent application. Consequently, the priority date of the US utility and PCT patent applications is the filing date of the US provisional patent application; May 5, 2015.7 US divisional Patent Application No. 18/465,427 was filed September 12, 2023 prior to the issue of the ‘193 patent. The development team used the provisional patent application as a placeholder while additional experimental activities progressed. In addition, the prosecution of
(continued on next page)
the US Patent Application began on October 31, 2017 when the PCT patent application entered the US National Stage.
An applicant who files a PCT patent application delays the filing of the US utility patent application. There could be several benefits of this approach8
1) a delay in the time when papers must be submitted to the national (e.g., US) office;
2) an international search (to judge the level of the relevant prior art) and a written opinion on the question of whether the claimed invention appears to be novel, to involve an inventive step (to be non-obvious)…;
3) a delay in the expenditure of fees;
4) additional time for research;
5) additional time to evaluate financial, marketing, commercial, and other considerations; and
6) the option of obtaining international preliminary examination.
The BACKGROUND section of the patent application describes problems in the prior art related to electrochemical carbon dioxide reduction to value-added industrial chemicals. Specifically,
“…prior electrochemical methods of reducing CO2 are hampered with high-energy consumption (high operating voltage), low conversion to high value products, and low selectivity, which prevent the implementation of the process.”
The BACKGROUND section of the patent application further describes problems in the prior art related to conventional catalytic dehydrogenation of hydrocarbons to olefins. Specifically,
“The process is traditionally carried out at high temperatures and in the presence of a metal-based catalyst. …the catalyst is quickly and easily coked, and the period of time the catalyst is stable is limited…many catalysts cannot withstand many cycles of regeneration and heat integration without substantial loss of activity and selectivity. The ability of catalysts to promote selective reactions is also limited… and the share of thermal, nonselective reactions is often larger than desired.”
The BACKGROUND section concludes with a “need” statement,
“In view of the foregoing, there is a need for new electrochemical cells, as well as new electrochemical methods for reducing CO2, for the dehydrogenation of hydrocarbons to corresponding olefins, or combinations thereof.”
The DETAILED DESCRIPTION section of the patent application further describes the carbon dioxide reduction/hydrocarbon oxidation electrochemical invention as
“…a new process is provided that enables the concurrent oxidation of a hydrocarbon and the reduction of carbon dioxide (CO2) to high value products; the process may be called the “HYCO2chem process.” However, each of the halfreactions may be practiced independently, e.g., by substituting the hydrocarbon with a different reducing agent or by substituting CO2 with a different oxidizing agent.
Thus, in an embodiment, the HYCO2chem process includes an electrochemical cell designed with an architecture that will control the transport of the species required for the oxidation/reduction reactions.”
The only figure from the patent application is reproduced in Fig. 1. The FIGURE is a diagrammatic depiction of a simplified electrochemical cell 10 configured for flow cell processing. The simplified electrochemical cell 10 comprises a cathodic chamber 15 containing a cathode electrode 20, an anodic chamber 25 containing an anode electrode 30, wherein the cathodic chamber 15 and the anodic chamber 25 are physically separated from each other by a separator 35. However, while also serving as a physical barrier between the cathode electrode 20 and the anode electrode 30, the separator 35 allows the transport of ions between the anodic chamber 25 and the cathodic chamber 15. The cathode electrode 20 and the anode electrode 30 are configured with an electrical connection therebetween via a cathode lead 42 and an anode lead 44 along with a voltage source 45, which supplies a voltage or an electrical current to the electrochemical cell 10
The cathodic chamber 15 comprises an inlet 50 by which an oxidizing agent-containing fluid 11 enters and an outlet 55 by which reduction product(s) and unreacted oxidizing agent 12 exit. The oxidizing agent may include, but is not limited to, carbon dioxide, oxygen, molecular halogens, metal ions, protons, or combinations thereof. Similarly, the anodic chamber 25 comprises an inlet 60 by which a reducing agent-containing fluid 13 enters and an outlet 65 by which oxidation product(s) and unreacted reducing agent 14 exit. The reducing agent may include, but is not limited to, hydrogen, hydrocarbons, amines, alcohols, coal, pet-coke, biomass, lignin, or combinations thereof. Each of the cathodic and anodic chambers 15, 25 may further comprise gas distributors 70, 75, respectively. The electrochemical cell 10 may be sealed at its upper and lower ends with an upper gasket 80 and a lower gasket 85.
The patent application included four independent claims and two dependent claims. Independent Claim 1 and Independent Claim 2 are reproduced herein.
1. A method for electrolytically reducing carbon dioxide in an electrochemical cell comprising a cathode, an anode, and a separator, the method comprising: exposing the cathode comprising a first conducting component to a carbon dioxide (CO2)-containing fluid at a first pressure and first temperature, wherein the first conducting component is active toward adsorption and oxidation of CO2 and is selected from the group consisting of platinum (Pt), iridium (Ir), ruthenium (Ru),
palladium (Pd), rhodium (Rh), osmium (Os), nickel (Ni), cobalt (Co), iron (Fe), copper (Cu), and their combinations;
exposing the anode comprising a second conducting component to a reducing agent-containing fluid at a second pressure and a second temperature, wherein the second conducting component is active toward adsorption and reduction of a reducing agent; and
applying a voltage between the cathode exposed to the CO2-containing fluid and the anode exposed to the reducing agent-containing fluid so as to facilitate adsorption of the CO2 onto the cathode and adsorption of the reducing agent onto the anode; wherein the voltage is sufficient to simultaneously oxidize the reducing agent and reduce the CO2
2. A method for electrolytically dehydrogenating a hydrocarbon to an olefin in an electrochemical cell comprising a cathode, an anode, and a separator, the method comprising:
exposing the cathode comprising a first conducting component to an oxidizing agent-containing fluid at a first pressure and a first temperature, wherein the first conducting component is active toward adsorption and oxidation of an oxidizing agent;
exposing the anode comprising a second conducting component to a hydrocarbon-containing fluid at a second pressure and a second temperature, wherein the second conducting component is active toward adsorption and reduction of hydrocarbons via a dehydrogenation reaction, and wherein a hydrophobic modifier is present on at least a portion of a surface of the second conducting component; and
applying a voltage between the cathode exposed to the oxidizing agent-containing fluid and the anode exposed to the hydrocarbon-containing fluid so as to facilitate adsorption of the oxidizing agent onto the cathode and adsorption of the hydrocarbon onto the anode; wherein the voltage is sufficient to simultaneously oxidize the hydrocarbon via a dehydrogenation reaction and reduce the oxidizing agent.
Subsequent to submitting the patent application, the applicant submitted an inventor declaration signed by the inventor.9 The declaration included an assertion by the inventor stating,
“The above-identified application was made or authorized to be made by me.
I believe I am the original inventor… I have reviewed and understand the contents of the aboveidentified specification, including the claims,… I am aware of the duty to disclose to the United States Patent and Trademark Office all information know to me to be material to the patentability [of the subject patent application]…”
The declaration also included an acknowledgement that the inventor was aware of the penalties for a false statement,10
“I hereby acknowledge that any willful false statement in the Declaration is punishable under 18 U.S.C. 1001 by fine or imprisonment of not more than five (5) years, or both.”
Importantly, the “named inventor” must be correctly represented on a US patent application.11 Specifically, inclusion of a colleague as a co-inventor who did not participate in the conception of the invention is known as a misjoinder and may invalidate an otherwise valid patent. Similarly, exclusion of a co-inventor who participated in the conception is known as a nonjoinder and may invalidate an otherwise valid patent. If an inventor is erroneously omitted or erroneously included as an inventor, the misjoinder/nonjoinder may be corrected and the patent remains valid.12
To establish a filing date, a utility patent application must include
1) Specification13 “…a written description of the invention, and the manner and process for making it… to enable any person skilled in the art… to make and use [the invention] …”
2) Minimum of one claim14 “…particularly pointing out… the subject matter…as the invention…”
3) Drawings15 “…where necessary for understanding the subject matter…to be patented…”
To maintain the filing date, the following additional criteria are required
1) Filing fee in accordance with the current USPTO fee schedule;16
2) Inventor oath or declaration asserting17
a. The patent application was authorized by the inventor(s),
b. The inventor(s) believe he/she is the original inventor or they are the original joint inventors.
As summarized above, the specification included a background and summary of the invention describing various embodiments of the invention. The patent application also included a drawing illustrating the “elements” of the subject invention. The utility patent application contained claims directed toward the electrochemical method and cells along with the filing fee.
The filing of the patent application and the subsequently submitted declaration met the requirements to both establish and maintain a filing date and thereby avoid being abandoned. On Feb. 22, 2018, the USPTO issued a filing receipt and assigned the patent application no. 15/570,848.
(continued on next page)
The inventor was employed by Ohio University (OU) at the time of the invention and the patent application was assigned to OU.18 The patent application included a statement asserting “small entity” status as the assignee was a nonprofit university.19 The small entity status entitled the applicants to reduced filing, issue, and maintenance fees. In addition, the applicants appointed patent attorneys/agents from the law firm Wood Herron & Evans LLC to prosecute the patent application at the USPTO.
The applicants submitted an “Information Disclosure Statement” (IDS) to the USPTO with the patent application. Updated IDSs were submitted during the prosecution of the patent application. The IDS included prior art references, including those of the inventors as required by the “Duty of Candor.” The “Duty of Candor” requires that the inventor(s) submit an IDS within a reasonable time following the submission of the patent application disclosing20
“…to the Office [USPTO] all information known to that individual to be material to patentability…”
The “Duty of Candor” is specific to any existing claim and requires that the IDS be continually updated while the claim is pending. The “Duty of Candor” ceases only when the claim is allowed and the patent issue fee is paid.
The “Duty of Candor” extends to any individual associated with the filing of the patent application, including
1) Inventor(s),
2) Patent Counsel, or
3) Persons who are substantially involved in the preparation or prosecution of the patent application.
Substantial involvement in the preparation of the patent application could include technical assistants, collaborators, or colleagues. Substantial involvement would generally not extend to clerical workers. Furthermore, the inclusion of a reference in an IDS21
“…is not taken as an admission that the reference is prior art against the claims.”
If a finding of a violation of the “Duty of Candor” resulting in “inequitable conduct” regarding any claim in a patent is determined, then all the claims of the subject patent are rendered invalid.22 Finally, in spite of the requirement of the “Duty of Candor”, the applicant is cautioned not to “bury” the examiner with a long list of non-material references in hopes that the examiner will not notice the relevant material references.23 The specific guidance from the USPTO is to24
“…avoid the submission of long lists of documents if it can be avoided…If a long list is submitted, highlight those documents which have been specifically brought to the applicant’s attention and/or are known to be of most significance.”
The USPTO began publishing patent applications eighteen months after their priority date for patent applications filed on or after November 29, 2000. Since the subject patent application was filed after this date, it was published on May 31, 2018.
On August 20, 2019, the USPTO issued a restriction/election requirement stating that the subject patent application contains two or more inventions and the applicant must “elect” which invention to prosecute first25
“If two or more independent and distinct inventions are claimed in one application… [the USPTO] may require the application to be restricted to one of the inventions…”
The USPTO stated that the patent application contained four inventions:
Group I: Claim 1; drawn to a method for electrolytically reducing carbon dioxide in an electrochemical cell comprising a cathode, an anode, and a separator.
Group II: Claim 2; drawn to a method for electrolytically dehydrogenating a hydrocarbon to an olefin in an electrochemical cell comprising a cathode, an anode, and a separator.
Group III: Claim 3; drawn to a method for electrolytically reducing carbon dioxide and dehydrogenating a hydrocarbon to an olefin in an electrochemical cell comprising a cathode, an anode, and a separator.
Group IV: Claims 4–6; drawn to an electrochemical cell for reducing carbon dioxide.
On Oct. 21, 2019, the applicants “elected” to prosecute the invention associated with Group III Claim 1 (renumbered Claim 3). Along with the election, the applicants amended the claims to include three dependent claims. Inventions related to Group, I, Group II, and Group IV claims can be prosecuted subsequently in divisional patent applications subject to certain conditions. Specifically, the divisional patent application must26
1) contain at least one common inventor as the “parent” patent application, and
2) be filed while the “parent” patent application is being examined.
As noted in Table I, divisional patent application 18/465,427 was filed Sept. 12, 2023 during the pendency of the ‘193 patent which issued on Oct. 17, 2023.
On Nov. 6, 2019, the USPTO issued a non-final office action (NF-OA) rejecting all the claims in the patent application based on “indefiniteness”27
“…as being indefinite for failing to specifically point out and distinctly claim the subject matter which applicant regards as the invention.”
or as being “obvious” in view of the prior art28
“A patent may not be obtained… if the differences between the subject matter sought to be patented and the prior art are such that the subject matter as a whole would have been obvious at the time the invention was made to a person having ordinary skill in the art...”
The patent application was not rejected for lack of “novelty,” which is29
“…the invention was patented or described in a printed publication in this or a foreign country or in public use or on sale in this country, more than one year prior to the date of application for patent in the United States.”
The indefiniteness rejections were related to formalities and were easy to correct and overcome. The obviousness rejections were based on multiple prior art references and can be challenging to overcome. Generally, obviousness rejections are overcome by adding limitations from dependent claims or from the specification to the rejected claims.30
The applicants submitted amended claims and arguments to the USPTO with the intent of overcoming the obviousness rejections. On March 20, 2020, the USPTO issued a final office action again rejecting all of the claims based on obviousness. After the final rejection, on August 20, 2020, the applicants filed a Request for Continued Examination (RCE) along with the associated filing fees.
An RCE may be thought of as another “two bites of the apple.” In essence, an RCE is another filing of the patent application with amended claims. A RCE submission must include a filing fee31 and new arguments of patentability. In support of patentability arguments, the RCE may include32
1) an information disclosure statement,
2) an amendment to the written description, claims, or drawings,
3) new arguments, or
4) new evidence in support of patentability.
An RCE must be filed prior to the earliest of33
1) payment of the issue fee,
2) abandonment of the patent application,
3) filing of a notice of appeal.
And finally,
“If an applicant timely files and submission and fee…, the Office [USPTO] will withdraw the finality of any Office [USPTO] action and the submission will be entered and considered.”
By filing the RCE, the applicant extended the prosecution of the patent application. The sequence of non-final/final rejections and RCEs associated with the subject patent application are presented in Table II. During the progression of Non-Final/Final rejections and RCEs, the applicants submitted amended claims with successively more restrictive limitations. Although the applicants were able to
overcome some of the prior obviousness rejections, the USPTO continued to reject the claims as obvious based on the prior art. The last Final rejection occurred on November 10, 2022.
On April 21, 2023, the applicant submitted a fourth RCE with a further amendment to independent Claim 1 to include all elements of Claim 2 as well as additional content from some of the other claims and content supported by the specification. The amended Claim 1 is reproduced herein with the inserted text depicted in [brackets].
1. A method for electrolytically reducing [a] carbon dioxide [and dehydrogenating a hydrocarbon to an olefin] in an electrochemical cell comprising [an anode,] a cathode, an anode, and a separator, the method comprising:
exposing the cathode comprising a first conducting component to a carbon dioxide (CO2)-containing fluid [comprising a first nanoparticle to a first fluid containing the carbon dioxide (CO2)] at a first pressure and first temperature, wherein the first conducting component is active toward adsorption and oxidation of CO2 and [the CO2, and the first conducting component] is selected from the group consisting of platinum (Pt), iridium (Ir), ruthenium (Ru), palladium (Pd), rhodium (Rh), osmium (Os), nickel (Ni), cobalt (Co), iron (Fe), copper (Cu), and their combinations;
exposing the anode comprising a second conducting component to a reducing agent-containing fluid [second fluid containing the hydrocarbon] at a second pressure and a second temperature, wherein the second conducting component is active toward adsorption and reduction of a reducing agent; and
applying a voltage between the cathode exposed to the CO2-containing fluid and the anode exposed to the reducing agent-containing fluid so as to facilitate adsorption of the CO2 onto the cathode and adsorption of the reducing agent onto the anode; wherein the voltage is sufficient to simultaneously oxidize the reducing agent and reduce the CO2.
2. A method for electrolytically dehydrogenating a hydrocarbon to an olefin in an electrochemical cell comprising a cathode, an anode, and a separator, the method comprising:
exposing the cathode comprising a first conducting component to an oxidizing agent-containing fluid at a first pressure and a first temperature, wherein the first conducting component is active toward adsorption and oxidation of an oxidizing agent;
exposing the anode comprising a second conducting component to a hydrocarbon-containing fluid at a second pressure and a second temperature, wherein the second conducting component is active toward adsorption and reduction of hydrocarbons via a dehydrogenation reaction, and wherein a hydrophobic modifier is present on at least a portion of a surface of [the hydrocarbon, the second conducting component is selected from the group consisting of platinum (Pt), iridium (Ir), ruthenium (Ru), palladium (Pd), rhodium (Rh), nickel (Ni), cobalt (Co), iron (Fe), copper (Cu), and their combinations, the second conducting component being layered on an anode substrate surface, an overlying layer of the second conducting component incompletely covering an underlying layer of the second conducting component, and wherein a hydrophobic modifier comprising an electrochemically reduced graphene oxide coating is present on both the first conducting component and] the second conducting component; and
Taylor and Inman
applying a voltage between the cathode exposed to the oxidizing agent-containing [first] fluid and the anode exposed to the hydrocarbon-containing [second] fluid so as to facilitate adsorption of the oxidizing agent [CO2] onto the cathode and adsorption of the hydrocarbon onto the anode;[,] wherein the voltage is sufficient to simultaneously oxidize the hydrocarbon [to a first olefin] via a dehydrogenation reaction and reduce the oxidizing agent [CO2 to form a second olefin].
On June 13, 2023, the USPTO issued a notice of allowance. The amended independent Claim 1 allowed the applicants to overcome the “obviousness” rejections. Additionally, all the dependent claims were allowed. After payment of the issue fees, the patent application issued as US Patent No. 11,788,193 on October 17, 2023.
In this installment of our “Looking at Patent Law” series, we present a case study of the prosecution of US Patent No. 11,788,193; “Electrochemical Cells and Electrochemical Methods.” The ‘193 patent issued on October 17, 2023 with inventor Gerardine G. Botte. The assignee of the patent at time of issue was Ohio University (OU). The case study begins with a brief synopsis of the background of the invention followed by 1) summary of the drawing and the specification of the invention, 2) inventor assignment and power of attorney designations, 3) submission of the Invention Disclosure Statement (IDS) and associated Duty of Candor, 4) summary of the multiple non-final/final office actions rejecting the patent application, and 5) applicant response and allowance of the patent application. The case study illustrates the use of Request for Continued Examination (RCE) to continue the prosecution of the subject patent application. With this case study, we hope to de-mystify the patent prosecution process and better prepare electrochemical and solid-state scientists, engineers, and technologists to interact with their patent counsel regarding their inventions.
©The Electrochemical Society. DOI: 10.1149/2.F05241IF
E. Jennings Taylor, Founder of Faraday Technology, Inc.
Research Interest: Founder of Faraday Technology, Inc., a small business focused on developing innovative electrochemical processes and technologies based on pulse and pulse reverse electrolytic principles. Until his recent retirement, Taylor led Faraday’s patent and commercialization strategy and negotiated numerous patents via field-of-use licenses as well as patent sales. He is admitted to practice before the United States Patent & Trademark Office (USPTO) in patents cases as a patent agent (Registration No. 53,676). He is a Member of the American Intellectual Property Law Association (AIPLA).
Pubs + Patents: Numerous technical pubs and presentations, inventor on 40 patents.
Maria Inman, Vice President, Faraday Technology, Inc.
Work Experience: Dr. Inman manages Faraday Technology’s pulse and pulse reverse research project portfolio and business development activities.
Pubs + Patents: >108 publications and 7 patents.
Work with ECS: Member for 27 years, Chair of the IE&EE Division.
Website: http://www.faradaytechnology.com/ https://orcid.org/0000-0003-2560-8410
1. E. J. Taylor and M. Inman, Electrochem Soc Interface 26(4), 57 (2017).
2. USPTO Patent Center, http://patentcenter.uspto.gov
3. G. G. Botte “Electrochmical Cells and Electrochemical Methods” U.S. Patent No. 11,788,193 issued October 17, 2023.
4. G. G. Botte, Electrochem Soc Interface, 32(3) 69 (2023)
5. 35 U.S.C. §111(b)(2) Provisional Application/Claim.
6. 35 U.S.C. §111(b)(5) Provisional Application/Abandonment.
7. 35 U.S.C. §119(a) Benefit of Earlier Filing Date; Right of Priority.
8. 35 U.S.C. 371 National Stage: Commencement Statements or Entries Generally.
9. 37 CFR 1.63 Inventor’s Oath or Declaration.
10. 18 U.S.C. §1001Statements or Entries Generally.
11. E. J. Taylor and M. Inman, Electrochem. Soc. Interface, 26(2), 45 (2017)
12. Manual of Patent Examination Procedure (MPEP) §1481.02 Correction of Named Inventor.
13. 35 U.S.C. §112(a) Specification/In General.
14. 35 U.S.C. §112(b) Specification/Conclusion.
15. 35 U.S.C. §113 Drawings.
16. https://www.uspto.gov/learning-and-resources/fees-andpayment/uspto-fee-schedule#Patent%20Fees
17. 35 U.S.C. §115(b)(1)(2) Inventor’s Oath or Declaration/Required Statements.
18. 35 U.S.C. §261 Ownership; Assignment.
19. 37 CFR §1.27(a)(3)(ii)(A) Definition of small entities and establishing status as a small entity to permit payment of small entity fees; when a determination of entitlement to small entity status and notification of loss of entitlement to small entity status are required; fraud on the Office.
20. 37 CFR §1.56(a) Duty to Disclose Information Material to Patentability.
21. Riverwood Int’l Corp. v. R.A. Jones & Co., 324 F.3d 1346, 135455, 66 USPQ2d 1331, 1337-38 (Fed Cir. 2003).
22. Manual of Patent Examination Procedure (MPEP) §2016 Fraud, Inequitable Conduct, or Violation of Duty of Disclosure Affects All Claims
23. R. B. Taylor, Mich Telecom & Tech Law Rev, 19, 99 (2012).
24. Manual of Patent Examination Procedure (MPEP) §2004.13 Aids to Comply with Duty of Disclosure.
25. 35 U.S.C. §121 Divisional Applications.
26. 35 U.S.C. §120 Benefit of Earlier Filing Date in the United States
27. 35 U.S.C. §112(2) (pre-AIA) Specification.
28. 35 U.S.C. §103(a) (pre-AIA) Conditions for Patentability; Nonobvious Subject Matter.
Work with ECS: Member for 42 years, ECS Fellow. Website: http://www.faradaytechnology.com/ https://orcid.org/0000-0002-3410-0267
29. 35 U.S.C. §102(b) (pre-AIA) Conditions for Patentability; Novelty and Loss of Right to Patent.
30. E. J. Taylor and M. Inman, Electrochem Soc Interface, 26(3), 39 (2017)
31. 37 CFR §1.17(e) Patent Application and Reexamination Processing Fees
32. 37 CFR §1.114(c) Request for Continued Examination
33. 37 CFR §1.114(a) Request for Continued Examination
BioLogic USA/BioLogic SAS (16*)
Duracell US Operations, Inc. (67)
Gamry Instruments (17)
Gelest Inc. (15)
Hydro-Québec (17)
PalmSens BV (1)
Pine Research Instrumentation (18)
Energizer Battery (79)
Faraday Technology, Inc. (18)
GE Global Research Center (72)
Lawrence Berkeley National Laboratory (LBNL) (20)
Scribner, LLC (28)
Toyota Research Institute of North America (TRINA) (16)
BASi (9)
Center for Solar Energy and Hydrogen Research Baden-Württemberg (ZSW) (20)
Central Electrochemical Research Institute (31)
Corteva Agriscience (2)
DLR – Institute of Engineering Thermodynamics (16)
EL-CELL GmbH (10)
Electrosynthesis Company, Inc. (28)
Ford Motor Company (10)
GS Yuasa International Ltd. (44)
Honda R&D Co. (17)
Medtronic, Inc. (44)
Nel Hydrogen (2)
Nissan Motor Co., Ltd. (17)
NSF Center for Synthetic Organic Electrochemistry (3)
Pacific Northwest National Laboratory (PNNL) (5)
Panasonic Energy Corporation (29)
Permascand AB (21)
Plug Power, Inc. (3)
Teledyne Energy Systems, Inc. (25)
UL Research Institutes (3)
Advanced Cell Engineering (3)
Cummins, Inc. (6)
Current Chemicals (2)
General Motors Holdings LLC (72)
Giner, Inc. (38)
Ion Power, Inc. (10)
John Cockerill Hydrogen S.A. (1)
Kanto Chemical Co., Inc. (12)
Los Alamos National Laboratory (LANL) (16)
Metrohm USA, Inc. (10)
Microsoft Corporation (7)
Occidental Chemical Corporation (82)
Sandia National Laboratories (48)
Sensolytics GmbH (1)
Sherwin-Williams (3)
Spectro Inlets ApS (2)
Technic, Inc. (28)
United Mineral & Chemical Corporation (3)
Western Digital (10)
Westlake Corporation (29)
Yeager Center for Electrochemical Sciences at CWRU (26)
Practical electrodes for commercial Li-ion batteries require higher mass loading, thicker electrodes than are typically reported for lab scale research. It is well known that increasing electrode thickness results in degradation of rate performance. Consequently, high areal capacities are only achievable at lower rates for thicker electrodes. This leads to a trade-off when attempting to maximize capacity and rate capability. To examine the challenges associated with this balancing, researchers from Trinity College Dublin have investigated how increases in specific or volumetric capacity may directly affect rate performance. The influence of volumetric capacity on rate performance was determined through electrochemical testing of graphite/ boron nitride/carbon nanotube composites with varying compositions. The rate performance of these cells was determined by fitting the relationship between volumetric capacity and rate to a semi-empirical equation and extracting a characteristic charge/discharge time. This characteristic time was found to increase linearly with volumetric capacity, suggesting a detrimental relationship between volumetric capacity and rate performance. This report highlights the challenges faced when attempting to optimize electrochemical performance of electrodes with practical mass loadings and thicknesses.
From: D. V. Horváth, V. Nicolosi,and J. N. Coleman, J. Electrochem. Soc., 170, 090518 (2023).
Modeling electrochemical systems typically involves using systems of coupled partial differential equations (PDEs), likely needing specialized software to solve relevant problems. Thus, publicly funded research often results in models formulated using commercial software tools, potentially rendering findings inaccessible to the wider community. Researchers at Georgia Tech have published a wide-ranging demonstration of open-source simulation tools based on the Python programming language, applied to a series of electrochemical boundary value problems. The problems chosen involve domains or boundaries with irregular shapes, for which straightforward solution methods were not previously available. The authors guide the reader through four examples solved using FEniCSx (finite element computational software), a popular opensource platform for solving PDEs. Current distributions are solved for (a) a Hull cell with a central insulator; (b) a battery active material particle in a domain of comparable size; (c) conduction through a surface with insulating segments; and (d) conduction through a surface with contact area loss. All of these are relevant to modern problems such as anode-free Li metal batteries. Models
are provided on a GitHub repository to guide the reader and help researchers make use of open-source software tools.
From: E. L. Molel and T. F. Fuller, J. Electrochem. Soc., 170, 103501 (2023)
Validating Electrochemical Active Surface Area Determination of Nanostructured Electrodes: Surface Oxide Reduction on AuPd Nanoparticles Gold palladium nanoparticles (NPs) are studied and employed for their high catalytic activities toward important reactions in fuel cell and other electrochemical applications. Proper characterization of these electrocatalysts depends on accurate determination of the electrochemically active surface area (ECSA). Researchers in Germany employed loading determination along with ECSA evaluation via surface oxidation reduction to demonstrate an approach that is accurate and reliable. Using AuxPdy NPs of known size and composition drop cast on a glassy carbon (inert) electrode, they performed ECSA measurements followed by anodic stripping voltammetry to calculate a NP diameter that is compared against TEM imaging. Critical to their approach development was obtaining NPs of regular spherical shape and narrow distribution size, which was achieved by using ~10% of Au or Pd in the shell alloy on the core Au seed particle. The ECSA data depended on the maximum potential (vertex) to which it was scanned. Several different Emax values were used to collect a range of calculated diameters, which were compared against the TEM analysis for each NP composition. Thus, using this approach for an unknown NP size requires employing a specified Emax for a known (or expected) composition. Different electrolytes and pH conditions were also explored during the investigation of suitable experimental conditions.
From: P. Cignoni, P. Hosseini, C. Kaiser, et al., J. Electrochem. Soc., 170, 116505 (2023).
Electrochemical Sensors Based on Metal-Organic Framework and Conductive Polymer HKUST-1@PANI for High-Performance Detection of Lead Ions Lead is a highly toxic heavy metal that is prevalent throughout the world. Detection of lead ions is of high interest, and numerous types of sensing techniques, such as spectroscopy and optical-based methods have been developed for heavy metals. However, these types of detection can be costly, complex, and have long detection times. Researchers at the University of Monastir recently demonstrated an electrochemical sensor for the detection of lead, based on a materials platform of a metal-organic framework (MOF), HKUST-1, loaded with a conductive polyaniline polymer, PANI. MOFs are known for their high selectivity to heavy metals but have low conductivity. Polyaniline polymers, however, have high conductivity and are known to be stable in water. By combining these attributes
in an HKUST-1@PANI composite-based electrochemical sensor, the researchers demonstrated an inexpensive, durable-inaqueous-solutions, and sensitive detection technique for lead. Differential pulse stripping voltammetry results showed the ability to detect concentrations in the range of 0.005 to 50 μM, with a minimum detection limit of 5 nM. Preliminary results also demonstrated that interference from other possible coexisting metal ions was minimal.
From: J. Wannassi, N. Missaoui, C. Mabrouk, et al., J. Electrochem. Soc., 170 117504 (2023).
Physics-Integrated Machine Learning for Efficient Design and Optimization of a Nanoscale Carbon Nanotube Field-Effect Transistor
The move to sub-10 nm scale technology node results in an increase in tunneling leakage current. One way to address this is to use carbon nanotubes. To efficiently transfer this technology, which involves multiple design variables, into mass production, researchers from Shanghai Jiao Tong University in China came up with a physics-based closed-loop design framework that integrated machine learning and multi-objective optimization. Starting with a calibrated technology computer-aided design (TCAD) model, the team performed statistical analyses to establish the correlation matrix between the design parameters and metrics. The correlation matrix helped guide the order of the support vector regression (SVR) model. In addition to SVR, they also evaluated a shallow learning method, random forest (RF), and a deep learning method, neural network (NN). The research team found that, with a data-scarce model, SVR performed better than RF and NN. They also found an unusual trend in the device performance and postulated a physicsbased hypothesis for the observed behavior. The machine learning model was then used to perform multi-objective optimization, which helped them to narrow the design space.
From: G. Fan and K. L. Low, ECS J. Solid State Sci. Technol., 12, 091005 (2023)
Tech Highlights was prepared by Joshua Gallaway of Northeastern University, Mara Schindelholz of Sandia National Laboratories, David McNulty of University of Limerick, Chock Karuppaiah of Vetri Labs and Ohmium International, and Donald Pile of EnPower, Inc. Each article highlighted here is available free online. Go to the online version of Tech Highlights in each issue of Interface, and click on the article summary to take you to the fulltext version of the article.
It is no surprise that the 244th ECS Meeting in Fall 2023 took place in Gothenburg, Sweden, which is ranked as the most sustainable city in the world. At the meeting, ECS President Gerri Botte proclaimed “ECS Toward Sustainability.”1 She articulated a vision for ECS to build off its past successes to enable a more sustainable society. However, it is not so easy to define sustainability— partly because of how large and encompassing it is. Unequivocally, ECS has been the most important professional society in advancing electrochemical energy conversion and storage technologies with the ultimate aim of decarbonization and curtailing the deleterious impacts of climate change. However, the adoption of clean energy is only one facet of sustainability. There are several other topics and global challenges that fall under the umbrella of sustainability (e.g., securing clean and potable water supplies, the production of food, chemicals, and materials with minimal environmental impact, and the removal of existing pernicious elements (for example CO2) from the environment).
This issue of Interface includes three articles that articulate the importance of electrochemical separations for sustainability. The articles emphasize electrochemical separations for industrial applications, such as gas purification (e.g., CO2 concentration, hydrogen purification, and ammonia compression), critical minerals refining, and nutrient and organic acid recovery. Electrochemical separations are a powerful tool for securing critical minerals and materials for the growing electrification of energy production and transmission while being more environmentally friendly (e.g., using renewable electrons and less solvent). For nutrient recovery, it is meaningful to capture and recycle phosphate from waste streams, as we are diminishing our phosphorus rock reserves. Plus, the energy intensive Haber-Bosch process contributes significantly to CO2 emissions. Recovering nitrate from wastewater and transforming nitrate back into ammonia can reduce the need for virgin ammonia from the Haber-Bosch process and the CO2 footprint for manufacturing fertilizers. Although water purification is an important sustainability topic in which electrochemical processes play an important role, water desalination by electrodialysis and other electrochemical platforms is not the focus of the articles in this issue due to the maturity of this technology.
It is worthwhile to note that ECS has organized the recurring symposium “Electrochemical Separations and Sustainability” at the Fall ECS Meeting for the past five years. The Journal of the Electrochemical Society published a Focus Issue on Electrochemical Separations and Sustainability in 2022.2 The topic of this Interface issue was born out of all these prior meaningful endeavors.
The first article in this Interface issue is a Chalkboard article by Arges. This article provides the various applications that currently use (or could benefit from) electrochemical separations. It also conveys the major electrochemical separations platforms, and the modes of action that enable selective separations. The next article, by Stracensky and Xu, discusses electrochemical gas separations integrated with green energy. This article highlights hydrogen, carbon dioxide, and ammonia separations using the concept of low-temperature and hightemperature electrochemical pumps. There are great opportunities and pending challenges for the viable application of electrochemical gas separations.
The final article, by Akolkar and co-workers, presents chloride molten salt electrolysis (MSE) to produce neodymium metal—a key component in permanent magnets found in electric motors for electric vehicles and wind turbines. The authors also discuss how the chloride molten salt electrolyte can be used to separate neodymium from used neodymium magnets (i.e., NdFeB).
We hope these articles motivate readers to work in the field of electrochemical separations. We believe the problems electrochemical separations can address are diverse. We envision that electrochemical separations will enable sustainability in food and critical minerals and removal of CO2 from the atmosphere, while also assisting in shoring up supply chains that facilitate a clean energy infrastructure.
©The Electrochemical Society. DOI: 10.1149/2.F07241IF
Christopher G. Arges, Research Engineer, Transportation and Power Systems Division, Argonne National Laboratory
Education: BS (University of Illinois at Urbana-Champaign), MS (North Carolina State University), and PhD (Illinois Institute of Technology) in Chemical Engineering; Postdoc (University of Chicago/Argonne National Laboratory) in Molecular Engineering
Research Interests: Ion-exchange membranes, Block copolymer self-assembly, Electrochemical separations, Fuel cells, Electrolysis
Work Experience: Argonne National Laboratory from 2023–current, Associate Professor in Chemical Engineering at Pennsylvania State University from 2021 to 2023, Assistant Professor in Chemical Engineering at Louisiana State University from 2016 to 2021. Pubs + Patents: 65 peer-reviewed publications, h-index 30, 60 invited talks, 5 ECS Interface articles, 6 papers in Journal of the Electrochemical Society
Honors & Awards: NSF CAREER, ECS Toyota Young Investigator Fellowship, 3M Non-Tenured Faculty Award, Invited Speaker for 2023 EU-US Frontiers of Engineering Symposium, Journal of Materials Chemistry Emerging Young Investigator
Work with ECS: 14 years of ECS membership, Session Organizer for IE&EE Division, Member at Large for IE&EE Division and Energy Technology Division
https://orcid.org/0000-0003-1703-8323
Hui Xu, Envision Energy USA
Education: BS in Chemical Engineering (Wuhan Institute of Technology), PhD in Chemical Engineering (University of Connecticut).
Research Interests: Electrolysis and fuel cells
Pubs + Patents: >50 papers, h-index: 39, 5 patents.
Awards: Key Contributor to 2021 Special Recognition Award from DOE H2 Program Annual Merit Review Meeting; Inducted into the University of Connecticut Academy of Distinguished Engineers (2020). Co-founder of Gordon Research Conference-Water Electrolysis to be launched in 2025.
Work with ECS: Energy Technologies Division Treasurer; Guest editor for three JES special focus issues
https://orcid.org/0000-0001-6829-7187
1. Highlights of the 244th ECS Meeting, Electrochem Soc Interface, 32, 12 (2023).
2. Focus Issue on Electrochemical Separations and Sustainability, J Electrochem Soc, 169 (2022).
Chemical separations are a cornerstone of industrial manufacturing processes that generate products and services that have improved the standard of living for humans across the globe. To give some context as to how ubiquitous separations are in the modern world, they are involved in the production of fuels, medicines, clean water, fertilizers, materials used in semiconductor chip manufacturing, and other goods. A 2019 report by the National Academies of Sciences, Engineering, and Medicine highlights that chemical separations account for about 10 to 15% of energy use in the United States.1 Of the four broad sectors (residential, transportation, industry, and commerce) that use energy in the United States, industry has the largest use at 32% and about half of the energy use in industry hails from separations.2 It is likely that the transportation and residential sectors will experience significant decarbonization in the next 25 years with the proliferation of wind and solar energy sources coupled with electrochemical energy storage and electrification of vehicles. Industrial decarbonization, on the other hand, is far more complex and challenging and it is imperative that future engineers and scientists work hard to devise alternative processes that can be powered on renewable electrons while generating little waste to produce the goods and services that make up our modern lives.
Chemical processes encompass two basic components: (i) the transformation of feedstocks to value-added products and (ii) the purification of individual products from mixtures that contain other products and unreacted reactants. It can be argued that the first basic component is simpler because it involves only a reactor(s) (batch, continuously stirred, plug-flow) and a catalyst to carry out the chemical transformation. Separations, on the other hand, often contains several unit operations, which may include distillation columns, flash units, adsorption/absorption columns with packings, and membranes. There has been a renaissance in the ECS community over the past 10 to 15 years in using electrolysis as an alternative to make commodity chemicals (e.g., ethylene from CO2 waste product3 and nitrate to make ammonia4) and specialty chemicals (e.g., Birch reduction to make pharmaceutical precursors).5 Within the past seven years, tremendous progress has also been made in the field of electrochemical separations for critical minerals recovery6 and recycling,7 purification of organics from process biomass,8 nutrient recovery,9,10 and gas separations.11–13
It is worth noting that electrodialysis and electrodeionization, both of which are electrochemical separations processes, are well established and commercialized for desalination and the production of ultrapure water used in manufacturing—such as semiconductor and pharmaceutical manufacturing.14,15 The present and future of electrochemical separations focuses on targeted removal of desired species or bad actors in complex mixtures. This challenge is contrary to conventional electrodialysis and deionization processes that aim to remove all ions from the liquid to make potable water or ultrapure water. Hence, this article does not focus on electrochemical separations for water desalination, as that topic is mature.
This Chalkboard article commences with a brief summary of the notable applications that currently use or could benefit from electrochemical separations. This section also introduces some basic system architectures, such
as electrodialysis, electrodeionization, electrochemical gas pumps, electrocoagulation, and electrosorption platforms (e.g., membrane capacitive/battery electrode deionization), used in electrochemical separations. Next, the article covers the modes of action that enable separations in various electrochemical platforms. The article closes with common expressions that inform the performance and design of electrochemical separation units. It is important to note that this article primarily focuses on low-temperature and intermediatetemperature electrochemical separation platforms (< – 250 °C).
As previously stated, electrochemical separations have garnered significant attention in recent years for securing critical minerals and materials (CMMs),6 CO2 purification11 and direct air capture (DAC) and CO2 removal from ocean water,16,17 purifying hydrogen from reformed hydrocarbon mixtures13 and natural gas mixtures,18 ,
recovering organic acids from processed biomass,8,19 and facilitating nutrient recovery.9,10 Figure 1 conveys the various applications that can use electrochemical separations. Figure 2 depicts different types of electrochemical separations platforms that can be deployed for various applications. Although Fig. 2 shows electrodialysis and electrodeionization performing desalination, these processes have also been deployed for critical minerals recovery, organic acid recovery,8,19 and nutrient recovery.9,10
a)
b) Electrodeionization Electrodialysis
c) Shock electrodialysis
e) Electrosorption with no membranes
g) Electrochemical pumps
h) Electrocoagulation
(ii) lithium, cobalt, and nickel for batteries; (iii) rare-earth elements (REEs), such as neodymium and dysprosium for magnets used in wind farms; (iv) gallium for the electronics industry; and (v) platinum group metals for green hydrogen production that will help decarbonize heavy-duty vehicle transportation, steel manufacturing, and fertilizer production.20 Electrodialysis and electrosorption platforms have been demonstrated for CMM capture and purification.21,22
Regarding nutrient recovery, lowering the burden on phosphorus supplies and decarbonizing fertilizer manufacturing (e.g., the HaberBosch process) will require recovery of nitrate and phosphate from waste streams such as agricultural runoff.23,24
d) Electrodeposition
f) Electrosorption with membranes
Because we do not have the technologies today to decarbonize every industrial process, such as cement manufacturing, CO2 capture at point sources and DAC will need to be a part of our technology portfolio to meet decarbonization goals by 2050. There are a multitude of electrochemical processes that purify CO2 directly via capture in electrodes (e.g., functionalized with poly-1,4anthraquinone),16 the use of molten carbonate fuel cell,25 and in-situ pH adjustment that generates an alkaline solution to form carbonate or bicarbonate products.17,26 The carbonate/bicarbonate species can be reconverted back to CO2 by changing the pH to be acidic.27 This latter step results in a concentrated CO2 stream that can undergo storage or utilization. The pH adjustment in electrochemical cells can occur with a bipolar membrane28 or via faradaic reactions29 on an electrode (e.g., oxygen reduction reaction (ORR) with water to generate hydroxide), water reduction reaction to generate hydroxide (i.e., hydrogen evolution reaction (HER) in base), hydrogen oxidation reaction (HOR) in acid to generate protons, and water oxidation to generate acid (i.e., the oxygen evolution reaction (OER) in acid)). It is beneficial to perform in-situ pH adjustment because the chemical processes for producing hydrochloric acid and sodium hydroxide, which are the most common acid and bases used for pH adjustment, hail from the energy-intensive chlor-alkali process (note: hydrochloric acid comes from reacting hydrogen with chlorine).
Regarding hydrogen, every decarbonization roadmap prominently features clean hydrogen to meet global decarbonization goals because it can power heavy-duty vehicle transportation,30 such as long-haul trucking, aviation, rail, and marine shipping, in addition to providing seasonal/longterm energy storage,31 and reducing greenhouse gas emissions from industry.23,32 In the latter example, clean hydrogen can be used as a reducing agent for making steel,3,32 as opposed to CO which is produced from fossil fuel sources, and it can be used in the production of fertilizers.23 Most hydrogen used in the Haber-Bosch process to make ammonia and fertilizers is gray—meaning it hails from reformed methane with little to no mitigation of methane and the CO2 emissions. Green hydrogen today has high production costs, $4 to $6 per kg, but there is a concerted effort to reduce those costs to $1 to $2 per kg of green hydrogen produced in the next 5 to 10 years. Producing hydrogen via steamreforming methane is about ~$2 per kg. Equally important to reducing green hydrogen costs is alleviating costs associated with the transportation and storage of hydrogen—which are on par with green hydrogen's current production costs. It has been proposed to blend hydrogen with natural gas for transportation via the natural gas pipeline
Critical minerals are vital for the growth of the electrification revolution, which will require more (i) copper for transmission lines; (continued on next page)
Arges (continued from previous page)
to lower hydrogen delivery costs.18 Gas pipelines can be the most cost-effective transportation route for gas delivery. Pure hydrogen cannot be transported in the natural gas pipeline because it embrittles the pipeline material. Rather, it must be diluted with natural gas. Electrochemical hydrogen pumps have been proposed to purify hydrogen from natural gas mixtures at end-user facilities.18 Purification is required when using hydrogen as a fuel in fuel-cell vehicles or in chemical manufacturing. One advantage of an electrochemical hydrogen pump is that it can simultaneously perform separation and compression of the purified gas (e.g., compression up to 1000 bar has been achieved).33 Furthermore, an electrochemical hydrogen pump can purify hydrogen from reformed hydrocarbons where the separated CO2 and CO from the hydrogen can then be sequestered or utilized to make other value-added products such as plastics and surfactants.13 It is also worth noting that an electrochemical hydrogen pump can be used to purify and compress ammonia when using hydrogen as a carrier gas (e.g., separating ammonia and hydrogen from nitrogen in a mixture; the ammonia can be separated from hydrogen via cooling and condensation of the ammonia).34
As part of the circular economy, it has been proposed to use biomass resources to generate hydrocarbon fuels and feedstocks used in chemical manufacturing.35 Plants and other biological systems uptake CO2 and use it as a carbon source to generate biomass. Chemical processes, such as fermenters and digestors, convert biomass into value-added chemicals such as methane and organic acids. Electrodialysis, electrodeionization, and membrane capacitive deionization have all been demonstrated to purify organic acids from model product mixtures that emanate from bioreactors.19,28,36 Notably, anion exchange membranes containing tethered 1-methyl imidazolium groups have been shown to promote the flux and selectivity of lactate and p-coumarate in electrodialysis and electrodeionization, respectively.19,36 Lactate anions can be polymerized to generate poly(lactide), an alternative and more sustainable plastic than poly(olefins). p-coumaric acid is a precursor used to synthesize pharmaceutical products that treat cancer and anxiety.
There are a multitude of actions that can be exploited to enable selective separations in electrochemical platforms. Effective selective separations can be enabled by advanced materials and/or by engineering the architecture and operating parameters of the electrochemical separation platform. Figure 3 highlights several modes of action that enable selective separations.
Given that all electrochemical cells are composed of two electrodes and an electrolyte that separates the two electrodes, it is not unexpected that electrode materials and electrolyte membrane materials are engineered to foster the efficacy and efficiency of selective separations. Hence, the modes of separations enabled by materials are first discussed.
Suss and Elimelech and co-workers showed that carbon, non-carbon, and intercalating electrodes can be tuned to exploit pore size, ionic mobility, hydration energy/ratio, and chemical affinity to enable selective separations.37 In other instances, the electrode’s chemistry and potential can be used cooperatively to enable a selective separation via electrodeposition, intercalation, or adsorption into the electrochemical double layer.38 Regarding intercalation, Liu and coworkers used TiO2-coated FePO4 electrodes with a pulse-rest-reverse-pulse-rest potential cycle to
extract lithium selectivity (50:1 over sodium) from model solutions.39 For rare-earth elements such as dysprosium, terbium, europium, neodymium, and yttrium, electrodeposition is an effective approach for separation based upon differences in the reduction potential for some of the elements.6 If a pure metallic form of the rare-earths is preferred, it is necessary to use non-aqueous electrolytes, such as eutectic electrolytes at high temperature40 or ionic liquids,41 because the rare-earth element reduction potentials are more negative than the water reduction potential. However, generating hydroxide ions from water reduction in the presence of rare-earth element cations can lead to rare-earth element oxides on the electrode during electrodeposition.6 Another notable electrode strategy for selective separations is the use of redox active polymers that change their oxidation/charge state based upon the oxidation/reduction potential of the polymer.42 The positively charged polymer removes anions via electrostatic interactions. Altering the other chemical moieties in the redox polymer can exploit other interactions, like van der Waal interactions, to assist in the selective removal of species (e.g., removal of short-chain perfluoroalkyl substances).43
Ion-exchange membranes are another important class of materials that can enable selective electrochemical separations. However, ion-exchange membranes generally do not have good counterion “i” selectivity over other counterions—especially if the counterions have the same valence.44 Conventional ion-exchange membranes (i.e., without modification) tend to have a slightly higher affinity for divalent ions over monovalent ions provided the divalent ions are
Modes of action for e-chem separations
a)Electrode materials
a1) Electrode properties
Electrode
size
• Hydration energy/extent
• Chemical affinity
• Ion mobility differences
a2) Redox active polymers
Electrode support
• Oxidation state
• Solvation
• Molecular interactions
• Electrostatic
• Dispersion
• Hydrogen bonding
b)Electrodeposition
Electrode support
or AOx
• Electrode potential
• Electrolyte type Temperature
c)In-situ pH adjustment
• Ionization of CO2, organic acids, and ammonia for ion-exchange and migration under electric fields
• Formation of metal oxides and/or spurring coagulation/precipitation
• Assist with electrosorption/electrodeposition
c1) Bipolar membranes
c2) Faradaic reactions
• Water dissociation for pH adjustment
d)Ion-exchange membranes
d1) Membrane chemistry
• Prefers multi-valent over monovalent ions
• Molecular interactions
• Electrostatic
• Dispersion
• Hydrogen bonding
d2) Surface modified membranes
• Layer by layer assembly of oppositely charged polymer electrolytes or semi-conducting polymers or inorganic materials
• Promotes monovalent ion selectivity over divalent ions
d3) Composite membranes
• Particle or resin additives that promotes counterion selectivity
e)Other modes of action
• Shockwaves, electro-osmosis, and streaming potential in confined structures
• Electrocoagulation
3. Modes of action that enable electrochemical separations. (a) Electrode materials that exploit electrode properties (a1) and redox active polymers (a2) for electrosorption, (b) electrodeposition, (c) in-situ pH adjustment achieved by bipolar membranes (c1) and faradaic reactions (c2), (d) ion-exchange membranes with distinct bulk properties (d1), surface modification (d2), and composite membranes (d3), and other listed modes of action.
small enough and hydrated well.45 Examining the general form of ionic conductivity derived from the Nernst-Planck framework for an ideal electrolyte,46 or for a hydrated membrane like NafionTM , 47 it is important to note that conductivity scales with the square of the ion’s valence. Ion-exchange membranes’ surfaces can be modified with layer-by-layer assembly of oppositely charged polymer electrolytes48 and other materials45 to improve monovalent selectivity. However, this improvement in selectivity comes at the expense of a reduced ion flux. Nanofiltration membranes are an alternative membrane that favors monovalent ions over divalent ions and have been deployed in electrochemical separation platforms such as electrodialysis.45
An interesting strategy in recent years for selective ion separations of the same valences is the fabrication of composite membranes that feature selective particles to foster selective separations. Long and coworkers used porous aromatic frameworks embedded in sulfonated polysulfone membranes for the removal of toxic metal cations from water.49 Manganese dioxide (MnO2) particles incorporated into cation exchange membranes have resulted in greater uptake of phosphate ions from model waste mixtures to enable phosphate recovery (e.g., Fig. 3d3).50 It is easier to achieve selective organic acid anion separations with tailored anion exchange membranes because interactions between organic moieties can be exploited to increase the partitioning coefficient and/or diffusion coefficient. Jordan et al. have shown that tethered imidazolium cations in anion exchange membrane facilitate closer binding of lactate and p-coumarate organic acid anions. Furthermore, the imidazolium moiety also favors p-coumarate uptake via pi-pi interactions. The permeability of the ion across an ion-exchange membrane is the product of the partitioning coefficient and the ratio of ionic mobility terms (akin to the solubilitydiffusion model for gas separation membranes).51
It should be noted that most ion-exchange membranes sold commercially report only the permselectivity of the membrane for a counterion over the co-ion for sodium chloride solutions.14 This metric is important for current efficiency in electrodialysis and membrane capacitive deionization, but it is not very useful for targeted counterion separations from a mixture of counterions—which is important for critical mineral separations and nutrient recovery. The past 15 years has seen tremendous progress in the development of theoretical treatments using Manning’s Theory of Counterion Condensation to predict ion-exchange membranes’ partitioning behavior and diffusion coefficients for counterions and co-ions for single salts without adjustable parameters (note: predicting diffusion coefficients often uses a modified version of the Mackie-Meares model).52–57 This theory works for monovalent salts and multivalent salts54 and can be useful for understanding the permselectivity tradeoff for counterions over co-ions versus conductivity.58 Manning's Theory has been recently extended by Lin and co-workers for predicting counterion “i” over counterion “j” selectivity for ion-exchange membranes interfaced with aqueous salt mixtures.55 The extended theory is effective by relaxing the assumption that condensed counterions are immobile. Several reports have shown that condensed ions are mobile and that they diffuse faster than uncondensed counterions.19,55,56,59
pH adjustment of the liquid stream can play an important role in electrochemical separations for gases, organic acid ions, and critical mineral ions. As previously stated, in-situ pH adjustment can occur using a bipolar membrane, which dissociates water at the anion exchange and cation exchange interface that features a water dissociation catalyst under applied potential (> –0.83 V for the whole cell voltage) and via faradaic reactions such as ORR in base, OER in acid, HOR in acid, and HER in base. For CO2 separations, the exposure of the gas to hydroxide ions in the alkaline stream converts CO2 into bicarbonate or carbonate ions. These carbonate species exchange with an anion exchange membrane and migrate to (i) an electrode performing hydrogen oxidation to generate a proton source to regenerate the CO2 gas11,12 or (ii) a junction region that has protons generated from OER26 or a bipolar membrane.27 In the case of ionic separations, one notable example that showcases the importance of pH adjustment is for chromium capture.29 In the first step, HER was used to generate an alkaline environment and make the ferrocene redox
active polymer ferrocenium for the capture of hexavalent chromium oxide anions. After the capture, OER was performed at the counter electrode to generate a proton and to make the redox active polymer neutral for chromium release and the conversion of chromium (VI) to chromium (III)—the latter species being more benign compared to chromium (VI). This example conveys how Pourbaix diagram principles can be leveraged to enable a separations strategy. Further, it also shows how electrodes and in-situ pH adjustment work in tandem to attain selective separations. In bipolar membrane capacitive deionization, the bipolar membrane can generate an acid environment that favors copper ion reduction when capturing copper from model wastewater solutions.28 In-situ pH adjustment also allows for the conversion of captured and concentrated lithium salts to lithium hydroxide—the preferred precursor for making battery electrodes at lower temperatures.60 Furthermore, protonation and deprotonation by in-situ pH adjustment can be used for ionizing species such as ammonia to ammonium61,62 or organic acids to organic acid anions.28 Ionizing these species makes them amenable to migration under electric fields, and ion-exchange in membranes or resins. These steps facilitate the capture and concentration of the said species. pH adjustment can be useful for converting metal cations to metal oxides that precipitate out and that can be collected via filtration or skimming off the surface of the liquid if it floated to the top (this bears some similarities to electrocoagulation discussed in the next section). This approach was used in the recovery of nickel, manganese, and cobalt from expired lithium ion battery electrodes.63 As previously stated, insitu pH adjustment using bipolar electrodialysis or faradaic reactions is important for phosphate recovery via struvite precipitation.9,64 Struvite forms in the presence of phosphate and ammonia when the pH of the solution is greater than 9 and there are magnesium cations present. Overall, in-situ pH adjustment in electrochemical separations represents another tool in the toolbox for selective separations.
It might seem easier to pH-adjust the process streams by the addition of acids and bases before feeding them into the electrochemical separation unit. However, it is not always convenient to have additional acids and bases on-site for pH adjustment, and these chemicals can hail from energy-intensive processes such as chlor-alkali or from sulfur precursors that derive from fossilfuel mining. Lienhard and co-workers have shown that bipolar membranes for generating acid and bases can have the lowest energy use, in theory, but much work is still needed in lowering the actual energy use for generating acids and bases using bipolar membranes.65 The overpotentials for water dissociation and ohmic resistances in bipolar membrane electrochemical cells significantly compromise energy efficiency. Boettcher and co-workers,66,67 and others,68,69 have researched several classes of new water dissociation catalysts, such as earth-abundant metal oxides, to lower the water dissociation overpotential. Engineering the junction interface via electrospinning70 or lithographic patterning71 can also reduce the overpotential for water dissociation. Bipolar ion-exchange resin wafers also perform pH adjustment with lower ohmic losses as the resin wafer compartment augments the solution conductivity under dilute solutions.72 Conversely, it can be challenging to generate highly concentrated acid and base streams using bipolar membrane electrodialysis, but a recent ion-injection alteration to bipolar electrodialysis resulted in a NaOH stream over 8 M.73 Faradaic reactions are also effective for in-situ pH adjustment, but OER and HOR to generate acid requires platinum group metals, and the formation of gas bubbles within the cell can be undesirable. Faradaic reactions generating hydroxide ions can be achieved by using stainless steel, or other low-cost transition metals, for both OER and HER.64,74
Electrocoagulation is an electrochemical process that induces coagulation—the aggregation or precipitation of particles that can undergo collection because they flocculate or sink upon formation (due to the coagulant’s density). An electrocoagulation process often features a sacrificial anode, such as aluminum or iron, and a stainless-steel cathode for hydroxide ion formation via HER.75 The hydroxide ions form metal oxides or hydroxides in solution and further coagulation will occur with the metal cations from the (continued on next page)
sacrificial anode. Electrocoagulation has proved useful for the removal of copper, nickel, manganese, and zinc from wastewater,76 indium from microelectronics industry wastewater,77 strontium from a model solution,78 and phosphate from a model wastewater solution (the latter example was achieved by using a sacrificial magnesium anode).64 Electrocoagulation demonstrates high recovery of metal ions from a process stream, deploys cheap electrodes, and does not feature a membrane separator. These attributes make it a low-cost electrochemical separation platform. However, its general principle of operation makes it ineffective for selective metal separations from a mixture and the electrodes are prone to fouling.6,75 Altering the electrodes’ potential can mitigate electrode fouling.
Shock electrodialysis is a fairly new electrochemical separation platform for selective ionic separations.38 This method falls under the broad category of electrokinetic-driven separation that also encompasses electrodialysis and electrodeionization. Electrodeposition, electrosorption, and electrocoagulation represent distinct categories in electrochemical separations. A shock electrodialysis setup will feature a cathode for HER and an anode for OER. The two electrodes have cation exchange membranes in front of them with a porous conducting medium (e.g., borosilicate glass frit or ion-exchange resin wafer). Operating the cell over the limiting current spurs electroosmosis, ion surface conduction, and ion shockwaves in the porous conductor that have been exploited for desalination, and more recently, the removal of multi-valent ions from monovalent ions (e.g., heavy metal cations from sodium).38,79 Alkhadra and co-workers demonstrated shock ED for the removal of heavy metal, multivalent ions (cobalt, copper, mercury, zinc, cadmium, nickel, and manganese) from water streams with retention selectivity of the heavy metal ions over sodium that range from 2 to 25.80 The mechanism behind selective ion removal with shock ED is not fully known, but it has been asserted38,81 that the deionization shockwave is larger with multivalent ions and that these ions have a greater affinity for the charged surfaces of the porous medium where fluid transport is smaller due to the no-slip condition at the solid surface. The streaming potential could also be bigger for the multivalent ions and the streaming potential runs in the opposite direction of ion transport fostered by advection.
In this section, some key expressions governing electrochemical separation performance and design considerations are provided. The key expressions cover various relationships for selectivity, represented by separation factor, recovery of species, and the unit’s specific energy use.
A challenge in the field today is the various embodiments of expressions to describe the separation factor. Su and co-workers have recommended equations 1 to 3 to define the separation factor for one species over another.6 These equations take different forms for mixtures beyond two species. Equation 1 normalizes the quantity of species adsorbed to the initial quantity in the system. Equation 2 normalizes the amount adsorbed to the amount remaining in solution after equilibrium. For systems that have two process streams, such as a stripping solution and a feed solution, the separation factor is defined by equation 3.
NA,ads and NB,ads are the species adsorbed at equilibrium and NA,sol and NB,sol are the remaining species in the solution after equilibrium (eq) is achieved.
AB Astrip solB stripsol Afeed solB feed sol NN NN , ,, ,, / / (3)
NA,strip sol: Amount of species A in the strip solution
NB,strip sol: Amount of species B in the strip solution
NA,feed sol: Amount of species A in the feed solution
NB,feed sol: Amount of species B in the feed solution
For electrochemical gas separations, selectivity is typically not used. Rather, the composition of the purified gas is often conveyed. It is important to clarify whether the recovery value corresponds to a single pass or a recycled stream. Equations 4a and 4b give two descriptions for hydrogen recovery for an electrochemical hydrogen pump.82,83
hydrogenrecovery q q x H out Hin = 2 2 100 , % (4a)
qH2,out: hydrogen flow rate leaving the cathode
qH2,in: hydrogen flow rate entering the anode
hydrogenrecovery I Fq x tot Hin = 2 100 2 , % (4b)
Itot: Total current passing through the cell F: Faraday’s constant
*note: Equation (4b) assumes 100% current efficiency
The energy use of an electrochemical separation platform is governed by the product of the current and voltage values of the unit integrated over a time during the separation. The energy use is often normalized to the amount of purified product being produced (equation 5). This is defined as the specific energy consumption for separating species “i” (SECi). When reporting SECi, it is important to note the purity of the product reported and the specifics of the separation (e.g., feed concentration). Reducing the specific energy requires design engineers to lower the cell voltage. The tradeoff when reducing cell voltage is a lower current density value for the cell and a smaller flux rate for the species being separated. Operating the cell at a higher current density is desired as it results in a smaller cell for a given separation. However, pushing the current density too high can lead to unwanted side reactions (e.g., corrosion of electrodes or water electrolysis) and/or compromised selectivity. Both attributes reduce the current efficiency (CE; equation 6) of the cell and reduce the denominator (the amount of purified product collected) in equation 5. A key challenge for the field of electrochemical separations in the coming decades is to realize units that minimize all sources of resistances to promote current density while maintaining product selectivity. Minimizing the resistances will lower the cell voltage and operating a higher current density will shrink the size of the separation unit. The former reduces operating expenditure (OpEx) costs, while the latter reduces the capital expenditure (CapEx) costs.
SEC UI dt N i tot t i p () 0 (5)
NA,ads: Amount of species A (targeted species in this case) adsorbed
NB,ads: Amount of species B (competing species in this case) adsorbed
NA,0: Initial amount of species A
NB,0: Initial amount of species B
U: Cell voltage
tp: time period for the separation
Ni: The amount of species i separated
CE: Current efficiency
n: # of electrons transferred per species separated
In closing, it is an exciting time in electrochemical separations because the field is important to decarbonizing industrial processes and achieving the sustainability goals of the 21st century. At first glance, it can be difficult to connect the shared attributes amongst the diverse applications that deploy electrochemical separations. However, a deeper look at the electrochemical separation processes and their basic electrochemical principles makes it easier to identify the synergies. The most important attributes in electrochemical separations are electrode and electrolyte materials that enable selective removal of targeted species from a mixture while also promoting high flux rates (typically expressed by current density and current efficiency) and low energy use (which is commensurate with the operating voltage of the cell). These attributes govern the cost and the overall competitiveness of the electrochemical separation process versus conventional separation processes. Other notable advantages include electrochemical separations’ ability to be powered on renewable electrons while also generating less chemical waste (e.g., regeneration of electrodes or resins can occur by changing the polarity of the cell and using electrical energy rather than chemicals).
This work was supported by the US Department of Energy, Office of Science, Office of Basic Energy Sciences Separation Science program under Award No. DE-SC0022304.
©The Electrochemical Society. DOI: 10.1149/2.F08241IF
Christopher G. Arges, Research Engineer, Transportation and Power Systems Division, Argonne National Laboratory
Education: BS (University of Illinois at Urbana-Champaign), MS (North Carolina State University), and PhD (Illinois Institute of Technology) in Chemical Engineering; Postdoc (University of Chicago/Argonne National Laboratory) in Molecular Engineering
Research Interests: Ion-exchange membranes, Block copolymer self-assembly, Electrochemical separations, Fuel cells, Electrolysis Work Experience: Argonne National Laboratory from 2023–current, Associate Professor in Chemical Engineering at Pennsylvania State University from 2021 to 2023, Assistant Professor in Chemical Engineering at Louisiana State University from 2016 to 2021.
Pubs + Patents: 65 peer-reviewed publications, h-index 30, 60 invited talks, 5 ECS Interface articles, 6 papers in Journal of the Electrochemical Society.
Honors & Awards: NSF CAREER, ECS Toyota Young Investigator Fellowship, 3M Non-Tenured Faculty Award, Invited Speaker for 2023 EU-US Frontiers of Engineering Symposium, Journal of Materials Chemistry Emerging Young Investigator
Work with ECS: 14 years of ECS membership, Session Organizer for IE&EE Division, Member at Large for IE&EE Division and Energy Technology Division
1. National Academies of Sciences, Engineering, and Medicine. A Research Agenda for Transforming Separation Science; The National Academies Press, 2019.
2. D. S. Sholl, and R. P. Lively, Nature, 532(7600), 435 (2016).
3. F. P. García de Arquer, C.-T. Dinh, A. Ozden, et al., Science, 367(6478), 661 (2020).
4. Z. Xu, L. Wan, Y. Liao, et al., Nature Comm, 14(1), 1619 (2023).
5. B. K. Peters, K. X. Rodriguez, S. H. Reisberg, et al., Science, 363(6429), 838 (2019).
6. K. Kim, R. Candeago, G. Rim, et al., iScience, 24(5), 102374 (2021).
7. X. Su, Electrochem Soc Interface, 29(3), 55 (2020).
8. N. Kim, J. Jeon, R. Chen, and R. X. Su, Chem Eng Res Design, 178, 267 (2022)
9. F. Gao, L. Wang, J. Wang, H. Zhang, and S. Lin, Environ Sci: Water Res Technol, 6(2), 383 (2020).
10. A. Kogler, M. Farmer, J. A. Simon, et al. ACS ES&T Eng, 1(4), 662 (2021).
11. S. Matz, B. P. Setzler, C. M. Weiss, et al., J Electrochem Soc, 168, 014501 (2021).
12. L. Shi, Y. Zhao, S. Matz, et al., Nature Energy, 7, 238 (2022).
13. G. Venugopalan, D. Bhattacharya, E. Andrews, et al., ACS Energy Lett, 1322 (2022).
14. H. Strathmann, A. Grabowski, and G. Eigenberger, Ind Eng Chem Res, 52(31), 10364 (2013).
15. F. DiMascio, J. Wood, and J. M. Fenton, Electrochem Soc Interface, 7(3), 26 (1998).
16. S. Voskian and T. A. Hatton, Energy Environ Sci, 12(12), 3530 (2019).
17. J. C. Bui, É. Lucas, E. W. Lees, et al., Energy Environ Sci, 16(11), 5076 (2023).
18. C. Jackson, G. Smith, and A. R. Kucernak, Int J Hydrogen Energy, 52, 816 (2024).
19. M. L. Jordan, G. Kokoszka, H. K. Gallage Dona, et al., ACS Sustain Chem Eng, 11(3), 945 (2023).
20. US Department of Energy, Critical Materials Assessment; 2023.
21. C. Li, D. L. Ramasamy, M. Sillanpää, and E. Repo, Sep Purif Technol, 254, 117442 (2021).
22. S. Cotty, J. Jeon, J. Elbert, et al., Sci Adv, 8(42), eade3094 (2022).
23. J. Lim, C. A. Fernández, S. W. Lee, and M. C. Hatzell, ACS Energy Lett, 6(10), 3676 (2021).
24. D. Egan, The Devil’s Element: Phosphorus and a World Out of Balance, W. W. Norton & Company (2023).
25. T. A. Barckholtz, K. M. Taylor, S. Narayanan, S. Jolly, and H. Ghezel-Ayagh, Appl Energy, 313, 118553 (2022).
26. P. Zhu, Z.-Y. Wu, A. Elgazzar, et al., Nature, 618(7967), 959 (2023).
27. S. Datta, M. P. Henry, Y. J. Lin, et al., Ind Eng Chem Res, 52(43), 15177 (2013).
28. T. Kulkarni, A. M. I. Al Dhamen, D. Bhattacharya, C. G. Arges, ACS ES&T Eng, 3(12), 2171 (2023).
29. X. Su, A. Kushima, C. Halliday, et al., Nature Comm, 9(1), 4701 (2018).
30. D. A. Cullen, K. C. Neyerlin, R. K. Ahluwalia, et al., Nature Energy, 6(5), 462 (2021).
31. Z. Yan, J. L. Hitt, J. A. Turner, and T. E. Mallouk, Proc Natl Acad Sci USA, 117(23), 12558 (2020).
32. M. Peplow, Can industry decarbonize steelmaking? C&E News, American Chemical Society (2021).
33. D. Marciuš, A. Kovač, and M. Firak, Int J Hydrogen Energy, 47(57), 24179 (2022).
https://orcid.org/0000-0003-1703-8323
34. A. Chouhan, U. R. Aryal, P. O. Sivakumar, et al., Int J Hydrogen Energy, 46(76), 37965 (2021).
(continued on next page)
Arges (continued from previous page)
35. M. Sauer, D. Porro, D. Mattanovich, and P. Branduardi, Trends Biotechnol, 26(2), 100 (2008).
36. M. L. Jordan, T. Kulkarni, D. I. Senadheera, et al., J Electrochem Soc, 169(4), 043511 (2022).
37. J. G. Gamaethiralalage, K. Singh, S. Sahin, et al., Energy Environ Sci, 14(3), 1095 (2021).
38. M. A. Alkhadra, X. Su, M. E. Suss, et al., Chem Rev, 122(16), 13547 (2022).
39. C. Liu, Y. Li, D. Lin, et al., Joule, 4(7), 1459 (2020).
40. Y.-S. Yang, M.-L. Zhang, W. Han, et al., Electrochimica Acta, 118, 150 (2014).
41. A. Kurachi, M. Matsumiya, K. Tsunashima, and S. Kodama, J Appl Electrochem, 42(11), 961 (2012).
42. X. Su, K.-J. Tan, J. Elbert, et al., Energy Environ Sci, 10(5), 1272 (2017).
43. A. Román Santiago, S. Yin, J. Elbert, et al., J Am Chem Soc, 145(17), 9508 (2023).
44. R. Sujanani, M. R. Landsman,. S. Jiao, et al., ACS Macro Lett, 9(11), 1709 (2020).
45. C. Tang and M. L. Bruening, J Polym Sci, 58, 2831 (2020).
46. T. Sata, Ion Exchange Membranes, Royal Society of Chemistry (2004).
47. K. N. Grew, D. Chu, and W. K. S. Chiu, J Electrochem Soc, 157, B1024 (2010).
48. J. Ying, Y. Lin, Y. Zhang, et al., Chem Eng J, 446, 137076 (2022).
49. A. A. Uliana, N. T. Bui, J. Kamcev, et al., Science, 372(6539), 296 (2021).
50. A. Iddya, P. Zarzycki, R. Kingsbury, et al., Nat Nanotechnol, 17(11), 1222 (2022).
51. T. Luo, Y. Zhong, D. Xu, X. Wang, and M. Wessling, J Membr Sci, 629, 119263 (2021).
52. J. Kamcev, D. R. Paul, and B. D. Freeman, Macromolecules, 48(21), 8011 (2015).
53. D. Kitto and J. Kamcev, J Polym Sci, 60(21), 2929 (2022).
54. R. Sujanani, O. Nordness, A. Miranda, et al., J Phys Chem B, 127(8), 1842 (2023).
55. R. Wang, R. Duddu, and S. Lin, (2023).
56. Y. Huang, H. Fan, and N. Y. Yip, (2024).
57. Q. Lei, K. Li, D. Bhattacharya, et al., 15962 (2020).
58. C. Espinoza, D. Kitto, and J. Kamcev, 5(12), 10324 (2023).
60. Y. Chen, S. Song, X. Zhang, and Y. Liu, J Phys Conf Ser, 1347(1), 012012 (2019).
61. H. Dong, C. M. Laguna, M. J. Liu, J. Guo, and W. A. Tarpeh, Environ Sci Technol, 56(22), 16134 (2022).
62. Y. Li, R. Wang, S. Shi, et al., Environ Sci Technol, 55(21), 14886 (2021).
63. S. Yang, P. Zhang, F. Lai, et al., Electrochimica Acta, 431, 141144 (2022).
64. L. Kékedy-Nagy, M. Abolhassani, S. I. Perez Bakovic, et al., J Am Chem Soc, 142(44), 18844 (2020).
65. A. Kumar, F. Du, and J. H. Lienhard, ACS Energy Lett, 6(10), 3563 (2021).
66. S. Z. Oener, J. M. Foster, and S. W. Boettcher, Science, 369 (6507), 1099 (2020).
67. L. Chen, Q. Xu, S. Z. Oener, K. Fabrizio, and S. W. Boettcher, Nature Comm, 13(1), 3846 (2022).
68. Z. Yan, L. Zhu, Y. Li, et al., Energy Environ Sci, 18(8), 2235 (2018).
69. M. B. McDonald and M. S. Freund, ACS Appl Mater Interfaces, 6(16), 13790 (2014).
70. C. Shen, R. Wycisk, and P. N. Pintauro, Energy Environ Sci, 10(6), 1435 (2017).
71. S. Kole, G. Venugopalan, D. Bhattacharya, et al., J Mater Chem A, 9(4), 2223 (2021).
72. M. L. Jordan, L. Valentino, N. Nazyrynbekova, et al., Mol Syst Des Eng, 5(5), 922 (2020).
73. R. Fu, H. Wang, J. Yan, et al., AIChE J, e18345 (2023).
74. B. Chen, A. L. G. Biancolli, C. L. Radford, and S. Holdcroft, ACS Energy Lett, 8(6), 2661 (2023).
75. N. Yasri, J. Hu, M. G. Kibria, and E. P. L. Roberts, in Multidisciplinary Advances in Efficient Separation Processes, ACS Symposium Series, Vol. 1348, American Chemical Society, 167 (2020).
76. B. Al Aji, Y. Yavuz, and A. S. Koparal, Sep Purif Technol 2012, 86, 248 (2012).
77. W.-L. Chou, C.-T. Wang, and K.-Y. Huang, J Hazard Mater, 167(1), 467 (2009).
78. Z. V. P. Murthy and S. Parmar, Desalination, 282, 63 (2011).
79. H. Tian, M. A. Alkhadra, K. M. Conforti, and M. Z. Bazant,
Rare-earth elements (REEs) are “critical” materials for many existing and emerging technologies. Electrified transportation, wind power generation, magnetic data storage, and even defense equipment rely on neodymium (Nd), praseodymium (Pr), and dysprosium (Dy) as key components of permanent magnets that enable these technologies. Demand for REEs has drastically increased over the past few decades and is predicted to continue to grow even further. Currently, the processing and refining of REEs involve environmentally hazardous and energy–intensive steps that restrict their practice primarily to countries with less stringent environmental regulations. But heavy dependence on a single source for critical materials access poses serious supply chain risks. Developing innovative yet sustainable methods for refining critical metals is crucial for mitigating the environmental impacts caused by currently practiced REE processing methods.1
REE production involves many steps (i.e., mining, ore beneficiation, solvent extraction, and purification) as shown in Fig. 1. These steps yield rare-earth oxides, which are converted into metal via electrolysis. Here, we focus on neodymium (Nd) metal production, although the overall approach to metal production is similar for other rare-earths. The state-of-the-art process for Nd production uses a Nd2O3-containing NdF3-LiF molten salt electrolyte (at temperatures exceeding 1050 oC) and a consumable carbon anode.2 This oxy–fluoride process converts purified Nd2O3 and the carbon to Nd metal (at the cathode) and generates CO2 (at the anode). See Fig. 1. However, the low solubility of Nd2O3 implies transport limitations on oxygen species at the anode, resulting in an appreciable amount of perfluorocarbon (PFC) gas emissions in addition to CO2. These greenhouse gases (CO2, PFCs) make the conventional oxy–fluoride molten salt electrolysis (MSE) process undesirable. Additionally, the consumable carbon anode necessitates a “batch” process, requiring frequent heating and cooling cycles, rendering it very energy intensive (>8 kWh/kg, accounting for all energy inputs).3 Despite these disadvantages, the state-of-the-art molten salt electrolysis (MSE) process does present some key advantages: molten salts offer excellent ionic conductivities and high diffusivities, and hightemperature operation provides fast reaction kinetics—these are essential features for process scale-up, for lowering overpotentials and energy consumption, and for achieving high electrolysis rates.2,4
Moderate- or high-temperature chloride–based molten salts present an alternative medium to conventional oxy-fluoride melts because they also possess exceptional transport and kinetics properties, while allowing for more energy-efficient and sustainable metal refining.4 A comparison between the chloride-based MSE process and the stateof-the-art oxy-fluoride process is depicted in Fig 1. In the chloride MSE route, Nd2O3 is first converted into NdCl3 via noncarbothermic reaction with HCl or Cl2 gas. This process is uniquely possible for rare-earths because their chlorides are more thermodynamically stable than their oxides.2 The resulting chloride salt is then used as a feedstock for molten salt electrolysis. The medium for electrolysis can be a variety of chloride eutectic melts (e.g., LiCl-KCl in a 59:41 mole % ratio), which are readily available, benign, and provide a wide electrochemical potential operating window for electrolysis.4 When operated at moderate temperatures, electrolysis yields solid Nd metal. The Cl2 gas emitted at the anode can be sold—as it is a valuable coproduct—or it can be recycled into the upstream chlorination step. This process offers numerous advantages over the conventional route: (i) First and foremost, the chloride MSE route does not generate direct
greenhouse gas (CO2, PFCs) emissions, and thus is fully sustainable. (ii) Cl2 evolution offers the possibility of using a dimensionally stable, catalytic anode which not only lowers anode overpotentials and thus the cell voltage and energy consumption, but also provides better process control, including continuous processing.5-7 (iii) Reactor design and electrolyte optimization can enable higher current efficiencies (>80%), thus enhancing overall process efficiency above and beyond the conventional oxy-fluoride process. These aspects are discussed in further detail below.
Specific energy consumption (i.e., the amount of energy required to produce a given mass of Nd metal, kWh/kg-Nd), is a metric that considers thermodynamics and overvoltage penalties associated with the irreversibility factor during electrolysis, as well as inefficiencies from parasitic side reactions.2 For the chloride MSE process, the thermodynamic potential for electrolysis of NdCl3 (2.7 V) is higher than the oxy-fluoride process because NdCl3 is more stable than Nd2O3. However, the chloride MSE process has significantly lower electrolyte resistance, improved species transport, and potentially offers fast kinetics at both the anode and the cathode. These characteristics
(continued on next page)
account of the higher current efficiency and lower cell voltage when using a catalytic DSA anode.
al. (continued from previous page)
Direct CO2 emissions 0.2 kg-CO2 / kg-Nd none
*electrical energy consumption is 3.7 kWh/kg; however, the need for heating batch reactors elevates the net energy consumption for the oxy-fluoride process. Chloride MSE opens up avenues for continuous processing, which could eliminate heating costs.
allow the chloride process to operate at cell voltages that are at parity with or lower than the oxy-fluoride process. Considering the higher cathodic coulombic efficiency (discussed below) in chloride MSE, this process can enable much lower specific energies, thus creating a more sustainable process by lowering the energy consumed as well as by eliminating direct greenhouse gas production.2 A comparison of the relevant process parameters is provided in Table I.
There are a few key considerations in designing efficient rareearth electrowinning processes, especially as they relate to chloride MSE. A key aspect of process design in MSE of rare-earths, including Nd, is their multivalency (i.e., stability of multiple oxidation states in a halide medium). For Nd, Nd3+ and Nd2+ are both stable.4 Thus, during electrolysis, NdCl3 is first converted to Nd2+ (very fast step [1] shown in Fig. 2), followed by reduction of Nd2+ to Nd metal (relatively slow step [2] in Fig. 2). Nd2+ is susceptible to diffusion away from the cathode, which represents an efficiency loss. To quantify this loss, an efficiency loss factor L may be defined as the ratio of the rate of Nd2+ loss through diffusion to the rate of Nd2+ reduction to metal. As shown in Fig. 2 (right), L is proportional to the Nd2+ diffusion–limited mass transfer coefficient (D2+/δ) and its concentration (C2+),
and inversely propositional to the exchange current density (i0) and overpotential (η) for Nd deposition. Thus, to minimize Nd2+ loss, the electrolysis process must be operated at very large overpotentials (as large as possible without triggering parasitic reactions such as LiCl or KCl electrolysis)–this ensures fast reduction of Nd2+ to metal, thereby preventing Nd2+ loss to the electrolyte. Also, strategies to employ porous separators or diffusion barriers are effective in preserving the Nd2+ near the cathode and avoiding Nd2+ oxidation back to Nd3+ at the anode. Finally, temperature has a key role to play, since increasing temperature increases i0 and thus lowers the loss factor.
Electrowinning of solid Nd sponge at the cathode is advantageous because, unlike conventional oxy-fluoride processing, the product Nd stays adhered to the cathode surface and thus continually experiences an externally imposed cathodic potential. This avoids the possibility of a spontaneous comproportionation reaction between Nd and the electrolyte via: Nd + Nd3+ → 2Nd2+. This reaction is thermodynamically favored4 when an externally unpolarized Nd droplet contacts the electrolyte, as is the case in the oxy-fluoride process (Fig. 1). However, producing solid Nd via chloride MSE comes with its own set of challenges. Because metal deposition in moderateand high-temperature melts takes advantage of the intrinsically high exchange current densities in these media, spongy or porous deposits are typically obtained.2 As shown in Fig. 3, high purity (spot purity >92 at.%) deposits are easily obtained via chloride MSE; however, FIB-liftout followed by SEM/EDS reveals porosity in the deposited Nd metal. Electrolyte salts (e.g., LiCl and KCl) become entrapped in the Nd pores during electrolysis. This necessitates postprocessing such as vacuum distillation or arc remelting to purify the Nd so that it is suitable for application in NdFeB permanent–magnet fabrication. Recent developments in the use of electrolyte additives are noteworthy in this context.8 Additives can enable more compact and thus ultra-high-purity electrowon metal that circumvents the need for postpurification, thereby maintaining the energy requirements at a minimal value during metalmaking.
To circumvent efficiency loss due to multivalency, and to mitigate salt entrapment when making solid Nd metal, higher temperature (>860 oC) chloride MSE may be practiced. Several chloride mixtures (e.g., BaCl2–KCl above 650 oC) are stable liquids at very high temperatures. When electrolysis is performed in such melts containing NdCl3 (≈2.4 M) at 860 oC, solid Nd is produced, as evidenced in the CV in Fig. 4. Here, the negative scan direction yields Nd electrodeposition at cathodic potentials, but the reverse scan (going toward positive potentials from –2.0 V to –0.4 V vs. Mo quasi-reference electrode) exhibits Nd stripping because the solid Nd product is adhered to the tungsten (W) working electrode. Electrolysis at even higher temperatures (1050 oC, i.e., above the Nd melting point) also yields Nd plating as expected, but the stripping peak during the reverse scan is largely absent. This indicates that liquid Nd formed during the negative scan does not adhere to the cathode surface
because liquid Nd (density = 6.9 g/cm3) settles at the bottom of the electrolyte (density < – 3 g/cm3) and thus is detached from the working electrode. This experiment suggests the possibility of electrowinning liquid Nd metal, as has been explored recently by several other investigators.9 Liquid metal plating exhibits very high exchange current densities (≈1–10 A/cm2) compared to solid metal plating (0.1 A/cm2),10 thus liquid Nd plating can lower the efficiency loss factor L (Fig. 2) as long as comproportionation-related losses can also be minimized. Finally, liquid metal plating opens the possibility for a truly “continuous” process in which metal is continually electrowon and separated—this route is attractive for scale-up, process control, and overall process technoeconomics.
While the chloride-based MSE route is a promising method for enabling clean and sustainable rare-earth metal production across the globe, there remain several unresolved challenges. Chlorine handling, particularly at high temperatures, is a key safety concern. High-temperature chlorine corrosion is more aggressive, due not only to the increased rate of transport of chlorine through passivating surface oxide films, but also to the volatility of metallic chlorides.11 While enhanced chlorine corrosion poses a materials challenge for chlorine-handling systems, it also restricts the usability of traditional anode materials. Conventional titanium-coated mixed metal oxide (MMO) dimensionally stable anodes (DSAs) are common in lower temperature aqueous chlor-alkali processes; however, the sintered oxide coating can delaminate from the titanium substrate, presumably due to chlorine penetration leading up to corrosive attack of the underlying titanium during high-temperature chloride MSE. Graphite itself can act as a chlorine-evolving anode, but it typically exhibits larger overpotentials for chlorine evolution than MMO and can itself oxidize to CO2 in the presence of trace water or oxygen during chloride MSE. New DSA materials or stacks need to be developed to address these issues of long-term anode stability while also lowering the anodic overpotentials during chloride MSE.12
Despite the many advantages of the moderate-temperature chloride MSE process, it remains a “batch” process around 500 oC. The electrowon solid Nd needs to be collected by removing the cathode from the electrolyte melt at the end of a batch. While a cathode “swap” could be performed while the cell is held at temperature to minimize process interruptions, a truly “continuous” process is very desirable for scalability, cost, and energy-efficiency. Work is underway in our laboratory to develop a novel reactor and process design to continuously electrowin liquid Nd metal at 1050 oC in a chloride melt. Using an inert yet catalytic anode material, a low volatility chloride electrolyte (e.g., BaCl2–KCl or BaCl2–SrCl2), and a reactor design to periodically siphon the molten metal akin to industrial Al electrowinning, a continuous process is possible. A continuous process would greatly reduce the specific energy consumption by eliminating the heating-cooling cycles necessary for the conventional oxy-fluoride route.
Implementation of chloride MSE requires a NdCl3 feedstock. Producing a chloride feedstock first requires Nd2O3 production from ore via the established methods of ore processing (Fig. 1). In ore
processing, a mixed rare-earth chloride stream undergoes treatment using an organic ligand such as oxalate to selectively precipitate Nd3+. The oxalate is then calcined into Nd2O3, which releases CO2. Targeting this chloride intermediate stream rather than a purified oxide could provide opportunities for upstream integration of chloride MSE, which would further reduce the overall CO2 emissions associated with primary Nd production. An even greener alternative feedstock is the large volume of used magnets in end-of-life devices such as hard disk drives and wind turbines. Additional reduction in CO2 emissions can be achieved through recycling of the Nd in used magnets. It has been shown that oxide feedstocks derived from used magnets could provide a >50% reduction in greenhouse gas emissions compared to those produced from ore bodies.13 Given that recycling also often involves an oxalate treatment followed by a calcination step, targeting the separation of Nd from NdFeB magnets into its chloride form (NdCl3) for direct input into chloride MSE offers an opportunity to achieve nearly complete circularity in rareearth processing.
Note here that Nd separation from NdFeB may also be achieved electrolytically in an electrorefining cell (Fig. 5) with chloride molten salt as the electrolyte. Here, Nd from NdFeB is selectively oxidized to Nd3+ (at the anode), which then undergoes reduction to pure Nd metal (at the cathode)—this has the potential to accomplish separation and purification in a single unit operation. Cyclic voltammetry of NdCl3and FeCl2-containing melts confirms that selective separation of Nd can be achieved over a rather wide potential range (Fig. 5). However, a key challenge in realizing such separation is the slow solid-phase diffusivity of Nd out of the NdFeB anode. Utilizing micro-sized NdFeB particles with short diffusion lengths and high surface areas will be key to achieving industrially relevant rates of Nd separation and electrorefining. Specialized pulsing waveforms can be employed as well to maximize transport of Nd out of the solid particle and into Nd3+ species while preserving selectivity.14
Critical materials, such as rare-earth metals, are essential to numerous applications, including clean energy; however, the present industrial practices for producing rare-earth metals involve environmentally damaging and thus unsustainable chemical and electrochemical processes. For example, neodymium metal
(continued on next page)
oC, liquid Nd is electrowon which separates (due to higher density than the electrolyte) from the W electrode, and thus is not re–oxidized during the reverse scan. This hints at the possibility of making liquid Nd via chloride MSE.
production using oxy-fluoride molten salt electrolysis is not only energy-inefficient but also generates potent greenhouse gases (CO2, PFCs). An alternative moderate-temperature chloride-based molten salt electrolysis process can address these issues, providing energyefficient and sustainable neodymium metal production. But more work is needed in advancing this process: developing catalytic yet stable chlorine-evolving anodes; “continuous” metal recovery while enhancing its purity; and upstream or downstream (recycling) integration into the supply chain for minimizing greenhouse gas emissions. The chloride MSE can be viewed as a platform technology. While it is being developed presently for rare-earth electrowinning, one can easily envision its broader application to rare-earth electrorefining and the electrolytic production of high-volume metals like Fe and Al. Presently, these high-volume metals industries account for nearly 10% of global greenhouse gas emissions. Thus, the chloride MSE process presents a huge opportunity for truly achieving sustainability if it is developed further for producing Fe, Al, Ti, Mg, and other commodity metals.
The authors acknowledge support from the US Department of Energy’s Office of Energy Efficiency and Renewable Energy under the Advanced Materials and Manufacturing Technologies Office, Award Number DE-EE0009434. This work was also supported by the Critical Materials Innovation Hub, an Energy Innovation Hub funded by the US Department of Energy, Office of Energy Efficiency and Renewable Energy, Advanced Manufacturing and Materials Technologies Office.
©The Electrochemical Society. DOI: 10.1149/2.F09241IF
Ben Holcombe, PhD Candidate, Chemical Engineering, Case Western Reserve University (CWRU) & Critical Materials Institute (CMI)
Education: BE in Chemical Engineering (CWRU)
Research Interests: Industrial electrochemistry and electrochemical engineering; Sustainable manufacturing; Electrochemical reaction engineering; Understanding and modeling of the interplay between charge and mass conservation in non-aqueous, multivalent metal electrodeposition; Developing novel electrodes and cell designs for high temperature molten salt electrolysis systems.
Honors & Awards: CWRU Monroe J. Bahnsen Award for excellence in design and undergraduate research, ACS Associate’s Award for Excellence in Organic Chemistry (Trenton Region), Betty Wallace Cornelius Award for Excellence in Mathematics.
https://orcid.org/0009-0003-4501-8431
Nick Sinclair, Staff Scientist & Adjunct Instructor, CWRU
Education: PhD in Chemical Engineering (CWRU)
Research Interests: Advanced energy storage systems based on redox organics in deep eutectic solvent electrolytes
Work Experience: As a staff engineer, researched electrochemical technologies from bench-top proof-of-concept to scaled-up systems leading to successful tech-transfer to an industrial licensee of a DOE ARPA-E sponsored energy storage concept utilizing Fe electrochemistry. Experienced with electrolysis cell design and scale-up, as well as manufacturing for a variety of industrial applications. Most recently, he has been involved in the development of a molten salt electrolysis process for the sustainable production of rare-earth metals.
https://orcid.org/0000-0002-8268-966X
Alex Baker, Staff Scientist, Actinide and Lanthanide Science Group, Lawrence Livermore National Laboratory (LLNL)
Education: MPhys in Physics (University of Durham), PhD in Condensed Matter Physics (University of Oxford and Diamond Light Source)
Research Interests: Understanding the structural, chemical, mechanical, magnetic, and electronic properties of metals.
Work Experience: Worked with the Ames National Laboratory Critical Materials Institute (CMI) for 6 years, including as an LLNL lead on efforts related to maturation of new permanent magnet formulations and on Nd electrowinning.
Pubs + Patents: >60 journal publications
Honors & Awards: R&D 100 award for his work on the Al-Ce alloy which seeks to improve the economics of rare-earth mining by developing a high-volume coproduct demand.
https://orcid.org/0000-0003-0700-0858
Eunjeong Kim, Postdoctoral Researcher, Materials Science Division, Actinide and Lanthanide Science Group, LLNL
Education: PhD in Materials Science Engineering (Hanyang University).
Research Interests: Characterization of hotdeforming NdFeB, CeFeN magnets and DSA anodes for Nd electrowinning, Development of heavy-metal high-entropy alloy hohlraum materials through a sputtering deposition process.
Work Experience: Prior to joining LLNL in 2022, her doctoral research centered around investigating magnetocaloric refrigerant materials for active magnetic regeneration systems.
https://orcid.org/0009-0004-1295-1484
Scott McCall, Physicist, LLNL & Lead, Crosscutting Research, Critical Materials Institute (CMI)
Education: PhD in Condensed Matter Physics (Florida State University)
Research Interests: Understanding the structural, chemical, mechanical, magnetic, and electronic properties of lanthanides and actinides.
Work Experience: Extensive background working on critical materials topics important for the energy, economic, and national security of the United States. A founding member of CMI, he currently leads its Crosscutting Research Focus Area. He is also the Actinide & Lanthanide Science Group Leader in the Materials Science Division LLNL
Pubs + Patents: >100 papers, of which ~50 are focused on rareearths; >12 patents on rare-earth materials.
1. B. J. Smith, M. E. Riddle, M. R. Earlam, C. Iloeje, and D. Diamond, Rare Earth Permanent Magnets: Supply Chain Deep Dive Assessment. (United States, 2022).
2. R. Akolkar, J Electrochem Soc, 169, 043501 (2022).
3. A. Schreiber, J. Marx, P. Zapp, and W. Kuckshinrichs, Adv Eng Mat, 22, 1901206 (2020).
4. D. Shen and R. Akolkar, J Electrochem Soc, 164, H5292-H5298 (2017).
5. R. Akolkar, System and process for sustainable electrowinning of metal. US Patent application 2023/0279572 A1 (2023).
6. M. F. Chambers and J. E. Murphy, Molten-Salt Electrolysis of Neodymium from a Chloride Electrolyte. Report of Investigations 9391, US Bureau of Mines (1991).
7. N. S. Sinclair, D. R. N. Wasalathanthri, B. Mainali, B. Holcombe, A. Orhan, and R. Akolkar, ECS Meeting Abstracts, MA2022-02, 2500 (2022).
https://orcid.org/0000-0002-7979-4944
Rohan Akolkar, Milton and Tamar Maltz Professor of Energy Innovation, CWRU
Research Interests: Span many areas of electrochemical engineering, including batteries, semiconductors, and electrometallurgy.
Work Experience: An Ohio Eminent Scholar, Faculty Director of CWRU’s Great Lakes Energy Institute, Director of the CWRU Electronic Design Center (EDC), and Chief Scientist at the Pacific Northwest National Laboratory. Prior to joining the CWRU faculty in 2012, he conducted R&D at Components Research Division of Intel Corporation.
Honors & Awards: 2023 Electrodeposition Division Research Award of ECS, Norman Hackerman Young Author Award of ECS, and recent election as Senior Member of the National Academy of Inventors (NAI).
Work with ECS: Associate Editor of the Electrochemical Society
8. S. A. Kuznetsov, A. V. Popova, Y. V. Stulov, and S. I. Markovich, J Electrochem Soc, 170 076508 (2023).
9. D. K. Sahoo, PhD Thesis, “Study on Electrodeposition of Light Rare Earth Metals and Alloys from Molten Chloride Electrolytes,” Homi Bhabha National Institute (2015).
10. J. Thonstad and S. Rolseth, Electrochmica Acta, 23, 223 (1978)
11. W. Zhai, B. Yang, S. Zhang, J. Ni, S. Li, and Y. Li, IOP Conference Series: Earth and Environmental Science, 450, 012006 (2020).
12. R. Akolkar, B. Holcombe, N. S. Sinclair, and D. R. N. Wasalathanthri, Dimensionally Stable Anode for Electrolytic Chlorine Evolution in Molten Salts. US patent application filed (2023).
13. H. Jin, P. Afiuny, T. McIntyre, Y. Yih, and J. W. Sutherland, Procedia CIRP, 48, 45 (2016).
14. H.-S. Lim et al., Report 34948, Pacific Northwest National Laboratory, Richland WA (Sept 2023).
https://orcid.org/0000-0002-9865-5704
Separation and purification of molecules and compounds is one of the fundamental processes of chemistry and a hugely important factor in industrialization, as shown in Fig 1, accounting for as much as 15% of world’s energy consumption.1As the world tries to limit and reduce the effects of climate change, simultaneous development of a green chemical economy and carbon sequestration strategies are needed. Both these goals need to effectively and efficiently separate gas phase molecules to make the implementation of these technologies feasible, requiring an even higher demand for economical and green gas phase separation. The current methods of gas phase separation used in industry rely predominantly on inefficient processes, such as cryogenic distillation or temperature or pressure swing absorption.2 Gas phase separation by electrochemical means can offer theoretically high energy efficiency separation, as the energy supplied can operate exclusively on the molecule of interest, if proper material and design criteria are met, while traditional methods act upon the entire gas mixture. Additionally, electrochemical separations can reduce the number of steps needed as they can simultaneously separate and compress the purified gas, easing the demand for high energy processing required when using mechanical pumps. However, the effectiveness of these technologies depends largely on the operating conditions and materials that make up the electrochemical cell. Thus, a key factor driving improvement in this field relies heavily on material development, such as improved membranes/separators, catalysts, and other cell materials which can in turn give rise to novel techniques and high performance of the electrochemically driven devices. Electrochemical gas phase separations integrated with green energy are critical to reaching climate change targets, but they in turn rely on the development of novel materials at enlarged scales and implementation of these technologies at reduced costs. In this article, we discuss the principles and applications of three electrochemical gas separations: hydrogen (H2) pump, ammonia (NH3) compression, and carbon dioxide (CO2) separation.
well as spontaneous hydrogen compression, makes an EHP highly attractive for this application.
A typical EHP fitted with a proton exchange membrane (PEM) works as described in Fig 2a where hydrogen is selectively oxidized via the hydrogen oxidation reaction (HOR) in the gas mixture at the anode, transported as H+ across the PEM and converted back to
(continued on next page)
Hydrogen, particularly clean hydrogen, is considered one of the primary molecules for decreasing reliance on conventional fossil fuels. It can be used either in its molecular form or attached to C or N, in the form of methanol and ammonia respectively, as a part of a power-tomolecule technology.3 While water electrolysis represents the most promising route toward the green hydrogen of the future, currently, ~95% of the 50 million tons of H2 produced annually is sourced from fossil fuels like methane.4 This industrial process is accomplished via gasification or reformation of methane into syn gas, typically hydrogen, carbon dioxide, and carbon monoxide. The resulting syn gas has many impurities, and the hydrogen must be separated prior to most applications, especially in fuel cell devices, which can be highly sensitive to any impurities. This separation can be done through pressure swing adsorption (comprising 85% of hydrogen purification5), cryogenic distillation, or membrane technologies (e.g., palladium membranes). While the first two technologies are only feasible for large-scale and centralized applications, membranes allow for a much more customizable approach. Using membranes to separate the hydrogen can be done through a physical process involving differential pressure, temperature, or concentration, or via an electrochemically driven process known as hydrogen pumps (EHPs). Its continuous operation, high efficiency, ultra-high electrochemica purity, and modulable device design for scale up, as
Stracensky and Xu
(continued from previous page)
hydrogen at the cathode by the hydrogen evolution reaction (HER), forming hydrogen gas with a much higher purity and/or pressure on the cathode side of the membrane. The latter is also called electrochemical hydrogen compression (EHC).6 The level of selectivity and efficiency depends largely on the catalyst, the membrane, and the operating conditions of the cell. The catalyst must be highly active for the HOR and/or HER reaction, resistant to poisoning from the other gases in the mixture, and economically viable for the application. Similarly, the membrane must conduct protons effectively and selectively and be durable in extended operation conditions and mechanically strong to withstand pressure differentials. The operating conditions largely affect selection of materials in a particular EHP technology. One of the most important operational parameters is the temperature of the EHP. Co-designing materials and operating conditions is critical to mitigate the poisoning effect from the impurities traditionally found in syn gas. Carbon monoxide (CO) poisoning of the HOR catalyst is a significant concern as CO strongly binds on the catalytic sites at lower temperature, thus blocking the reaction sites.7 One approach to decreasing poisoning effects is to develop catalyst materials with higher CO tolerance than traditional HOR catalysts. The development of PtRu catalysts has demonstrated increased CO tolerance over Pt and PtNi, but are still limited to low concentrations of impurities, with significant poisoning effects observed with just 20 ppm CO when operated at lower temperatures.7,8 An alternative approach to decreasing the poisoning effects is operating EHPs at higher temperatures, which facilitates the removal of CO from the catalyst sites by decreasing the binding energy of CO to the catalytic site and increasing the rate of desorption, thus improving the efficiency of the cell.9 However, development of materials that are durable for long-term operation at elevated temperatures of 160–220 °C remains paramount to reducing these poisoning effects. Typical PEM materials such as the sulfonic acid-containing NafionTM cannot run for long above 100 °C, as water is needed to facilitate the transport of the ions via the Grotthuss mechanism. As these membranes become dehydrated, the dominant method of proton transfer shifts to the vehicle mechanism which causes a significant decrease in proton conductivity.10 Thus, work has investigated the development of phosphoric acid (PA) based-PBI materials that can operate at elevated temperatures that tolerate CO ~160 to 220 °C; however, leaching of the phosphoric acid occurs and is exacerbated at temperatures above 180 °C.11,12 When water activity in the PA-PBI system is high, exponential decay of PA loss occurs via the water replacement mechanism. Los Alamos National Laboratory (LANL) and others addressed this issue by developing protonated pair-coordinated proton exchange membranes, in which quaternary ammoniumfunctionalized membranes are doped with phosphoric acid.11,12 Due to the high basicity of the quaternary ammonium hydroxide,
the acidic proton of phosphoric acid is completely deprotonated to form a strong ionic interaction between quaternary ammonium and biphosphate. Alternatively, a phosphoric acid blended with sulfuric acid ionomers shows improved kinetics and ionic conductivity while suppressing phosphate ester formation.13 Figure 2b shows PEM EHC performance using this membrane technology. This high-temperature PEM technology is being commercialized by Advent Technologies.14
Further elevating the temperature to reduce the effect of the poisoning of catalysts is achievable by removing the polymeric membrane completely in favor of a proton-conducting ceramic which can operate at temperatures up to 1000 °C, but which typically operates at much lower temperatures to reduce the materials’ thermal and mechanical property requirements. This high-temperature operating condition offers greater resistance to poisoning as well as the intrinsic advantages of high-temperature electrochemistry, which lowers the activation barrier of reaction and thus allows for lower overpotentials and for the use of cheaper catalysts.15 These solid oxide electrochemical hydrogen pumps (SOEHPs) typically utilize proton-conducting metal oxide electrolytes comprising barium or strontium combined with zirconium (Zr), cerium (Ce), terbium (Tb), or thorium (Th) doped with yttrium (Y) to stabilize the conductive phase.16 Because of the high operating temperatures offered by the solid oxide membrane, more options for electrodes are possible— from cheaper transition metals such as Ni and Cu to more expensive materials such as Pt and Pd.15,17 However, material design is still a crucial barrier to overcome with this technology due to the typical high operation temperatures of ≥500 °C. The materials used in the cell experience are thus uniquely challenged by coking, sealing, thermal expansion, and chemical compatibility. Each component of the cell must be resistant to these heat-induced effects, while still maintaining the desirable properties such as activity and ion transport that provide the electrochemical cell performance. A particularly difficult degradation pathway is thermal expansion mismatch. Issues arise when the different materials have dissimilar thermal expansion coefficients, which leads to fractures and loss of sealing during the temperature ramp-up from room-temperature to the high operating temperature.18 This degradation is further exacerbated with thermal cycling.19 Thus, there has been significant effort to reduce the operating temperatures below 500 °C while still maintaining the benefits of poison resistance and increased performance.17 Further development of this promising route for future hydrogen pump technologies requires a multidisciplinary approach of engineering, chemistry, and material science before widespread application of this technology can occur.
The other advantage of EHP is its ability to compress hydrogen without using a mechanical pump. When purified hydrogen is generated on the cathode, the hydrogen pressure can be consistently built up. This process, which can compress hydrogen above 10,000 psi, is also called electrochemical hydrogen compression (EHC).6
Skyre, Inc. and Giner, Inc. are two companies that specialize in EHC. Giner’s recent EHC stack was successfully pressure tested at 12,687 psi (875 bar) using a special membrane and electrode design as shown in Fig 2b.20 Commercial H2RENEWTM manufactured by Skyre, Inc., can effectively separate and compress hydrogen for industrial-scale H2 recycling, H2 energy storage, and fueling applications. To reach the desirable pressure, the proton exchange membranes must be reinforced and mechanically strong and special sealing materials must be designed to sustain this high non-balanced hydrogen pressure. In addition to the pressure, energy consumption is another important consideration. State-of-the-art EHC technologies can achieve energy consumption as low as 2 kWh/kg H2 at 500 mA/cm2 and 5,000 psi.20
Hydrogen generated from renewable energy often needs to be transported across long distances before it can reach its end use. Storage and transportation of hydrogen can be very difficult; thus converting the hydrogen gas into a hydrogen carrier molecule or a higher value product onsite, such as methanol (CH3OH) or ammonia (NH3), has become an attractive idea. There is a strong need for ammonia, as ~80% of global ammonia production (~180 metric tonnes/yr) is used in fertilizer production to feed the world’s growing population.21 However, the synthesis of ammonia required to meet this demand consumes ~1% of the world’s energy production22 and produces ~1.8% of global CO2 emissions.21 Currently the most common method for ammonia synthesis is via the Nobel-winning Haber-Bosch process which accounts for nearly all ammonia production. The ammonia produced by this method and others needs to be of high purity and pressurized for economical transport and storage. Traditional mechanical pumping methods are often used but they lack high effectiveness with only a 60–75% isentropic efficiency.23,24
An electrochemical ammonia compression process can be highly efficient, as it is noiseless and vibration free. However, for the electrochemical compression of ammonia, the decomposition of ammonia must be avoided when operating at higher voltages, as the ammonia can be electrochemically oxidized. A PEM electrochemical compressor has been developed in which the ammonia reacts with the carrier hydrogen on the low-pressure anode to form a positively charged ammonium species that exchanges and migrates across the ammonium-soaked PEM and then regenerates as ammonia and hydrogen at higher pressure on the cathode, as demonstrated in Fig 3.25 This cell showed high compression efficiency, >93%, and stable operating conditions for several hours.25
Ammonia is typically considered a poison for the PEM when operated as a fuel cell or hydrogen pump. The process of exchanging
the PEM with ammonium cations allows for the transport of ammonia while restricting the transport of protons.3,25 Further work on this system looked at the backflow of ammonia and hydrogen through the NafionTM membrane and found that when the cathode compartment was pressurized, back diffusion of ammonia occurred at a much higher rate than hydrogen.26 This effect was also observed when running a stack, where the back diffusion at higher pressures reduced the isentropic efficiency. However, increasing the cell current leads to a higher ammonia ratio in the exhaust gas because larger current values promote the forward flow of ammonia across the membrane while the back diffusion rate is constant.27 Although electrochemical pumps for purifying and compressing hydrogen is a promising technology, further membrane and system design is needed to limit the back diffusion of ammonia when operated at high enough pressures to rival the efficiency of mechanical pumps. Additionally, while this pump can remove reactant nitrogen from the gas stream, it requires H2 as a transport gas which is oxidized for the protonation of ammonia and results in simultaneous transport of H2 along with ammonia across the membrane. However, NH3 can be easily condensed under mild pressure (20°C and 7.5 bar), while hydrogen will remain as a gas even at a high pressure.
After H2/NH3 separation, the cathode hydrogen can be recirculated to the anode for the further protonation of ammonia, allowing for a complete loop. This recirculation eliminates the need for an EHP to pump out the hydrogen, which is necessary because NH3 can cause damage to phosphoric acid-based hydrogen pumps and lowtemperature fuel cells.3,28 The major degradation in the presence of ammonia comes from the formation of ammonium which can exchange with the cation groups of the PEM, lowering the conductivity and hindering transport of hydrogen, making the electrochemical separation of hydrogen and ammonia difficult via PEM-ECPs. While currently energy intensive, ammonia can be synthesized via electrochemical reduction of nitrogen with hydrogen29–31 which, when paired with water electrolysis to produce hydrogen, can provide a completely electrified ammonia production system that pairs well with renewable energy sources and dramatically reduces the amount of CO2 produced from ammonia synthesis. Alternatively, ammonia can be synthesized from nitrates in agriculture run-off, or mining waste streams, simultaneously removing harmful concentrations of nitrates from the environment and producing a chemical commodity while avoiding the challenges of direct N2 reduction.32,33
With an increasing global population and rapid industrialization, the mitigation of greenhouse gases is required to limit the impact of climate change to less than 2 °C above pre-industrial levels. Carbon dioxide (CO2) is a well-known greenhouse gas that is pervasive in the industrialized world. To meet these climate goals, CO2 capture needs to be implemented. CO2 can be removed from a point source, often an industrial process such as flue gas emissions or methane reforming gas, where the CO2 concentration can be as high as 15%, or by direct air capture (DAC) where CO2 is in a diluted state of ~400 ppm.34 The concentrated captured CO2 not only reduces the amount of CO2 in the air but can also be used as a product (e.g., for enhanced oil recovery or for use as a supercritical fluid for separations) or converted to higher value products via electrochemical CO2 reduction reaction. Traditional methods of separating CO2 from the flue gas of a powerplant rely on thermal or pressure swings to modulate the affinity of a sorbent for CO2 during capture and release of the molecule.34,35 Although these processes can be effective with the amine adsorbents that are considered state-of-the-art in large-scale plant demonstrations,36–38 significant increases in efficiency and costs are required if these methods are to become economically viable. Electrochemical processes for CO2 capture are also gaining more attention due to their ability to operate isothermally and without depending on temperature control. As with the previous systems, the electrochemical design allows for modularity, easy coupling with renewable energy systems, and access to different operational configurations such as continual flow. Currently there are many
(continued on next page)
Stracensky and Xu (continued from previous page)
methods of CO2 capture that are being explored that deploy different principles and configurations, as demonstrated in Fig. 4.
One method for continual operation DAC at concentrations of 400–5,000 ppm of CO2 is the use of a shorted electrochemically driven CO2 separator (EDCS) cell.39,42 In this method the anion exchange membrane (AEM), which is typically poisoned by CO2, is converted to the carbonate form and electrically shorted to allow for the transport of CO2 from the cathode to the anode, as shown in Fig. 4a. This electrical short prevents voltage control of the cell but is instead operated by the flow of hydrogen across the anode. In this configuration the hydroxide is generated on the cathode via the electrochemical reaction of oxygen with water. The hydroxide anions react with CO2 in the air to form carbonate or bicarbonate that can transfer across the AEM and oxidize back to CO2 at the anode—releasing back the hydroxide which reacts with the hydrogen to reform water and release the energy to power the cell. This device is capable of removing > 98% of CO2 from air in a single pass when in a spiral-wound design with a high air flow rate of 10,000 sccm. Powered by hydrogen, this device can be easily integrated with green hydrogen generation. The flow-through design achieves high efficiency and costs approaching $100/kW stack.
A second method of CO2 capture is by electro-swing adsorption which works well for point sources, such as flue gas, where higher concentrations of CO2, in the range of 0.6–10%, are present.40 This technology works like a battery, where during the charging phase the CO2 is passed over the poly-1,4-anthraquinone-decorated
substrate, covalently binding with electrons provided by the oxidation of poly(vinyl ferrocene). During the discharge phase, the CO2 is electrochemically removed, the substrate is regenerated, and the electrons transfer back to reduce poly(vinyl ferrocenium) to poly(vinyl ferrocene), as shown in Fig. 4b. The technology was originally invented by researchers at MIT who demonstrated the capture of CO2 both in a sealed chamber and in an adsorption bed from inlet streams of CO2 concentrations as low as 0.6% and up to 10%, at a constant CO2 capacity with a faradaic efficiency of >90% and an energy consumption of 40–90 kJ/mole of CO2 captured.34 This technology has shown good stability over 7,000 cycles with high faradaic efficiency, >90 %, attracting interest for industrial implementation. The technology has been licensed by Verdox, Inc. In addition, the Italian startup Energy Dome, maker of the world’s first CO2 battery, is officially entering the US market. Energy Dome’s battery uses carbon dioxide to store energy from wind and solar on the grid.
High-temperature systems using ceramic molten carbonate membranes have also been exploited for electrochemically driven CO2 separations. This technology is particularly applicable for removal of CO2 emissions from high-temperature industrial flue gases where traditional polymer membranes would degrade.43 These systems are dual-phased and consist of molten carbonate salts, which are impregnated in a meticulously designed porous metal oxide substrate (e.g., alumina or zirconia). The principle of operation is molten carbonate fuel cells (MCFCs). In the MCFC shown in Fig 4c, CO2 reacts with O2 to form carbonate ions at the cathode; carbonate ions move across the molten carbonate electrolyte to reach
the anode where they react with hydrogen to produce water and CO2 again. The net reaction is 2H2 +O2 → 2H2O while CO2 in the flue gas or reformate gas from methane is pumped from the cathode to the anode with a much higher purity emanating from the anode. In addition to their high working temperature, which allows for energy-efficient operation, the mixed-matrix membranes allow for higher selectivity and permeability over traditional materials such as polymer membranes and MOFs.44 This high operating temperature affects the viscosity of the electrolyte and electrolyte evaporation rate when heated to >650 °C.43 Fuel Cell Energy, Inc. has developed this technology for commercial application by implementing it for power plant flue gas to remove ~ 90% of CO2 from the exhaust while generating additional electricity from natural gas or another fuel source, as seen in Fig 4c.45 This system integrates well with the existing power-generation infrastructure, which can help ease the transition to renewable energy sources. Despite its remarkable advantages, CO2 capture via a MCFC has been challenged by the limited life of MCFCs. The dominant factors that lead to the poor lifespan of MCFCs include hot corrosion on key components due to molten carbonate, increased cell resistance due to evaporated electrolyte, and Ni shorting due to the deposition of Ni catalysts in the matrix electrolyte. These problems must be addressed before MCFC technologies for CO2 capture can be viably applied.
Carbon capture is a meaningful technology to reduce the impact on climate change. Carbon capture enabled by electrochemical separations has been shown to operate at high efficiency while generating highly concentrated CO2 effluents. Furthermore, some embodiments lead to the cogeneration of other valuable products like electricity and clean hydrogen. However, in this still developing field, new materials and operation strategies must be continually discovered. It will require diverse technologies to provide the best solution to a variety of challenges. Thus, it is recommended that multiple CO2 technologies be developed, in order to respond to unique application conditions.
There is a huge energy demand for separations, with the energy typically sourced from fossil fuels. Electrochemical gas separations offer high theoretical efficiency and purity, and ease of integration with renewable energy sources. The main challenge facing many of these technologies is the materials, specifically separators/membranes and catalyst materials with high performance and long lifetimes, to achieve practical high efficiencies and low costs. In addition, there are likely many unknown challenges that may arise during scaleup and integration when used in the field; thus it is vital to focus on advanced materials development, cell and stack validation, and system integration with intermittent renewable energy in the field to better understand the challenges before electrochemical gas separations can be widely applied.
©The Electrochemical Society. DOI: 10.1149/2.F10241IF
Hui Xu, Envision Energy USA
Education: BS in Chemical Engineering (Wuhan Institute of Technology), PhD in Chemical Engineering (University of Connecticut).
Research Interests: Electrolysis and fuel cells.
Pubs + Patents: >50 papers, h-index: 39, 5 patents.
Awards: Key Contributor to 2021 Special Recognition Award from DOE H2 Program Annual Merit Review Meeting; Inducted into the University of Connecticut Academy of Distinguished Engineers (2020). Cofounder of Gordon Research Conference-Water Electrolysis to be launched in 2025.
Work with ECS: Energy Technologies Division Secretary; Guest editor for three JES special focus issues.
https://orcid.org/0000-0001-6829-7187
Thomas Stracensky, Envision Energy USA
Education: BS in Chemistry (Salve Regina University), PhD in Chemistry (Northeastern University).
Research Interests: Electrolyzers, Fuel cells, Redox flow batteries.
Work Experience: Graduate student internships at Giner and LANL, Electrochemist at Envision Energy since 2023.
Pubs + Patents: 7 papers.
https://orcid.org/0000-0002-2408-0063
1. D. S. Sholl and R. P. Lively, Nature, 532, 435 (2016).
2. M. Rhandi, M. Trégaro, F. Druart, J. Deseure, and M. Chatenet, Chinese J Catalysis, 41, 756 (2020).
3. L. Vermaak, H. W. J. P. Neomagus, and D. G. Bessarabov, Membranes (Basel), 11, 1 (2021).
4. F. Zhang, P. Zhao, M. Niu, and J. Maddy, Int J Hydrogen Energy, 41, 14535 (2016).
5. S. Sircar and T. C. Golden, in Hydrogen and Syngas Production and Purification Technologies, pp. 414–450, John Wiley & Sons, Inc. (2009).
6. D. Marciuš, A. Kovač, and M. Firak, Int J Hydrogen Energy, 47, 24179 (2022).
7. C. Jackson, L. F. J. M. Raymakers, M. J. J. Mulder, and A. R. J. Kucernak, Appl Catal B, 268, 118734 (2020).
8. D. Lee, S. Hwang, and I. Lee, J Power Sources, 145, 147 (2005).
9. J. Xu, S. Xiao, X. Xu, and X. Xu, Appl Energy, 318, 119214 (2022)
10. S. Ochi, O. Kamishima, J. Mizusaki, and J. Kawamura, Solid State Ion, 180, 580 (2009).
11. G. Venugopalan, D. Bhattacharya, E. Andrews, L. BricenoMena, J. Romagnoli, J. Flake, and C. G. Arges, ACS Energy Lett, 7, 1322 (2022).
12. K. H. Lim, I. Matanovic, S. Maurya, et al., ACS Energy Lett, 8, 529 (2022).
13. K. Arunagiri, A. Jark-Wah Wong, L. Briceno-Mena, et al., Energy Environ Sci, 16, 5916 (2023).
14. B. Keenan, Discover LANL (2023).
15. N. S. Patki, A. Manerbino, J. D. Way, and S. Ricote, Solid State Ion, 317, 256 (2018).
16. M. K. Hossain, R. Chandra, A. Eldenglawey, et al., Ceram Int, 47, 23725 (2021).
17. Y. Tong, Y. Wang, C. Cui, et al., J Power Sources, 457, 228036 (2020).
18. L. Blum, Electrochim Acta, 223, 100 (2017).
19. J. Pan, J. Yang, D. Yan, et al., Int J Hydrogen Energy, 45, 17927 (2020).
20. M. Hamdan, Electrochemical Compression, (2019).
21. The Royal Society, Ammonia: zero-carbon fertiliser, fuel and energy store (2020).
22. International Fertilizer Energy Association, Ammonia production: moving towards maximum efficiency and lower GHG emissions (2014).
23. A. Sathe, E. Groll, and S. Garimella, International Compressor Engineering Conference Proceedings (2008).
24. A. Giuffrida, Appl Therm Eng, 93, 813 (2016).
25. Y. Tao, W. Gibbons, Y. Hwang, R. Radermacher, and C. Wang, Chem Comm, 53, 5637 (2017)
26. A. Chouhan, U. R. Aryal, P. O. Sivakumar, B. Bahar, and A. K. Prasad, Int J Hydrogen Energy, 46, 37965 (2021).
27. J. Baker, L. Cao, Y. Hwang, C. Wang, and R. Radermacher, Int J Refrigeration, 153, 67 (2023).
28. R. Halseid, P. J. S. Vie, and R. Tunold, J Power Sources, 154, 343–350 (2006).
29. X. Fu, J. Zhang, and Y. Kang, Chem Catalysis, 2, 2590 (2022).
30. M. C. Hatzell, ACS Energy Lett, 7, 4132 (2022).
Stracensky and Xu (continued from previous page)
31. C. A. Fernandez, S. Varanasi, and M. Hatzell, Electrochem Soc Interface, 24, 51 (2015).
32. Y. Zeng, C. Priest, G. Wang, and G. Wu, Small Methods, 4 (2020).
33. X. Liang et al., Small Struct, 4 (2023).
34. C. Halliday and T. A. Hatton, Ind Eng Chem Res, 60, 9313 (2021).
35. K. M. Diederichsen, R. Sharifian, J. S. Kang, et al., Nature Reviews Methods Primers, 2, 68 (2022).
36. H. C. Mantripragada, H. Zhai, and E. S. Rubin, Int J Greenhouse Gas Control, 82, 59 (2019).
37.
38. A. Cousins, A. Cottrell, A. Lawson, S. Huang, and P. H. M. Feron, Greenh Gas Sci Technol, 2, 329 (2012).
39. L. Shi, Y. Zhao, S. Matz, et al., Nat Energy, 7, 238 (2022).
40. S. Voskian and T. A. Hatton, Energy Environ Sci, 12, 3530 (2019).
41. L. Caprile, B. Passalacqua, and A. Torazza, Int J Hydrogen Energy, 36, 10269 (2011).
42. S. Matz, L. Shi, Y. Zhao, et al., J Electrochem Soc, 169, 073503 (2022).
43. S. Afzal and A. Khan, Scientific World Journal, 2021 (2021).
44. C. A. Trickett, A. Helal, B. A. Al-Maythalony, et al., Nat Rev Mater, 2 (2017).
45. C. Bettenhausen, Chem Eng News, 99 (2021)
Section Name
Arizona Section
Brazil Section
Canada Section
Chile Section
Section Chair
Candace K. Chan
Raphael Nagao
Steen B. Schougaard
José H. Zagal
China Section Open
Detroit Section
Europe Section
Georgia Section
India Section
Israel Section
Japan Section
Korea Section
Mexico Section
Erik Anderson
Jan Macak
Seung Woo Lee
Sinthai Ilangovan
Daniel Mandler
Yasushi Idemoto
Won-Sub Yoon
Carlos E. Frontana Vázquez
Mid-America Section Nosang Myung
National Capital Section Chunsheng Wang
New England Section Sanjeev Mukerjee
Pacific Northwest Section Corie Cobb
Pittsburgh Section Open
San Francisco Section Gao Liu
Singapore Section
Zhichuan J. Xu
Taiwan Section Chi-Chang Hu
Texas Section
Twin Cities Section
Jeremy P. Meyers
Victoria Gelling
The ECS Honors & Awards Program recognizes outstanding technical achievement in electrochemistry, solid state science, and technology, along with exceptional service to the Society. Award opportunities are provided in the categories of Society Awards, Division Awards, Section Awards, and Student Awards.
Recognizing that today’s emerging scientists are the next generation of leaders in our field, ECS offers competitive fellowships and grants that enable students and young professionals to make discoveries and shape science long into the future.
Visit
Allen J. Bard Award: Established in 2013 to recognize distinguished contributions to electrochemical science, the award consists of a plaque containing a glassy carbon medallion; $7,500*; complimentary meeting registration for the award recipient and companion; dinner held in the recipient’s honor during the designated meeting; and ECS Life Membership.
Nomination deadline: April 15, 2024
ECS Toyota Young Investigator Fellowship: Established in partnership with the Toyota Research Institute of North America in 2015, the award encourages young professionals and scholars to pursue research into batteries, fuel cells, and hydrogen, and future sustainable technologies. Each year, at least one candidate receives the fellowship restricted grant of no less than $50,000 to conduct the proposed research within one year, and a one-year complimentary ECS membership. Recipients present at a Society biannual meeting and publish their research in a relevant ECS journal within 24 months of receiving the award.
Materials deadline: January 31, annually
Gordon E. Moore Medal for Outstanding Achievement in Solid State Science and Technology: Established in 1971 for distinguished contributions to the field of solid state science and technology, the award consists of a silver medal; plaque containing a bronze replica of the medal; $7,500; complimentary meeting registration for the award recipient and companion; dinner held in the recipient’s honor during the designated meeting; and ECS Life Membership.
Nomination deadline: April 15, 2024
John B. Goodenough Award: Distinguished contributions to the fundamental and technological aspects of electrochemical materials science and engineering are recognized, especially in the areas of batteries, solid ion conductors, fuel cells, transitionmetal oxides, and/or magnetic materials. Established in 2022, the award consists of a gold medal; plaque containing a bronze replica of the medal; $10,000; complimentary meeting registration for the award recipient and companion; dinner held in the recipient’s honor during the designated meeting; and ECS Life Membership.
Nomination deadline: June 15, 2024
Leadership Circle Awards: Established in 2002 to honor and thank our electrochemistry and solid state science partners, these awards are granted in the year that an institutional member reaches a milestone level. Awardees receive a commemorative plaque and recognition on the ECS website and ECS Interface magazine.
Nominations not accepted
Dielectric Science and Technology Division Thomas D. Callinan Award: The award, which was established in 1967, encourages excellence in dielectric investigations, the preparation of high-quality science and technology papers and patents, and publication in the Journal of The Electrochemical Society. Outstanding contributions to the field of dielectric science and technology are recognized by the award consisting of a framed certificate and $1,500.
Nomination period: March 15 – June 15, 2024
Electronics and Photonics Division Award: Established in 1969 to encourage excellence in electronics research and outstanding technical contribution to the field of electronics science, the award recognizes authors making noteworthy scientific contributions and enhancing the scientific stature of the Society in physics, chemistry, and metallurgy of electronic materials and devices by presenting well-received papers in Society journals and meetings. The award consists of a scroll; $1,500; and the choice of ECS Life Membership or up to $1,000 in meeting travel expenses.
Nomination period: March 15 – June 15, 2024
Energy Technology Division Research Award: Established in 1992 to encourage excellence in energyrelated research, the award consists of a framed certificate; $2,000; and ECS Energy Technology Division membership for the duration of the recipient’s ECS membership.
Nomination period: March 15 – June 15, 2024
Energy Technology Division Supramaniam Srinivasan Young Investigator Award: Established in 2011 to recognize and reward outstanding young researchers in the energy technology field, the award consists of a framed certificate; $1,000; and complimentary meeting registration.
Nomination period: March 15 – June 15, 2024
(continued on next page)
(continued from previous page)
Industrial Electrochemistry and Electrochemical Engineering Division New Electrochemical Technology (NET) Award: The Dow Chemical Company Foundation endowed the award in 1997 to recognize significant advances in industrial electrochemistry and promote high-quality applied electrochemical research and development. Recipients receive commemorative plaques and IE&EE Division Business Lunch tickets and are required to present a paper on their technology development at a designated meeting divisionsponsored symposium.
Nomination period: March 15 – June 15, 2024
Nanocarbons Division Robert C. Haddon Research Award: Established in 2018, the award encourages excellence in nanocarbon research and recognizes individuals making outstanding contributions to the understanding and applications of carbon materials. The award consists of a framed certificate; $1,000; and up to a maximum of $1,500 in meeting travel expenses.
Nomination period: March 15 – June 15, 2024
Pacific Northwest Section Electrochemistry Research Award sponsored by Gamry Instruments: Established in 2021, the award recognizes excellence in electrochemistry and solid state science and technology research by an independent scientist or engineer working in Washington, Oregon, or Idaho. The award consists of a framed certificate and $1,000.
Nomination deadline: July 15, 2024
Biannual Meeting Travel Grants: Many ECS divisions and sections offer travel grants to undergraduates, graduate students, postdoctoral researchers, young professionals, and faculty presenting papers at ECS biannual meetings. The awards consist of financial support ranging from complimentary meeting registration to luncheon/reception tickets, travel support, and more. Each division and section has its own application requirements.
PRiME 2024 Travel Grant applications accepted April 12 –July 8, 2024
Colin Garfield Fink Fellowship: First awarded in 1962, the fellowship supports research from June through August in a field of interest to the Society by a postdoctoral scientist/researcher. The award consists of $5,000 and publication of a summary report in the award year’s ECS Interface winter issue.
Materials deadline: January 15, annually
ECS General Student Poster Session Awards: This forum enables undergraduate and graduate students to present research results of general interest to ECS. Established in 1993, the session fosters and promotes work in electrochemical and solid state science and technology and stimulates active student interest and participation in ECS. Accepted posters are eligible for awards of $1,500 for 1st place, $1,000 for 2nd place, and $500 for 3rd place. To be considered for awards, authors must submit their abstracts to the Z01 General Student Poster Session; be accepted for inclusion in the session; upload a digital poster; and be present for in-person judging.
Materials deadline: The abstract deadline for the ECS Meeting in which the poster will be presented (April 12, 2024, for PRiME 2024)
ECS Outstanding Student Chapter Award: The award recognizes distinguished student chapters that demonstrate active participation in the Society’s technical activities; establish community and outreach activities in electrochemical and solid state science and engineering education; and create and maintain a robust membership base. The Outstanding Student Chapter receives a recognition plaque and certificates; $1,000; and award recognition and chapter group photo in ECS Interface or other electronic communications. Up to two Chapters of Excellence are also awarded.
Materials deadline: April 15, 2024
ECS Summer Fellowships: Established in 1928, up to four fellowships are awarded each year to support students’ research from June through August in a field of interest to ECS. The Edward G. Weston Fellowship, Joseph W. Richards Fellowship, F. M. Becket Fellowship, and H. H. Uhlig Fellowship winners receive $5,000 and publication of a summary report in the award year’s ECS Interface winter issue.
Materials deadline: January 15, annually
Energy Technology Division
Graduate Student Award
sponsored by BioLogic: Established in 2012 to recognize and reward promising young engineers and scientists in fields pertaining to the division, the award consists of a framed certificate; $1,000; complimentary student meeting registration; and admission to the division’s business meeting.
Nomination period: March 15 – June 15, 2024
Georgia Section Outstanding Student Achievement Award: Established in 2011, the $500 prize recognizes academic accomplishments by students pursuing PhDs at universities in the Georgia section region in any area of science or engineering in which electrochemical and/or solid state science and technology is the central consideration.
Nomination deadline: August 15, 2024
Industrial Electrochemistry and Electrochemical Engineering Division H. H. Dow Memorial Student Achievement Award: Established in 1990 to recognize promising young engineers and scientists in the field of electrochemical engineering and applied electrochemistry, the award consists of a framed certificate and $1,000 for education or research project-related expenses.
Nomination period: March 15 – June 15, 2024
Industrial Electrochemistry and Electrochemical Engineering Division Student Achievement Award: Established in 1989 to recognize promising young engineers and scientists in the field of electrochemical engineering, the award consists of a framed certificate and $1,000.
Nomination period: March 15 – June 15, 2024
Industrial Electrochemistry and Electrochemical Engineering Division Ralph E. White Outstanding Student Award: Established to encourage recipients to continue careers in electrochemical engineering or applied electrochemistry, the award of a certificate and $1,000 recognizes promising young engineers and scientists in the field of modeling and simulation of electrochemical systems.
Nomination period: March 15 – June 15, 2024
*All prize amounts are in US dollars.
Join us in celebrating your peers as we extend congratulations to all! The following awards are part of the ECS Honors & Awards Program, which has recognized professional and volunteer achievement in our multi-disciplinary sciences for decades.
Uziel Landau is Professor Emeritus of Chemical Engineering at Case Western Reserve University. His research combines fundamental analysis with practical applications and focuses on the study of deposit distribution and structure on macro and micro scales. Prof. Landau received his BSc (1964) and MSc (1968) from the Technion – Israel Institute of Technology. He completed his PhD (1975) at the University of California, Berkeley, with Prof. Charles Tobias, who, as his research advisor, introduced him to electrochemical engineering. After graduating, Prof. Landau joined Bell Laboratories where he developed plating processes. To combine teaching with research, Prof. Landau joined the Department of Chemical Engineering at Case Western Reserve University in 1977. He has taught and conducted research there for 45 years, serving as Department Chair from 2009 to 2016. Addressing the multi-disciplinary nature of electrochemical engineering, Prof. Landau developed a novel electrochemical engineering course specifically designed for students from different disciplines. The week-long condensed version of this course, offered annually, has attracted over 450 participants from industry, government, and academia. This short course has also been taught on site at numerous industrial corporations and universities.
Many of Prof. Landau’s former graduate students occupy leading industrial positions; others continue his legacy in academia. Prof. Landau is a Fellow of The Electrochemical Society and received the ECS Cleveland (now Mid-America) Section Ernest B. Yeager Electrochemistry Award, Intel Outstanding Researcher in Interconnects Award, and Case Western Reserve School of Engineering Innovation Award.
Daniel Lincot is Emeritus Researcher at the Centre national de la recherche scientifique (CNRS) and former Scientific Director of the Institut de recherche et développement sur l’énergie photovoltaïque (IRDEP). He began PhD studies in Solid State Physics in 1978, then joined CNRS at ChimieParisTech for a PhD in photoelectrochemistry of semiconductors, with a focus on interfacial energetics. Prof. Lincot’s passion for the fabrication of functional semiconductors from solution by electrodeposition or chemical deposition produced key results on CdTe, zinc oxide, and later CIGS. He broadened his research in photovoltaics with materials and device elaboration (atomic layer deposition and PVD) and characterization. At IRDEP from 2005 to 2019, Prof. Lincot started the industrial transfer of electrodeposition processes, culminating in the creation of the startup NEXCIS (2009 to 2015) with EDF Energies Nouvelles. In 2013, he pioneered the creation of the Institut Photovoltaïque d’Île de France
(IPVF), serving as scientific director until 2019. In 2021, he founded a startup to produce thin film solar cells and from 2021 to 2022, held the Industrial Innovation Chair on Photovoltaic Solar Energy and Energy Transition at the Collège de France.
Prof. Lincot received the 2020 Académie des Sciences’ Ivan Peychès Prix, 2015 Société Chimique de France Prix Pierre Süe, 2013 ECS Electrodeposition Division Research Award, 2011 Société Française de Métallurgie et des Matériaux Prix Charles Eichner, and 2004 Médaille d’argent du CNRS.
Peter Mascher holds the William Sinclair Chair in Optoelectronics at McMaster University. He leads research groups active in the fabrication and characterization of thin films for optoelectronic applications, the development and application of silicon-based nanostructures, and the characterization of defects in solids by positron annihilation spectroscopy. His research work has been continuously funded for more than 34 years, for a lifetime total surpassing $25M. He is a professional engineer and Fellow of the Canadian Academy of Engineering and The Electrochemical Society.
Prof. Mascher earned a PhD in Engineering Physics from the Technische Universität Graz (TUG) in 1984. Since joining McMaster in 1989 as a professor in the Department of Engineering Physics, he has supervised more than 85 graduate students and post-doctoral fellows and about 70 undergraduate research students. His many international collaborations create a vibrant and stimulating research environment and foster greater global awareness. Mascher has authored or coauthored close to 250 publications in refereed journals and conference proceedings and has presented many invited lectures at international conferences and workshops. His publications have been cited close to 5,000 times. He is Past Chair of ECS’s Dielectric Science and Technology Division, and since 2017, a Technical Editor for the ECS Journal of Solid State Science and Technology. He has co-organized many ECS symposia and is the lead organizer of a series of symposia titled “Nanoscale Luminescent Materials,” the 8th edition of which is scheduled for the 245th ECS Meeting.
Travis J. Anderson is the Head of the Power Electronics and Advanced Materials Branch at the US Naval Research Laboratory (NRL), where he leads a group of scientists studying fundamental material growth, device processing, and performance evaluation of all relevant power electronic materials from Si to diamond. In addition to his management duties, his research work focuses on wide bandgap III-N power (continued on next page)
(continued from previous page)
switches. He has expertise in processing, reliability, failure mechanisms, and radiation effects in Si, GaN, SiC, Ga2O3, diamond, and graphene-based devices. Dr. Anderson received a PhD in Chemical Engineering from the University of Florida in 2008, and a BS in Chemical Engineering from the Georgia Institute of Technology in 2004. He is the author of more than 250 publications and more than 370 presentations (>100 invited). He has been awarded 41 patents. He is a recipient of the 2014 and 2022 Edison Awards for best NRL patent, 2022 NRL Technology Transition Award, and 2016 Dolores M. Etter Top Navy Scientist Award.
William Mustain is a Professor in the Department of Chemical Engineering and Associate Dean for Research in the College of Engineering and Computing at the University of South Carolina. He works in areas related to electrochemical energy generation and storage, including high capacity materials for Li-ion batteries, novel electrode structures for Li-S batteries, corrosion and passivation of materials, catalysts and supports for proton exchange membrane and anion exchange membrane fuel cells and electrolyzers, electrochemical reactor design and electrochemical synthesis of fuels. He has published more than 140 peer-reviewed articles (h-index 50) and has given more than 100 invited lectures. Throughout his career, Prof. Mustain has been highly active in The Electrochemical Society, serving in many roles, including as Chair of the Energy Technology Division and Chair of the Individual Membership Committee. He has received awards that including the US Department of Energy Early Career Research Program Award, Connecticut Quality Improvement Platinum Award, ECS Energy Technology Division Supramaniam Srinivasan Young Investigator Award, UConn Chemical Engineering Faculty of the Year Award, University of South Carolina Chemical Engineering Publication Award and College of Engineering and Computing Research Achievement Award, Illinois Institute of Technology Young Alumnus Award, and Fulbright Scholar Fellowship.
Sponsored by Bio-Logic
Noor Ul Hassan recently joined the National Renewable Energy Laboratory (NREL), Chemistry and Nanoscience Center as a postdoctoral researcher with Dr. Bryan Pivovar. His research in the Hydrogen and Fuel Cells group at NREL explores the science behind polymer electrolyte fuel cells and water electrolyzer systems, specifically focusing on improving performance and durability. He received his PhD in Chemical Engineering from the University of South Carolina with Professor William Mustain, investigating various component level aspects of fuel cell and water electrolyzer systems such as electrode engineering and optimization, ionomeric binders, catalyst ink rheology, and porous transport layers. While working on his PhD thesis, he
collaborated with US universities and national labs as well as foreign institutions in developing fuel cells and water electrolyzers. For his MSc at İstanbul Şehir Üniversitesi under the supervision of Prof. Bahadir Tunaboylu and in collaboration with TÜBİTAK Marmara Araştırma Merkezi and Gebze Teknik Üniversitesi, he developed proton exchange membrane fuel cell stacks for small air-breathing unmanned aerial vehicles.
Maha Yusuf is a Presidential Postdoctoral Research Fellow in the Department of Mechanical and Aerospace Engineering and Andlinger Center for Energy and Environment at Princeton University. During her postdoc research, she is combining her expertise in advanced neutron and X-raybased characterization with physics-based modeling and 3D manufacturing to engineer anodes for long-life lithium-metal–free solid state batteries. She is co-advised by Prof. Kelsey Hatzell (Assistant Professor of Mechanical and Aerospace Engineering and Andlinger Center for Energy and the Environment, Princeton University) and Prof. Craig Arnold (Susan Dod Brown Professor of Mechanical and Aerospace Engineering, and Vice Dean for Innovation, Princeton University). Dr. Yusuf completed an MSc and PhD degrees in Chemical Engineering at Stanford University with support from the Schlumberger Faculty for the Future Fellowship and Diversifying Academia, Recruiting Excellence Fellowship. Advised by Prof. Michael F. Toney and Dr. Johanna Nelson Weker, her PhD research used advanced imaging diagnostic tools, particularly neutrons and X-rays, to understand the failure mechanisms of lithium-ion batteries during extreme fast charging (XFC). Dr. Yusuf’s received awards include the 2022 ECS Edward G. Weston Fellowship, 2022 American Chemical Society Chemical Abstracts Service Future Leader Award, and 2020 Stanford Distinguished Student Energy Lecturer Award. Dr. Yusuf holds a BE in Chemical Engineering from National University of Sciences & Technology, Pakistan, following which she worked as a drilling engineer on oil and gas rigs in Colombia.
Nikolay Kornienko is a Full (W3) Professor in the Institut für Anorganische Chemie, Universität Bonn. His team’s overarching motivation is to discover and implement the chemistry necessary to transition to a sustainable energy-based society. Specifically, the lab develops materials geared to convert renewable electricity to chemical fuels as energy storage media and innovates operando methods to probe them in their course of action. Prof. Kornienko completed his BSc studies at the University of Pittsburgh where he first experienced scientific research in the lab of Prof. Sanford Asher, graduating in 2011. He completed his PhD in 2016 in the lab of Prof. Peidong Yang, focusing on materials and energy research. Prof. Kornienko received a Royal Society Newton Fellowship and investigated biobased energy conversion in the lab of Prof. Erwin Reisner at the University of Cambridge from 2016 to 2018. In 2019, Prof. Kornienko was appointed Assistant Professor at the Université de Montréal and promoted to Associate Professor with tenure in 2022. He moved to the Universität Bonn in 2023.
Yirui Zhang is a Schmidt Science Fellow in the Dionne Lab at Stanford University, leveraging electrokinetics and plasmonics for biophotonics and catalysis. Her research has focused on electrode-electrolyte interfaces in Li-ion batteries and fuel cell reactions, where she developed in-situ characterizations and electrochemical methods to understand the interfacial reaction pathways and charge transfer kinetics during electrochemical reactions. Her contributions include uncovering electrolyte oxidation pathways in Li-ion batteries using in-situ Fourier transform infrared spectroscopy, demonstrating a coupled-ion electron transfer mechanism for Li-ion intercalation, and elucidating the mechanistic role of water structures and hydrogen bonding in shifting the protoncoupled electron transfer reaction kinetics. Her research aims to establish design principles for electrolytes, offering insights beyond solid electronic structures to tailor electrochemical reaction activity and kinetics.
Dr. Zhang obtained a BS in Mechanical Engineering from Tsinghua University in 2017 and completed a PhD in Mechanical Engineering in 2022 at the Massachusetts Institute of Technology under the guidance of Prof. Yang Shao-Horn. Her research accomplishments have been recognized through the ECS Energy Technology Division Graduate Student Award, MRS Graduate Student Award, and the Schmidt Science Fellowship, among other honors.
Deepra Bhattacharya is a research scientist at GE Vernova. Prior to completing his PhD, he worked on post-combustion carbon capture membranes at the University of Manchester and on solid oxide fuel cells at the Central Glass and Ceramic Research Institute (CSIR). He obtained his PhD at The Pennsylvania State University, developing morphology controlled precious metal nanostructures aided by block copolymer self-assembly to probe the dependence of catalyst electroactivity on geometry. After completing his PhD, he worked briefly on carbon dioxide electroreduction at the National Renewable Energy Laboratory as a postdoctoral researcher.
R. Bruce Weisman is Professor of Chemistry and of Materials Science and NanoEngineering at Rice University. His current research focuses on basic and applied studies of single-wall carbon nanotubes using the methods of experimental spectroscopy and photophysics. Prof. Weisman is best known for leading the discovery and spectral interpretation of near-infrared fluorescence from carbon nanotubes.
Prof. Weisman earned his BSc in Chemistry from Johns Hopkins University and his PhD in Chemistry from the University of Chicago before postdoctoral studies at the University of Pennsylvania. He then joined the faculty of Rice University. Prof. Weisman has been a National Science Foundation Graduate Fellow, Hertz Foundation Graduate Fellow, National Science Foundation Postdoctoral Fellow, and Arthur P. Sloan Research Fellow. He has held the Green Lectureship at Texas Christian University and the Xingda Lectureship at Peking University. Prof. Weisman is a Fellow of The Electrochemical Society, American Physical Society, and American Association for the Advancement of Science.
As a member of ECS for 30 years, Prof. Weisman has been a frequent symposium organizer and served in all elected positions in the Nanocarbons Division and on several of the Society’s standing committees. He co-edited the journal Applied Physics A and is currently on the editorial board of Scientific Reports and a Series Editor of the Handbook of Carbon Nanomaterials. In addition to his academic activities, Prof. Weisman has since 2004 been the founder and president of Applied NanoFluorescence, LLC, a company that designs, constructs, and sells specialized spectroscopic instruments. These systems enable advanced characterization of nanomaterials in academic, industrial, and government laboratories around the world.
Delphine Bouilly is Associate Professor in Physics and Principal Investigator at the Institut de recherche en immunologie et en cancérologie (IRIC) at the Université de Montréal. She currently holds the Canada Research Chair in Bionanoelectronics and leads a multidisciplinary research group focusing on nanoelectronic sensors based on carbon nanotubes and graphene, as well as their application in fundamental biophysics and biomedical technology. Her interdisciplinary approach draws from materials physics, surface chemistry, device engineering, and molecular biology. Prof. Bouilly has made noteworthy contributions to the field of nanocarbon materials, particularly in understanding the effect of covalent surface chemistry on the electronic properties of these materials, and in applying nanocarbon devices for singlemolecule measurements and biosensing.
Prof. Bouilly earned a BSc (2007) and PhD (2013) in Physics from the Université de Montréal, under the guidance of Prof. Richard Martel. Her graduate work investigated the interplay between surface chemistry and electrical properties in functionalized carbon nanotubes. She then joined Prof. Colin Nuckolls’ research group at Columbia University as a Postdoctoral Fellow (2013–2016) developing ultraminiaturized device architectures based on carbon nanotubes and graphene for single-molecule experiments. Prof.
(continued on next page)
Bouilly has received notable awards, including Canada’s National Sciences and Engineering Research Council (NSERC) Vanier, Banting, and André Hamer Awards.
Habin Park is a PhD candidate in Chemical Engineering at the Georgia Institute of Technology (Georgia Tech). His research interests are sustainable energy conversion and storage via polymer electrolytes with a focus on developing chemically and physically durable anion-exchange membrane low-temperature alkaline water electrolysis. Habin received a BS in 2017 and an MS in 2019 from the University of Seoul (UOS). His MS thesis was titled “Design of High-Performance Supercapacitor by Rearrangement of Activated Carbon Electrodes and Methacrylate-based Gel Polymer Electrolytes.” He worked in the Polymer Electrochemistry Laboratory as a graduate research assistant, focusing on developing gel polymer electrolytes for supercapacitors to improve performance at elevated temperature and voltage. As a researcher for half a year at the UOS Institute of Industrial Technology, he worked on improving the thermal stability of acetonitrile-based electrolytes for enabling reflow-soldering. Habin went from there to Georgia Tech where he is co-advised by Prof. Nian Liu and Prof. Paul A. Kohl on researching advanced solid-state lithium-ion batteries focused on 3D electrodes using high conductivity solid polymer electrolytes. In 2022, he transitioned to water electrolysis projects led by Prof. Kohl.
Fengyi Zhao is a PhD candidate in Chemistry at Emory University under Prof. Tianquan Lian’s supervision. His research focuses on the development of in-situ spectroscopic methods to examine semiconductor-electrolyte junctions in photoelectrochemical processes using techniques such as transient absorption spectroscopy (TAS), in-situ Raman spectroscopy, and second harmonic generation (SHG). These tools enable him to investigate the intricate details of electron transfer processes from semiconductors to catalysts at the early timescale and to monitor the changes in surface molecules and electric fields during the reactions. Fengyi completed his BS at Sun Yat-sen University. Beyond his academic pursuits, Fengyi is an avid sports enthusiast, particularly enjoying skiing, basketball, and badminton, and finds solace in cooking.
Hui (Claire) Xiong is Professor in the Micron School of Materials Science and Engineering at Boise State University. Her research focuses on defect engineering and interface/interphase engineering of battery materials, Li-ion and Na-ion batteries and beyond, ion irradiation effects on electroceramics, mechanistic insights on electrolyte degradation, and in-situ and operando characterizations.
Dr. Xiong received her BE degree in Applied Chemistry (1998) and MS degree in Inorganic Chemistry (2000) from East China University of Science and Technology, and her PhD in Electroanalytical Chemistry (2007) from the University of Pittsburgh. From 2008 through 2012, she conducted postdoctoral work at Harvard University and Argonne National Laboratory where her research involved electrochemical characterization of micro-fabricated cathode materials for micro-solid oxide fuel cells and the development of novel nanostructured metal oxide electrode materials for rechargeable batteries. She joined Boise State’s faculty in 2012. Dr. Xiong has authored and co-authored 70 peer-reviewed publications and written four book chapters. She holds two US patents and has one US patent application. Dr. Xiong received a 2015 NSF CAREER Award and is a Fellow of the Royal Society of Chemistry, Fellow of the American Ceramic Society, Scialog Fellow, and Fellow of the Center for Advanced Energy Studies.
WINNER:
Sang Cheol Kim, Stanford University
Sang Cheol Kim is a Stanford Energy Postdoctoral Fellow in Prof. Steven Chu’s group, working on novel electrochemical solutions for tackling energy and sustainability challenges. He recently completed his PhD in Materials Science and Engineering at Stanford University. His research focuses on electrolytes for electrochemical energy storage, particularly lithium metal batteries. He has made numerous contributions to the field, including the development of novel potentiometric techniques for electrolyte solvation characterization, data-driven methods that led to new insights and state-of-the-art cycling performance for lithium metal anodes, and design of the high entropy electrolyte for long-cycling and fastcharging lithium metal batteries. In addition to his scientific contributions, Dr. Kim is passionate about energy policy, having served as a Stanford Emerging Technology Review Fellow and on the panel at the Trans-Pacific Sustainability Dialogue. He has served as a Session Chair at the 242nd ECS Meeting, Stanford MSE Rising Stars Committee Co-Chair, Stanford StorageX Industrial Student Liaison, and President of the Korean Student Association at Stanford.
HONORABLE MENTION:
John Petrovick, University of California, Berkeley
John Petrovick is a postdoctoral researcher in the Chemical Engineering Department at Kungliga Tekniska Högskolan (KTH), advised by Prof. Göran Lindbergh. Dr. Petrovick’s research focuses primarily on understanding the role of liquid electrolyte in the performance of anion exchange membrane water electrolyzers. This is investigated via the experimental characterization of, and modeling of, transport phenomena within the catalyst layer. He completed his PhD at the University of California, Berkeley under the supervision of Dr. Adam Weber and Prof. Clay Radke. When not in the lab, John likes to read, hike, and play ultimate frisbee and all types of games. (continued
ECS is proud to announce the new members for October, November, and December 2023 (Members are listed alphabetically by family/last name.)
Lutz Ackermann, Göttingen, NI, Germany
Ayoob Alhammadi, Dubai, Abu Dhabi, UAE
Rajeev Assary, Naperville, IL, USA
C
Wenlong Cheng, Clayton, VIC, Australia
Jung-Il Choi, Seodaemun-gu, Seoul, ROK
G
Ricardo Galván Martínez, Boca del Rio, Veracruz, México
Mark Goorsky, Los Angeles, CA, USA
Matthew Jones, Sterling Heights, MI, USA
K
Dmitri Kilin, Fargo, ND, USA
Svetlana Kilina, Fargo, ND, USA
Jongsoon Kim, Suwon, Gyeonggi-do, ROK
Nattanai Kunanusont, Khlong Nueng, Pathum Thani, Thailand L
Donald Luke, Lakeland, FL, USA
M
Chong-Geng Ma, Chongqing, Chongqing, China
Hai-bin Ma, Singapore, Singapore, Singapore
Julia Maibach, Gothenburg, Vastergotland, Sweden
Tomoyo Manaka, Toyama City, Toyama, Japan
Kevin Martin, Easton, PA, USA
Fabiana Marzano, L´Isle Adam, Val-d’Oise, France
Erika Méndez-Albores, Puebla, Puebla, México
P
Alexander Papandrew, Farmington, CT, USA
Iliyan Popov, Sofia, Sofia City, Bulgaria
S
Ken-ichi Saitow, Higashi Hiroshima, Hiroshima, Japan
T
Haibin Tang, Hefei, Anhui, China
Yosuke Tani, Toyonaka, Osaka, Japan
Andre Taylor, Brooklyn, NY, USA
K. George Thomas, Vithura, KL, India
Haining Tian, Uppsala, Uplandia, Sweden
Iurii Timrov, Villigen, AG, Switzerland
Anju Toor, Atlanta, GA, USA
Daniel Torelli, Guildford, England, UK
U
Aki Ushiku, Chuo-ku, Tokyo, Japan
W
Hidenobu Wakita, Morioka, Iwate, Japan
Tza-Huei Wang, Baltimore, MD, USA
Xuehang Wang, Delft, South Holland, Netherlands
Jamie Weaver, Jefferson, MD, USA
Björn Wickman, Gothenburg, Vastergotland, Sweden
Y
Neslihan Yuca, Istanbul, Istanbul, Turkey
Haimei Zheng, Berkeley, CA, USA
A
Mohamed Abdelsalam, Cairo, Cairo, Egypt
Emily Abdo, Encinitas, CA, USA
Nick Adams, Concord, CA, USA
Peace Adesina, Cambridge, Cambridgeshire, UK
Barıs Adıguzel, Istanbul, Istanbul, Turkey
Toprak Aktaş, Atasehir, Istanbul, Turkey
Mukarram Ali, Changwon, Gyeongsangnam-do, ROK
Islam Aly, Giza, Giza, Egypt
Isabel Antony, Oxford, Oxfordshire, UK
Ridwan Ayinla, Starkville, MS, USA
BMohamed Ahmed Baba, Kaunas, Kaunas County, Lithuania
Arunraj Balaji-Wright, Pleasanton, CA, USA
John Balseiro, South Miami, FL, USA
Akichika Banno, Nagoya, Aichi, Japan
Ananya Bansal, Roorkee, UT, India
Sheraz Bashir, Potsdam, NY, USA
Nila Beamon, New Orleans, LA, USA
Erling Beck, Richardson, TX, USA
Katherine Betts, Henderson, NV, USA
Abhirup Bhadra, Sydney, NSW, Australia
Prashant Bhargav, Kanpur, UP, India
Colton Bruni, Gulfport, MS, USA
Boya Cao, Cambridge, Cambridgeshire, UK
Tyson Carr, Seattle, WA, USA
Matthew Chagnot, Raleigh, NC, USA
Jaewoong Choi, Seoul, Gyeonggi-do, ROK
César Coello, Querétaro, Querétaro Arteaga, México
Shashwati da Cunha, Austin, TX, USA
John Mark Christian Dela Cruz, Budapest, Central Hungary, Hungary
Itzel Díaz, Corregidora, Querétaro Arteaga, México
Sepanta Dokhani, Boras, Vastergotland, Sweden
Mary Ebbert, Pittsburgh, PA, USA
Lisa Eggart, Houghton, MI, USA
Dalia Elgendy, Cairo, Cairo, Egypt
Meva Elitas, Cekmekoy, Istanbul, Turkey
Hosni Elwan, Newcastle upon Tyne, England, UK
Elisa Emanuele, Buccinasco, Lombardia, Italy
Zachary Feng, Austin, TX, USA
Natalia Firlej, Warsaw, Mazowieckie, Poland
Leon Focks, Münster, NRW, Germany
Filippo Franceschini, Leuven, Leuven, Belgium
Bouho Franck, Poitiers, Nouvelle-Aquitaine, France
Matthew Friskey, Dundee, Scotland, UK
Ariadna García Caballero, Pedro Escobedo, Querétaro Arteaga, México
Carol-Lynn Gee, Burnaby, BC, Canada
Michael Geiwitz, West Boylston, MA, USA
Ryan Gettler, Columbia, MO, USA
Alicja Głaszczka, Warsaw, Mazowieckie, Poland
Jean-Pierre Glauber, Bochum, NRW, Germany
Johanna Gonzalez, Lubbock, TX, USA
Adora Goodluck, Houston, TX, USA
Hubert Grabowski, Kolbudy, Gdańsk, Poland
Sarp Güngör, Istanbul, Istanbul, Turkey
Ning Guo, Berkeley, CA, USA
Yiming (Bill) Guo, London, England, UK
Saptarshi Gupta, Guwahati, Assam, India
Chengrui Han, Miami, FL, USA
Hinaka Hashimoto, Sanda, Hyogo, Japan
Sulagna Hazarika, Austin, TX, USA
Arvind Singh Heer, Cleveland Heights, OH, USA
Hugo Hernandez Ortega, Monterrey, Nuevo León, México
Hannah Hilton-Tapp, Leicester, England, UK
Alexander Hoffmann, Brighton, MI, USA
(continued from previous page)
Mamoru Honda, Sanda, Hyogo, Japan
Shifeng Hong, Ithaca, NY, USA
Rayhan Hossain, Stillwater, OK, USA
J
Amitesh Jayaraman, Stanford, CA, USA
Jizhou Jiang, Mishawaka, IN, USA
KKanyawee Kaewpradub, Meuang, Southern Thailand, Thailand
Chika Kano, Nagoya, Aichi, Japan
Vamika Kapur, Waterloo, ON, Canada
Nawaraj Karki, Louisville, KY, USA
Devadharshini Kathan, Columbia, SC, USA
Kausthubharam Kausthubharam, West Lafayette, IN, USA
Josef Keilhofer, Garching, BY, Germany
Marta Klepacka, Trondheim, Trondelag, Norway
Abbey Knoepfel, State College, PA, USA
Katja Kress, Sheffield, Yorkshire & the Humber, UK
Mukesh Kumar, Kanpur, UP, India
Raj Kumar, Jamshoro, Sindh, Pakistan
Uday Kumar, Chennai, TN, India L
Jaeheon Lee, Berkeley, CA, USA
Kyu Lee, Waterloo, ON, Canada
Dania Leyva Ruiz, Querétaro, Querétaro Arteaga, México
Weihao Li, Glasgow, Scotland, UK
Wei Ying Lieu, Singapore, Singapore, Singapore
Jiaxin Liu, Philadelphia, PA, USA
M
Mallikarjun Madagalam, Torino, Piemonte, Italy
Prottay Malakar, Ann Arbor, MI, USA
Iain Malone, London, England, UK
Toshiki Masago, Sanda, Hyogo, Japan
Federico Mattera, Copenhagen, Hovedstaden, Denmark
Bengu Mete, Lubbock, TX, USA
Kepei Miao, Coral Gables, FL, USA
Ivan Mondaca-Medina, Austin, TX, USA
Sebastian Mori Huerta, Querétaro, Querétaro de Arteaga, México
Zyanya Mota Davila, San José Iturbide, Guanajuato, México
Jesy Motchaalangaram, Hattiesburg, MS, USA
Jethrine Mugumya, Richmond, VA, USA
Muhammad Abdun Nafi, Lafayette, IN, USA
Nchumi Sah Ndaleh, Oxford, MS, USA
Mayu Negishi, Nagoya, Aichi, Japan
Leah Nuss, Garching, BY, Germany
OOriyomi Ogunbanjo, Birmingham, England, UK
Sayaka Okada, Sanda, Hyogo, Japan
Tatsunori Okamura, Sanda, Hyogo, Japan
Mehmetcan Öksüz, Ankara, Ankara, Turkey
Luis Oxenfart, Oberschleißheim, BY, Germany
Kerem Özmert, Istanbul, Istanbul, Turkey
PLujin Pan, Berlin, BE, Germany
Xian Pan, Coral Gables, FL, USA
Mudit Panda, Fort Worth, TX, USA
Sathiya Priya Panjalingam, Münster, NRW, Germany
Florian Parhofer, Garching, BY, Germany
Sean Peyres, White Heath, IL, USA
Philipp Pfändner, Garching, BY, Germany
Daniel Poposki, Gothenburg, Vastergotland, Sweden
Klaudia Prusik, Gdańsk, Pomerania, Poland
QJing Qin, Coral Gables, FL, USA
Carlos Quintero, Peterborough, ON, Canada
Micah Quirie, Lincoln, NE, USA
RRohit Raj, Austin, TX, USA
Manasa Rajeev, Eugene, OR, USA
Basirat Raji-Adefila, Albuquerque, NM, USA
Silvia Rangel Duarte, Querétaro, Querétaro de Arteaga, México
Justin Rastinejad, Berkeley, CA, USA
Mahmoud Reda, Vienna, Vienna, Austria
Marcus Ringström, Kungsbacka, Halland, Sweden
Daria Roda, Gdańsk, Pomerania, Poland
Sankhadip Saha, Randwick, NSW, Australia
Devin Schinski, Berkeley, CA, USA
Joshua Schut, Edmond, OK, USA
Ahmed Shafik, Cairo, Cairo, Egypt
Sange Sherpa, Chennai, TN, India
Alper Söğüt, Istanbul, Istanbul, Turkey
Erick Soto Hernandez, Querétaro, Querétaro de Arteaga, México
Elisabeth Springl, München, BY, Germany
Paweł Stepnicki, Warsaw, Warsaw, Poland
David Strickland, Redlands, CA, USA
Suganthan Suriyamoorthy, Ann Arbor, MI, USA
Francesco Tavola, Santa Maria Hoè, Lombardia, Italy
Greta Thompson, London, England, UK
Isaac Triplett, Eugene, OR, USA
Zachary Tronstad, Albany, CA, USA
Bedreddin Boran Turan, Istanbul, Istanbul, Turkey
Arslan Umer, Dallas, TX, USA
İnci Varol, Sancaktepe, Istanbul, Turkey
Scarleth Vasconcelos, Bryn Mawr, PA, USA
Eduardo Veliz Gonzalez, Guadalupe, Nuevo Léon, México
Paola Vilchis Gutiérrez, El Marqués, Querétaro de Arteaga, México
Yue Wen, London, England, UK
Wanrong Xie, Durham, NC, USA
Archie Yao, South Lyon, MI, USA
Daiwei Yao, Miami, FL, USA
Arma Yau Musa, Gothenburg, Vastergotland, Sweden
Hao Yu, Waterloo, ON, Canada
Wan-Ju Yu, Hsinchu, Hsinchu County, Taiwan
Hanna Zakiyya, Miskolc, Northern Hungary, Hungary
Zuzanna Zarach, Gdańsk, Pomerania, Poland
Davy Zeng, Ann Arbor, MI, USA
Elia Zonta, Münich, BY, Germany
What inspired you to join?
“ECS has established a remarkable platform, bringing together diverse backgrounds of individuals to collaboratively contribute to electrochemistry.”
Which member benefit is the most valuable to you?
“Certainly networking, exchanging ideas, and collaborating with our ECS community.”
New Members by Country Look who joined ECS in the Fourth Quarter of 2023.
Australia
Austria
Belgium
Bulgaria
Canada
China
Denmark
Egypt
France
Germany
Hungary
India
Italy
Japan
Lithuania
Mexico
Netherlands
Norway
Pakistan
Poland
Singapore
South Korea
Sweden
Switzerland
Taiwan
Thailand
Turkey
Science is increasingly globalized. Since the late 20th century, collaborative research, often with international partners, has emerged as a cornerstone of cuttingedge scientific discoveries.1 This paradigm shift is best illustrated by the Nobel Prize, which in recent years has been increasingly conferred upon collaborative groups whose combined efforts have ushered in transformative breakthroughs.
International conferences play a vital role in initiating and maintaining scientific collaborations, standing out as a go-to platform for sharing latest research developments, connecting leading experts, and establishing connections that can translate into future collaborative ventures.2,3 As global collaboration gains importance in the contemporary science landscape, international conferences are emerging as key players, forging connections across borders and fostering a global network aimed at collectively addressing shared problems. Diverse geographic and cultural perspectives not only facilitate the generation of novel ideas, but also pave the way for unconventional, groundbreaking solutions.3
Graduate students can play a crucial role in enabling the worldwide exchange of knowledge, by helping initiate and sustain scientific collaborations. Attending an international conference offers student participants a unique opportunity to showcase their research on a global stage, elevating their visibility and bolstering their academic standing. The conference setting can expand their network beyond the confines of their home institutions, providing a platform to connect with peers from around the world. Engaging with researchers of diverse backgrounds can also foster an appreciation of varied scientific and cultural perspectives. This exposure holds the potential to challenge pre-existing assumptions and enrich students’ understanding of their research areas. Beyond academic benefits, these conferences can become avenues for students to establish connections with potential mentors, paving the way for global career opportunities. Further, international conferences offer students a chance to explore a new country, interact with locals, and get a taste of a different culture. This exposure can add an extra dimension to their overall learning experience, contributing to personal development alongside academic and professional advancement.
As the current generation of students transitions into the future workforce, their active involvement in addressing global challenges is paramount. At the forefront of these challenges is the pressing issue of climate change. The 2023 National Academies of Sciences, Engineering and Medicine (NASEM) report4 emphasizes that electrochemical pathways toward decarbonization are poised to play a critical role in making renewable energy affordable and accessible to all sectors of society.5 Future leaders, emerging from today’s students, can play a key role in shaping energy policies that extend from grassroots initiatives to the highest echelons of governance, thus facilitating an equitable, just, and inclusive clean energy transition for a sustainable future.
Against the backdrop of the ongoing energy transition, we present our experience from the recently concluded 244th Meeting of The Electrochemical Society (ECS) held in Gothenburg, Sweden in October 2023, captured through the lens of a scientific travelogue by one of the authors, ECS Student Ambassador Debanjali Chatterjee. This narrative aims to illuminate the profound impact that international experiences of this nature can have on both graduate students and mentors alike.
Sweden stands out as a global leader in sustainability, with the city of Gothenburg earning the remarkable distinction of being named the world’s most sustainable destination in 2023 by the Global Destination Sustainability Index. The commitment to sustainability is evident from the moment one enters the country, with both Stockholm and Gothenburg airports showcasing Volvo electric cars plugged into charging stations—a fitting nod to Sweden being the birthplace of Volvo. More surprising are messages encouraging recycling on commonplace items like coffee cups and paper napkins. Tap water in Sweden is perfectly safe for drinking, and water filters at the airport go a step beyond by clearly stating where their water is naturally sourced from. All this shows that sustainability is not just a buzzword for Sweden, but is ingrained into the Swedish way of life. This further justifies why Sweden is an most apt location for a conference on electrochemistry for energy storage systems.
The ECS meeting was held from October 8 to 12, 2023, at the Swedish Congress and Exhibition Centre. Research highlights from the authors’ institution, the Purdue University Energy and Transport Sciences Laboratory (ETSL), were an invited keynote talk on thermo-electrochemical interactions in solid-state batteries6, 7 by Partha Mukherjee; oral presentations on mechanistic underpinnings of heterogeneities in solid-state battery cathode8 and on void growth dynamics in solid-state batteries9 by PhD candidate Kaustubh G. Naik; and an oral presentation on mechanics-coupled interface kinetics in solid-state batteries by Debanjali Chatterjee10. The enthusiasm surrounding these presentations was evident to us in the impressive attendance and the thought-provoking questions and discussions that followed. This engagement not only enhanced the exchange of ideas but also fostered the establishment of new contacts, laying the foundation for potential research collaborations.
The ECS plenary and award lectures by distinguished researchers were another highlight of the conference for us. The presentations on batteries and energy storage systems, with a particular focus on lithium-ion and next-generation batteries, electrochemical interfaces, and battery safety, provided valuable insights into the latest advances in the field. As a Symposium Travel Grant awardee, it was an honor to be invited to the A07 Symposium Luncheon on the Interplay between Temperature and Battery Phenomena, which provided the opportunity to interact and reconnect with research peers, many of those connections having been formed in previous ECS meetings.
A novel aspect of Debanjali’s experience was her role as an ECS Student Ambassador, where she worked at the registration desk assisting conference attendees with badge pick-up. This was an excellent avenue to network with fellow ambassadors, and also reconnect with professors she hosted at Purdue while serving as the President of the ECS Purdue Student Chapter.
Gothenburg is a beautiful city with a relaxed, rustic vibe. We saw electric buses and trams crisscrossing the city center, living up to its sustainable standards. We visited the beautiful Horticultural Garden of Gothenburg, which is located right in the heart of the city. Walking around on the cobblestone paths with European architecture all around was a treat for the mind and soul.
When in Europe, immersing oneself in the charm of quaint cafés is a must, and Sweden offers an exceptional setting for this delightful experience through the Swedish tradition of fika. This cherished practice involves setting aside a part of the day to enjoy coffee and a snack while socializing. We found that there could be no better place to experience fika than Café Husaren, an iconic landmark nestled in the heart of Gothenburg’s old city, Haga.
Café Husaren is globally renowned for its colossal, freshly baked cinnamon buns known as hagabullen. A cortado paired with a cinnamon bun that rivals the size of one’s face created a heavenly breakfast. Adding to its charm, the building in which the café is housed is a piece of history in itself—it is one of the oldest buildings in Haga and is protected by the Swedish Heritage Act. The preserved glass and stucco buildings date back to the late 19th century!
We learned that Gothenburg boasts a dynamic and diverse food scene. The city’s culinary offerings span the globe, showcasing a rich tapestry of cuisines. We were able to sample both fine-dining experiences, complemented by breathtaking aerial views of the city, and delectable local renditions of Indian, Thai, and Italian cuisine. We especially enjoyed indulging in the local dairy delights. From sumptuous cheese platters to decadent cheesecakes and the quintessential Swedish bread, these milk-based delicacies proved to be irresistible must-haves.
The 244th ECS Meeting in Gothenburg not only provided invaluable insights into the latest advances in electrochemical energy storage and conversion, but also brought to our attention a city that exemplifies sustainability and innovation at its core. From engaging discussions and inspiring presentations to forging new connections and the opportunity to contribute as an ECS Student Ambassador, the conference marked a defining experience in this student’s academic journey. From a mentor’s perspective, exposing graduate students to an international scientific community in the emerging era of energy transition made for a unique and lasting experience that can profoundly shape the thought leaders of tomorrow. The
picturesque surroundings, cultural experiences, and culinary delights of Gothenburg added a layer of richness to this adventure. For both of us, this international conference experience was a transformative journey, symbolizing the convergence of cutting-edge research, cultural exploration, and personal growth.
Debanjali expresses her sincere thanks to Prof. Partha P. Mukherjee for giving her the opportunity to attend this highly impactful international conference. She also extends her gratitude to the organizers of Symposium A07 (Interplay between Temperature and Battery Phenomena), especially Dr. Rachel Carter from the US Naval Research Laboratory, for supporting her through a Travel Grant award.
Debanjali Chatterjee is a PhD student at the Energy and Transport Sciences Laboratory, School of Mechanical Engineering, Purdue University. Her research focus lies in unveiling the mechanisms that govern interfacial stability in solid-state batteries using mesoscale modeling and analytics.
Partha P. Mukherjee is a Professor of Mechanical Engineering, University Faculty Scholar, and Director of the Energy and Transport Sciences Laboratory, Purdue University. His research interests lie at the confluence of transport, interface, and materials aspects in electrochemical energy storage and conversion.
Debanjali Chatterjee: @_debanjali_
Partha P. Mukherjee: @ParthaPMukherj1 Energy and Transport Sciences Laboratory (ETSL): @etslpurdue
1. Y. Dong, H. Ma, Z. Shen, and K. Wang, Proceedings of the 23rd ACM SIGKDD International Conference on Knowledge Discovery and Data Mining, 1437 (2017).
2. C. R. Sugimoto, N. Robinson-García, D. S. Murray, et al., Nature, 550(7674), 29 (2017)
3. R. C. Blackman, A. Bruder, F. J. Burdon, P. Convey, et al., Nat Ecol Evol 4(5), 668 (2020).
4. National Academies of Sciences, Engineering, and Medicine, Accelerating Decarbonization in the United States: Technology, Policy, and Societal Dimensions (2023).
5. S. Sarkar, D. Chatterjee, N. Goswami, and P. P. Mukherjee, ACS Energy Lett 7(6), 2105 (2022).
6. B. S. Vishnugopi, E. Kazyak, J. A. Lewis, et al., ACS Energy Lett, 6(10), 37343 (2021).
7. B. S. Vishnugopi, M. T. Hasan, H. Zhou, and P. P. Mukherjee, ACS Energy Lett 8(1), 398 (2022).
8. K. G. Naik, D. Chatterjee, and P. P. Mukherjee, ACS App Mater Interfaces, 14(40), 45308 (2022).
9. B. S. Vishnugopi, K. G. Naik, H. Kawakami, et al., Adv Energy Mat, 13(8), 2203671 (2023).
10. D. Chatterjee, K. G. Naik, B. S. Vishnugopi, and P. P. Mukherjee, Adv Sci, 2307455 (2023).
Electrochemistry has become critical to enabling a sustainable future. As we build new electrochemical technologies for energy storage and conversion, we must cultivate the future workforce. Given the cutting-edge nature of these technologies, traditional university courses may not sufficiently prepare students with the skills required for careers in electrochemistry.1
To explore the connection between electrochemical education and marketable skills, the ECS Colorado School of Mines Student Chapter hosted a career path panel discussion on October 27, 2023. The event was hybrid, with panelists participating virtually, and students, professors, and other participants gathering in person. The panelists were from national laboratories, academia, and industry, and represented different electrochemical technologies (batteries, electrolyzers, electrochemical separation of radioactive materials, and electrochemical production of steel): Carrie Siu and Krista Hawthorne, Argonne National Laboratory; Lorena Grundy, Tufts University; Rachel Carter, US Naval Research Laboratory; Yuliya Preger, Sandia National Laboratories; Abhay Gupta, Tesla; Adam Maraschky, Electra; Andrew Motz, Nel Hydrogen; Darren Law, General Motors; and Dickens Law, General Motors.
Discussion ranged from different electrochemistry careers to scientific and non-scientific skills students should master for future electrochemistry jobs, as well as potential barriers to accessing an electrochemical education. Topics reflected the audience, which included undergraduate and graduate students; professors; National Renewable Energy Laboratory researchers in electrochemistry; John Newman and Andrew Herring, Colorado School of Mines ECS Fellows; and university staff from graduate programs and the career center. In the spirit of the Society, the event was open to all
interested participants.2 The chapter looks forward to organizing more events to spread awareness about electrochemistry and engage a new generation of electrochemists.
Acknowledgement: Content contributed by Zoey Huey, Melissa Popeil, Alec Howard, and Mayuri Kushare
1. C. G. Arges, Electrochem Soc Interface, 31(3), 50 (2022).
2. F. Chaves, Electrochem Soc Interface, 31(2), 39 (2022).
The ECS University of Houston (UH) Student Chapter collaborated with NAATBatt International to host NAATTBatt’s sixth annual workshop on zinc battery technology and first annual workshop on sodium battery technology. This two-day program, held November 30–December 1, 2023 at UH, explored the scientific progress and commercial potential of these technologies as emerging competitors to lithium batteries.
Dr. Claudia Neuhauser, UH Interim Vice President/Vice Chancellor for Research, extended a warm welcome and discussed the university’s commitment to build a cutting-edge battery manufacturing facility at UH, aimed at fostering user engagement and industry collaboration. Michael Sanders, Senior Advisor at Avicenne Energy, delivered a keynote speech on the future of the sodium battery market and opportunities for North American manufacturers.
The workshop’s first day featured four panel discussions that covered the commercial prospects of sodium-ion battery technology, R&D on electrodes and electrolytes, molten sodium metal batteries, and safety aspects of sodium batteries. The second day was dedicated to zinc battery technology. With more than 130 participants, the event facilitated vibrant discussions, knowledge exchange, and networking among industry professionals and researchers. It showcased the latest advances in sodium and zinc battery technology and explored their applications across a variety of chemistries.
In addition to fostering collaboration between the battery industry and academia, the chapter is committed to facilitating dialogue between national laboratories and universities. On November 10, the chapter hosted a seminar featuring Dr. Sergiy Kalnaus, Senior
Scientist at Oak Ridge National Laboratory. Dr. Kalnaus specializes in the mechanics of materials and numerical simulations of mass and charge transport to enhance battery performance and safety. Liqun Guo, student chapter president, warmly welcomed Dr. Kalnaus to UH. His captivating presentation, “The Story of Solid-State Batteries from a Mechanical Perspective,” explored challenges in batteries, delving into how mechanical properties influence electrochemical performance and safety. His expert insights offered a unique mechanical viewpoint on the field of battery technology.
These successful events reinforced the chapter’s role in bridging academia, national labs, and industry, and highlighted our commitment to advancing electrochemical technology through research, collaborative learning, and community engagement.
Acknowledgement: Content contributed by Liqun Guo.
(continued from previous page)
The ECS Technische Universität (TU) Ilmenau Student Chapter elected new officers for 2024: Nurul Amanina Binti Omar, Chair; Lukas Esper, Vice Chair; Gisella Lucero, Secretary; and Ivan Genov, Treasurer.
The chapter held its fall 2023 meeting from October 19 to 20 at TU Ilmenau. The scientific focus was on the fundamentals of X-ray crystallography methods regarding qualitative and quantitative phase analysis as well as Rietveld refinement. Esteemed Prof. Lothar Spieß, one of the authors of Modern X-ray diffraction: X-ray diffraction for materials scientists, physicists and chemists, was the presenter. Prof. Spieß has long-standing experience in teaching students on these topics. Dr. Michael Stich followed with an overview of battery research methods and common mistakes.
PhD candidates in the chapter also discussed the status of their PhD projects. Marius Grad gave a talk on the surface modification of titanium-based materials through the diffusion of carbon. Gisella Lucero presented her work on the development of electrochemical sensors based on PEDOT and hollow bimetallic nanoparticles for the detection of nitrite and phenolic compounds. Lukas Grohmann and Charan Mukundan gave updates on the combination of
electroplated and CVD layers and materials for aluminum-graphite dual-ion batteries, respectively.
This spring, the chapter hosted an in-person meeting at SCHOTT, Landshut, Germany.
The ECS Indian Institute of Technology Madras (IITM) Student Chapter organized five events in 2023. To celebrate Teacher’s Day on September 5, one of their most celebrated solid state teachers, Prof. Kanamaluru Vidyasagar, presented a lecture on structural correlations of nonmolecular solid-state energy materials. More than 60 IITM students and faculty members, and students from other Chennai region institutes, attended the event.
From September 20 to 21, in association with the SRM Institute of Science and Technology (SRMIST), the chapter held an event titled “Guest Lectures and Hands-on on Electrochemical Energy Storage.” This two-day event featured guest lectures by Prof. Jinwoo Lee and Prof. Seongbeen Kim, The Korea Advanced Institute of Science and Technology (KAIST); Prof. Sangaraju Shanmugam, Daegu Gyeongbuk Institute of Science and Technology; and Prof. Kothandaraman Ramanujam and Prof. Ramanathan Srinivasan, IITM.
The event also featured hands-on sessions focused on electrochemical energy storage covering zinc-ion batteries, fuel cells, and impedance spectroscopy. A “fastest finger first” quiz marked the workshop’s conclusion, testing participants on the topics discussed during the lectures and hands-on sessions. Winners received cash prizes.
In December, the chapter hosted the “Energy Summit and Preconference Workshop on Electro-sustainability” on the sidelines of the IITM Energy Consortium’s Energy Summit 2023 conference. Cyclone Michaung caused the event to transition to an online format at the last minute. Dr. K. Ramesha, Director of the Central
Electrochemical Research Institute (CECRI), inaugurated the event and delivered the plenary lecture covering topics that included DFT calculations, lithium-ion batteries, sodium-ion batteries, and electroorganic synthesis. More than 60 participants engaged in the online workshop.
Chapter members played a pivotal role as volunteers during the Energy Summit held from December 6 to 7. Dr. Raman Vedarajan, a chapter faculty advisor, delivered an invited talk titled “Circular Solution: Giving New Life to Polymer Electrolyte Membranes from Fuel Cells and Electrolyzers” during the conference.
On August 11, 2023, the ECS Jawaharlal Nehru University (JNU) Student Chapter and the Special Centre for Nanoscience held their fourth one-day symposium, “Sensor for Society.” Our supportive faculty advisor, Dr. Pratima Solanki, is head of the Nano-Bio Laboratory, Special Centre for Nanoscience, for biosensors and pointof-care devices. With enthusiastic efforts by President Reena Sajwan, Vice-President Archana, Secretary Amit K. Yadav, and Treasurer Jayendra Kumar Himanshu, the chapter succeeded in achieving our tremendous yet attainable vision for the organization.
The chapter has been active for a year and has been thrilled to host speakers and distinguished guest speakers, including renowned scientist Prof. Anees Ahmad Ansari, Associate Professor, King Abdullah Institute for Nanotechnology, King Saud University. Dr. Ansari’s research interests include the application of nanomaterials
in the development of electrochemical and optical biosensors and synthesis of organometallic lanthanide metal complexes. His talk, “Fluorescent Nanomaterials-based Fingermarks Detection in Forensic Science and Anti-counterfeiting” explained the significance of lathanide series elements for the use of optical sensors. Lanthanideseries-based fluorescent material has long-lived fluorescence which usually surpasses common nanomaterials such as carbon quantum dots. He also emphasized facile and low-cost synthesis of such nanomaterials. This seminar was held in the presence of esteemed faculty and Dr. Balaji Birajdar of the Special Centre for NanoScience, JNU. Prof. Bijoy Kundu congratulated Prof. Ansari. The talk concluded in an interactive brainstorming session with the audience and speakers.
The ECS University of Michigan Student Chapter commenced its inaugural semester in the fall of 2023 with a comprehensive series of events that focused on academic enrichment, discussion, and community engagement. A virtual seminar was conducted with Prof. Shannon Boettcher, followed by an in-person session featuring Dr. Steve Harris. These seminars addressed topics ranging from bipolar membranes to statistical and machine learning techniques in energy storage, along with professional career insights. The diverse subject matter facilitated a broader engagement across various academic disciplines within our student body.
Continuing the chapter’s commitment to academic exploration, the Journal Club Series provides students with a platform to lead discussion on recent academic publications. This initiative fosters critical analysis and reflection on significant advances in
electrochemistry. It also encourages peer-to-peer learning, allowing participants to gain insight into diverse topics outside their immediate expertise.
Toward the end of the semester, the chapter organized social and recruitment events at strategic locations on campus. These activities were designed to enhance collaboration and inclusivity, aiming to engage students from a variety of departments and to foster a cohesive community.
Through the spring semester, the chapter continued academic initiatives and community outreach to advance the field of electrochemistry. The chapter appreciates our members’ ongoing support and participation and looks forward to furthering our mission in the future.
In 2023, the ECS Technische Universität München Student Chapter continued its “Materials & Methods Club” speaker series with four exciting events. Experts in their fields of electrochemistry gave overviews of their current research or work in electrochemistry. These events were an ideal opportunity to learn from experts in academia and industry, helping to expand chapter members’ electrochemistry knowledge while promoting scientific exchange. Overall, the chapter events promoted scientific exchange beyond university research and reached an audience that largely exceeded the number of chapter members.
In January, Dr. Kathrin Ebner, former Coordinator (Hybrid-) Electric & Hydrogen Aviation, Bauhaus Luftfahrt e.V., spoke about the potential and challenges of hydrogen aviation. In February, Dr. Steffen Garbe, CSO at the Munich-based start-up Carbon Atlantis, presented their new patented technology for electrochemical direct air capture. In November, Dr. Christian Hagelüken, former Director for EU Government Affairs at Umicore AG & Co. KG, gave a talk on
(continued on next page)
(continued from previous page)
fuel cell and battery recycling at Umicore. Three weeks later, Prof. Fikile R. Brushett, Associate Professor of Chemical Engineering, Massachusetts Institute of Technology, delivered “Redox Flow Batteries for Grid Energy Storage: Navigating an Emerging Design Space.” Further activities in 2023 included the annual Christmas party, where we reviewed the past year, planned activities for the next year, elected new board members, and enjoyed a shared dinner.
In early 2024, the chapter hosted talks by Prof. Sergio GranadosFocil, Gustaf Carlson School of Chemistry and Biochemistry, Clark University, about novel single-ion conducting electrolytes for lithium-ion batteries; Dr. Matthias Breitwieser, CTO, ionysis, on catalyst-coated membranes for PEM fuel cell applications, and Prof. Jeff Dahn, Professor of Physics and Professor of Chemistry, Dalhousie University, on his current lithium-ion battery research.
The ECS University of the Philippines Student Chapter, based at the University of the Philippines Diliman (UPD), successfully co-organized the Energy-X 2023: Advanced Energy Storage and Conversion Technologies International Workshop and Symposium, which took place from November 27 to December 1, 2023. The event was organized by the Laboratory of Electrochemical Engineering, UPD, through Project CIPHER (Clean and Vertically Integrated Pure/Applied Hydrogen Energy Research for Next Generation Power Systems), in collaboration with the Advanced Batteries Center Philippines, The Ertl Center, and the Gwangju Institute of Science and Technology Electrochemical Reaction & Technology Laboratory.
Energy-X 2023 consisted of a three-day workshop from November 27 to 29, followed by a two-day symposium from November 30 to December 1. The three-day workshop hosted 26 local and international speakers who shared their expertise, insights, and experiences in their respective fields. Formally welcomed by Prof. Joey Ocon, the first day featured the following speakers and their respective talks: Assoc. Prof. Julie Anne Del Rosario-Paraggua, “Introduction to Electrochemical Energy Storage Technologies,”
Dr. DJ Donn Matienzo, “Numbers to Narrative: Critical Soft Skills in Research,” Prof. Richard Espiritu, “Advanced Materials for Energy Applications,” and Prof. Karl Andreas Friedrich, “Green Hydrogen Production via Water Electrolysis.” The second day featured Dr. Ryan Corpuz, “Aqueous Battery Chemistries: Redox Flow, Zn-based, etc.,” Engr. Adam John de Serra, “Pb-acid Battery Manufacturing and Development,” Dr. Maricor DivinagraciaLuzadas, “High Energy Density Batteries,” Prof. Allan Abraham Padama, “Density Functional Theory in Batteries and Fuel Cells,” and Dr. Harry Handoko Halim, “Machine Learning and DFT for Catalysis.” The third and final day featured Assoc. Prof. Karl Ezra Pilario, “Machine Learning in Energy Storage Applications,” Prof. Raymond Tan, “Process Integration in Energy and Publishing High Impact Papers,” Assoc. Prof. Po-Ya Abel Chuang, “Fuel Cell Science and Technology,” and Prof. Jhud Mikhail Aberilla, “Sustainability
in Energy Storage Technologies.” Local and international participants from academic, government, and R&D institutions around the world attended and gained valuable insights.
The workshop was followed by the symposium, which convened more than 112 participants with speakers from eight different countries. Speeches by the undersecretaries of the Department of Energy and Department of Science and Technology opened the symposium. Four keynote speakers and more than 23 invited speakers shared insights into energy storage and conversion technologies, presenting studies from their respective laboratories and industries. Keynote speakers included Prof. Karl Andreas Friedrich, Prof. Allan Abraham Padama, Prof. Tetsu Yonezawa, and Prof. Po-Ya Abel Chuang Discussions and abstracts covered research scales that ranged from atomic-scale studies utilizing density functional theory and kinetic models to cell-
(continued on next page)
(continued from previous page)
scale research, such as the design and development of electrodes, membranes, and other energy storage materials, and further extended to systems-scale research, featuring life cycle assessments of energy storage and conversion solutions. In addition to talks and discussions on the experts’ research pursuits and industrial endeavors, the Energy-X 2023 Symposium hosted a poster session where selected researchers from local and international universities presented their research. Most of the chapter members actively engaged in the poster session, presenting their research in the fields of electrochemistry and renewable energy. With experts and students from a variety of fields of expertise, the poster session served as a perfect avenue to further scientific communication and research collaborations among local and international groups. Importantly, it also served as a good platform to encourage new members to join the chapter.
Energy-X 2023 ended with “Charging in Parallel: A Scientific and Cultural XChange ” The program began with icebreakers and games that lightened participants’ moods after a long week of learning about different energy storage technologies. Best Poster Awards were given to researchers who presented their work in a comprehensive yet aesthetic manner. The gala night’s highlight was a showcase of different Philippine cultural dances performed by the Bughaw Folkloric Dance troupe. The event was graced by speeches from the University of the Philippines’ president and vice chancellor for research and development. The event was a huge success, providing an opportunity for knowledge-sharing and collaboration while highlighting the importance of various energy storage technologies and their role in sustainable development. This is the largest event the chapter has managed since its founding. We are thrilled with the turnout and engagement of attendees. Moving forward, the chapter is dedicated to planning more events of this kind.
The chapter welcomes its new Executive Committee officers: Ms. Rhoda Lyn Ramos as Chair; Engr. Glennise Faye Mejica as Vice-Chair; Engr. Alvin Mar Martin as Secretary; Ms. Luce Vida Sayson, M.Sc as Treasurer; and Engr. Mecaelah Palaganas as Director of Communications and Publicity. The chapter also recognizes its faculty advisers for their unwavering support: Prof. Joey D. Ocon; Asst. Prof. Michael T. Castro; and Asst. Prof. Goran T. Tomacruz. To stay up-to-date on chapter events and initiatives, the chapter encourages Interface readers to follow its Facebook page. The Energy-X 2023 website is at https://www.energyx2023.com/
The newly formed ECS student chapter at Texas A&M University took its inaugural steps into the world of scientific outreach, participating in the university’s Chemistry Open House. Chapter members were inspired as they captivated and educated the public about the electrifying world of electrochemistry.
Each year during National Chemistry Week, Texas A&M welcomes the community to its Chemistry Open House, a vibrant celebration of chemistry’s wonders. The event is open to everyone and aims to show how exciting and important chemistry is in our daily lives. The Chemistry Department promotes the understanding of
chemistry by proudly extending its outreach to the local community, inviting everyone to participate. Following the theme set by National Chemistry Week, “The Healing Power of Chemistry,” on October 21, 2023, the university celebrated with many activities, including lab tours, hands-on experiments, and lectures by chemistry faculty.
The newly established student chapter added an extra layer of excitement this year. Comprising students from various academic backgrounds, including chemical engineering and chemistry, they chose the fascinating topic “Electrolytes for Health and Power” to teach the public about the role of electrolytes in our bodies,
demonstrating how electrolytes are essential for life’s basic functions, from maintaining electrical neutrality in cells to generating and conducting action potentials in nerves and muscles. With a simple yet effective setup featuring a copper-zinc electrochemical cell, the students showcased the magic of electrolytes. Using commercial energy drinks and substances like Gatorade, Powerade, sugar, table salt, Pedialyte,
and more, they turned on a lightbulb to illustrate how electrolytes conduct electricity in solutions, which is vital for the normal functioning of the human body. The students didn’t stop there; diving deeper, they demonstrated real-life applications of electrochemical devices such as batteries and how electrochemistry impacts our lives in numerous ways.
The audience was a diverse mix of children, high school students, and their parents. The impact was profound, with a teacher-parent expressing keen interest in replicating the ECS Student Chapter’s experiment for her own students. This successful demonstration not only educated the public but also promoted electrochemistry to young minds. The brand-new chapter brought a spark of enthusiasm to the day filled with scientific exploration and ignited young minds with the incredible world of electrochemistry.
During the fall 2023 semester, The ECS University of Texas at Austin Student Chapter focused on growing the number of active members, continuing to provide a platform for graduate students and postdoctoral researchers to compare interdisciplinary electrochemistry research, and interacting with other ECS student chapters at other Texas universities.
Currently, with 40 active members, the chapter has doubled its membership since the beginning of the semester! To recruit more members, they engaged with other UT Austin Department of Chemistry student organizations. The chapter enjoys exposing graduate students to the power of electrochemistry to answer research questions in unexpected areas and building a sense of community for students to collaborate on research projects and make friends.
The chapter continues to host “Chalk Talks” where graduate students and postdoctoral researchers showcase research in their areas of expertise. The chapter emphasizes research methods, experimental techniques, and professional development topics rather than the broader scientific implications. This enables members of the audience to gain a fundamental understanding of an electrochemistry research area that is different from theirs. We hope this leads to interdisciplinary research collaborations. These “Chalk Talks” were hosted in the fall 2023 term: “iR Compensation: From Fundamental Principles to Practical Applications” by Dr. Yoon Jun Son; “DFT 101: Theory and Practice of DFT in Research” by Zachary H. Levell; and “Mastering the Art of Composing Scientific Graphics” by Raul A. Marquez. “Chalk Talk” recordings are on the chapter’s YouTube channel and student chapter website
The chapter is happy to announce that they have begun collaborating with ECS student chapters beyond UT Austin. This past fall, ECS student officers and members from UT Austin, UT Dallas, and Texas A&M met face-to-face for the first time to discuss plans for joint ECS activities in 2024. Stay tuned! In 2024, the chapter is committed to building a strong community of electrochemistry researchers at UT Austin and engaging with scientists in the ECS community beyond our campus!
Hook ’em Horns!
ECS University of Texas at Austin Student Chapter members explain the basics of Li-ion insertion batteries using Jenga tower sets during a UT Chemistry social event. The gray-topped Jenga tower represents the cathode; the orange-topped Jenga tower represents the anode; and the white bricks represent lithium ions which move back and forth between the anode and cathode.
Acknowledgement: Content by Jay T. Bender and Anthony C. Concepción, Chairs; Tushar Telmasre, Vice Chair; Jeremy Brinker, Treasurer; and Raul A. Marquez, Secretary.
Photos: Raul A. Marquez.
245th ECS Meeting
SAN FRANCISCO, CA
May 26-30, 2024
Marriott Marquis San Francisco
PRiME 2024
Joint International Meeting of ECS, ECSJ, & KECS
HONOLULU, HI
October 6-11, 2024
Hawaii Convention Center & Hilton Hawaiian Village
247th ECS Meeting
MONTRÉAL, CANADA
May 18-22, 2025
Palais des Congrès de Montréal
248th ECS Meeting
CHICAGO, IL
October 12-16, 2025
Hilton Chicago
www.electrochem.org/upcoming-meetings