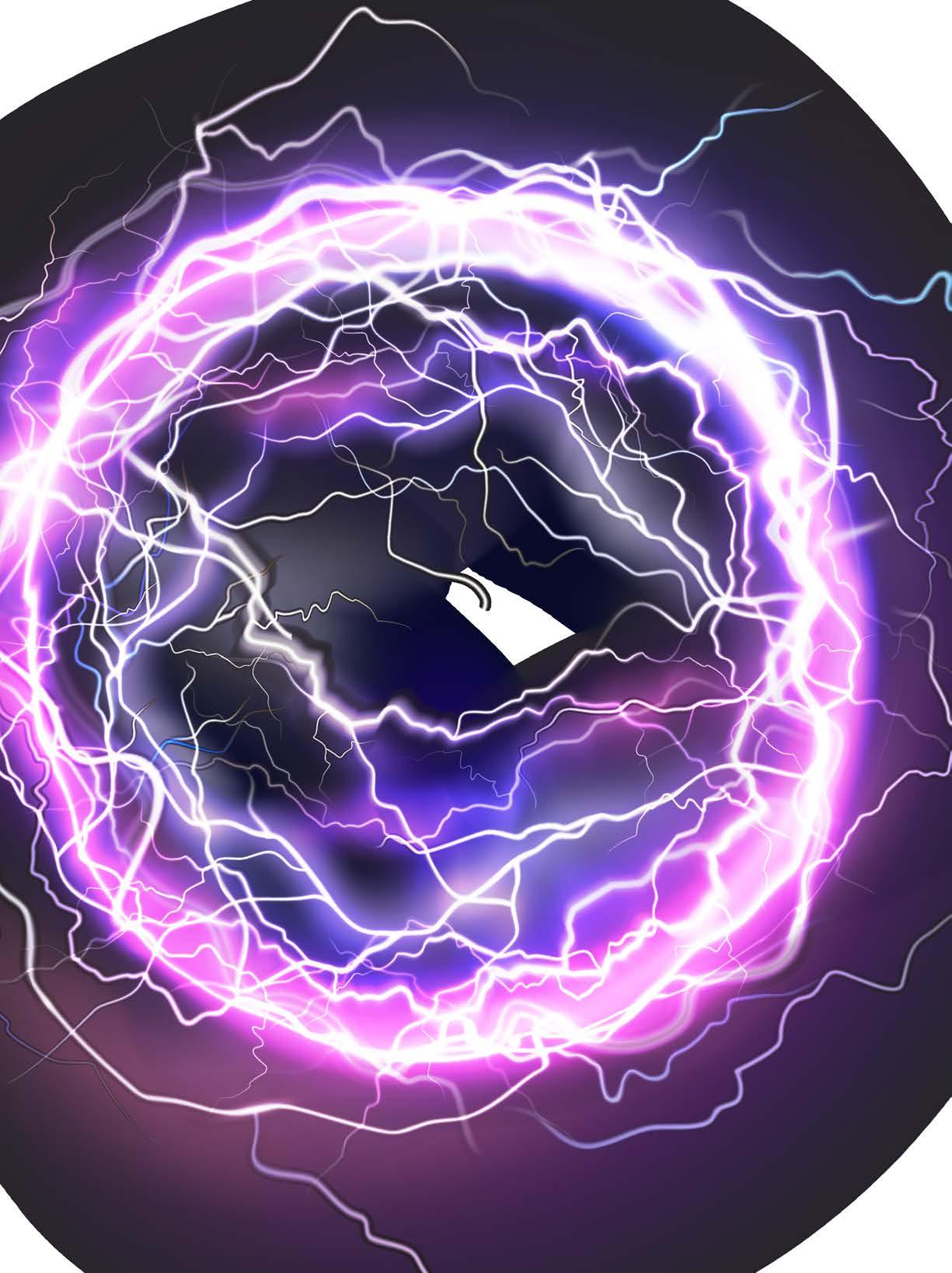
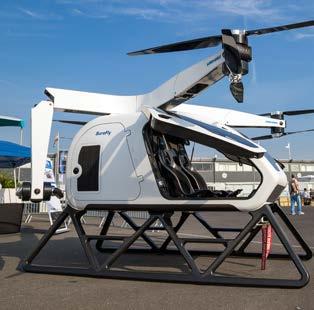
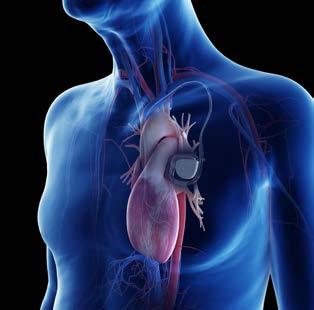
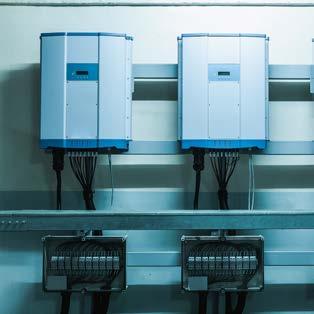
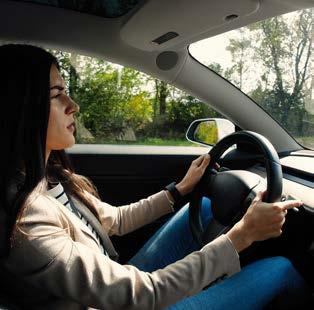
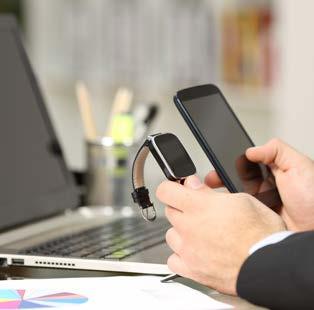
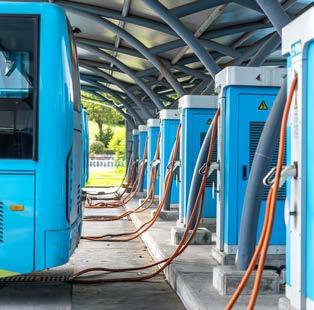
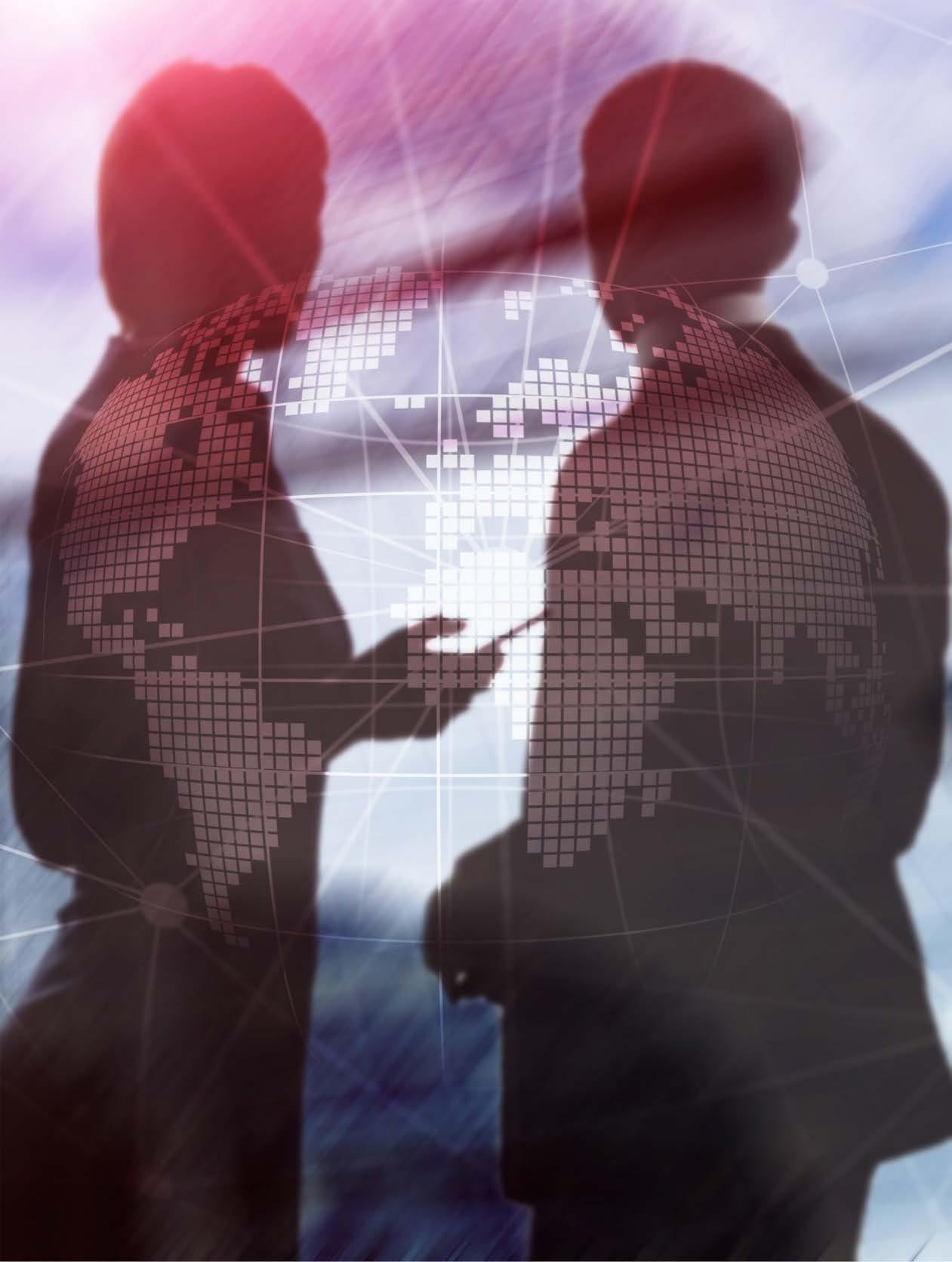
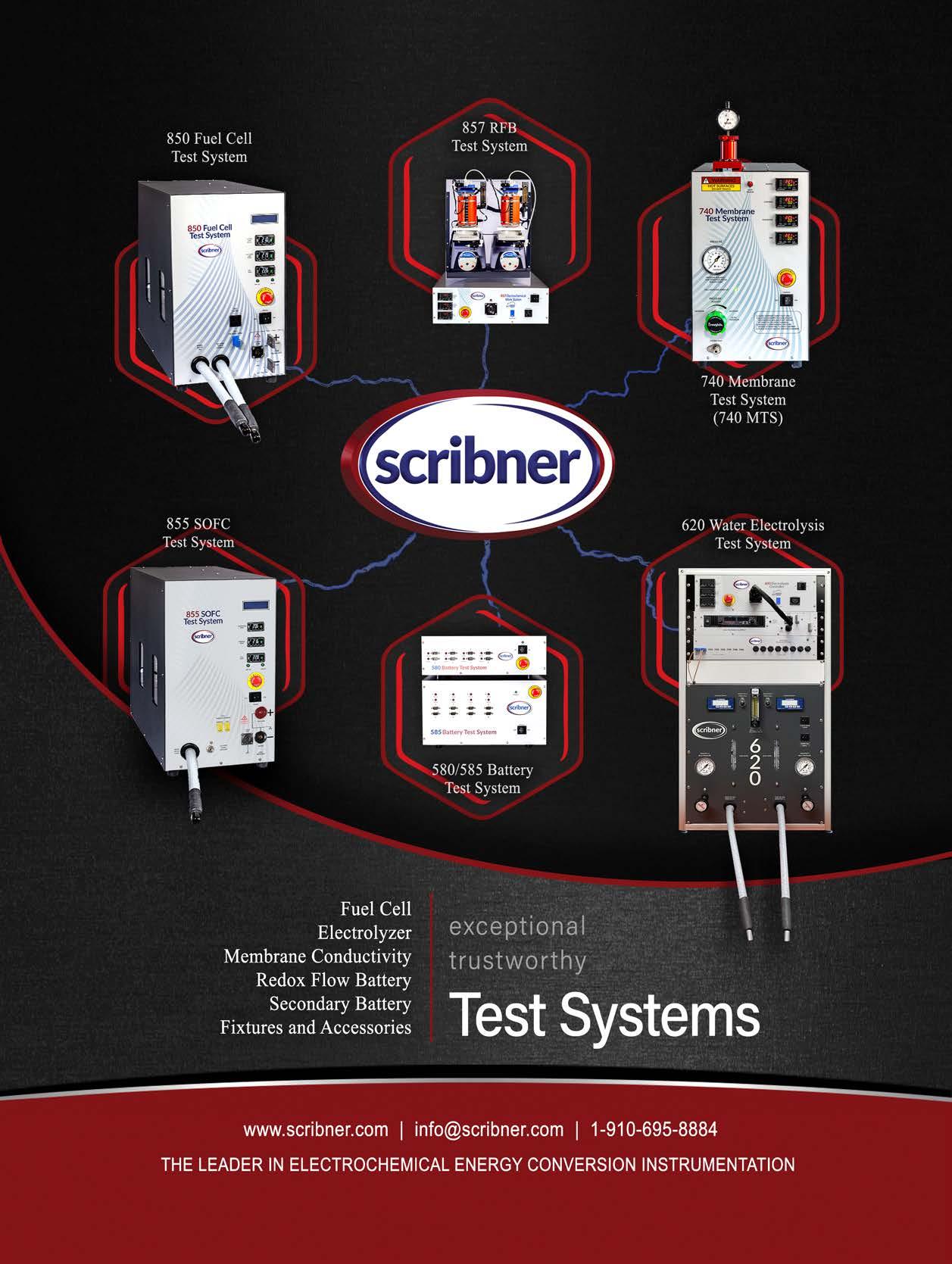
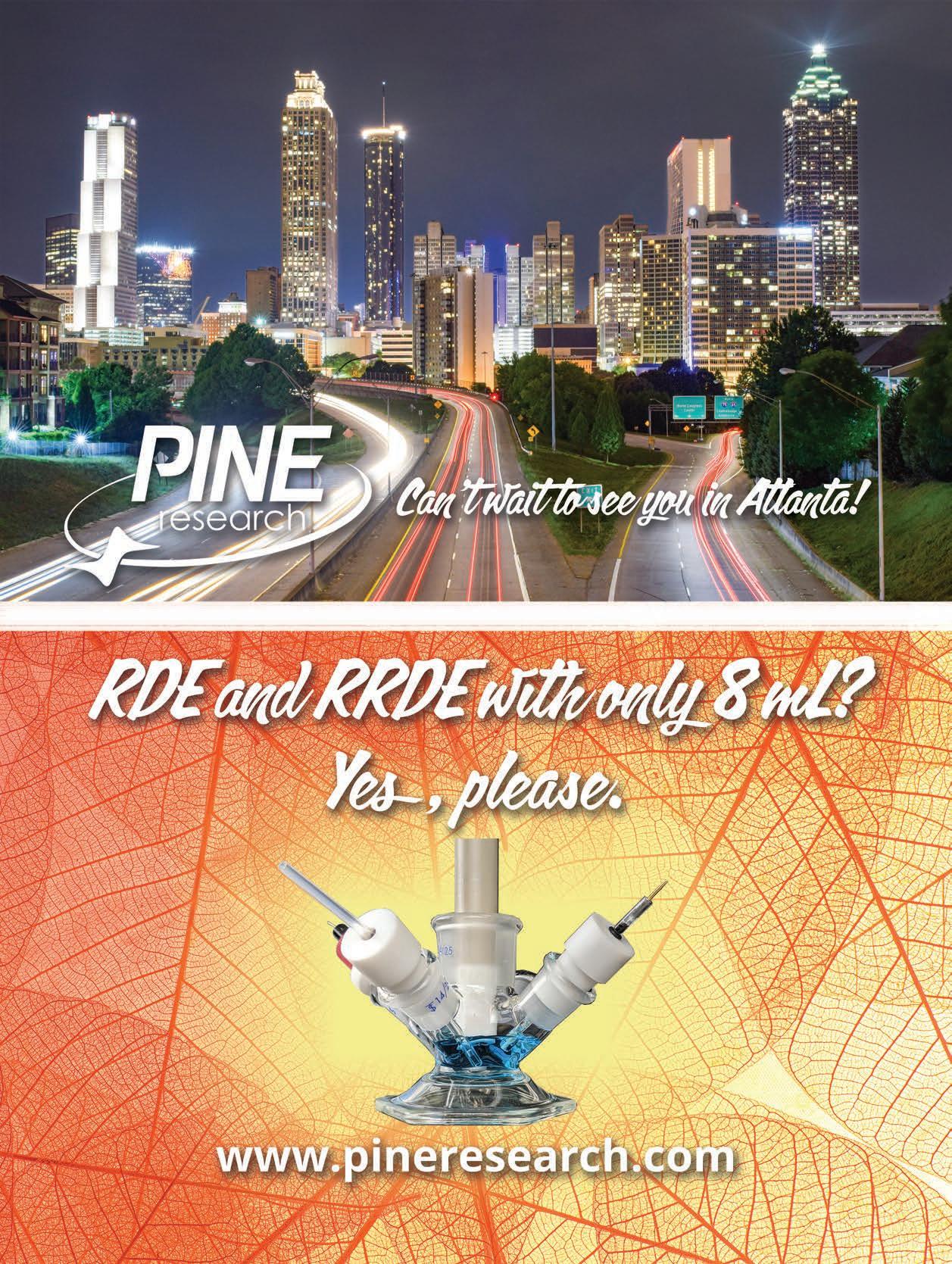
As you read this latest rant, I am preparing my talk for the Gordon Research Conference (GRC) on Aqueous Corrosion, chaired this year by the illustrious Jamie Noël of Western University (see more about him in People News). By “preparing” I mean procrastinating. Why does agreeing to give a talk seem so great at the time, only to transform into a heart-racing horror as the date approaches? There is a psychology dissertation in there somewhere. I mentioned in a previous editorial how much I enjoy the GRC although my family makes fun of my going; I guess scientists in shorts is not something you can unsee.
In explaining to my students why I enjoy GRC so much, I kept returning to how participants interact, and I realized how much that has changed since my first GRC over 35 years ago. In the 1986 conference, the first thing that struck me was the concentration of big names all in one place. It was like being at an all-star game. Even more amazing to me was the willingness of each and every one of them to (a) invite wide-eyed students to eat meals with them, and (b) sit down with students for extended research discussions. It turns out even famous people eat food and can be nice. That said, what bothered me in 1986 was that it seemed that the discussion periods after the talks were barely civil. Of course, I had seen this type of scientific discourse at conferences, but the extended Q&A did not encourage increased politeness, but rather increased rancor. It also made the vast majority of the audience tentative, at a minimum, about getting involved and asking questions.
Over the past three decades, the tone of the GRC Q&A has become more collegial without losing its rigor. Speakers are still challenged on their conclusions, speculations, and approaches, but in a more measured and balanced way. They thank and compliment the speaker for the talk, and then ask their question. Future questions often build on the exchange, and thus the ideas are given a thorough examination, just like the brochure says happens. I have learned that there are a lot of ways one can tell someone else they are full of it, but not all lead the other to honestly self-assess. When a question feels like a personal attack, the first and often only order of business is self-protection, rather than saying to oneself, “Hmm, could they be right?” Scientific progress withers when egos emerge from the watering can of discussions.
This change in behavior would appear to be counter to what has become the new norm in public discourse. People who believe what I believe are clearly intelligent and honorable, whereas those OTHERS are (fill in as many pejoratives, both adjectives and nouns, as you can). I would love to be able to say that corrosion scientists found some miracle food that lets us be nice to each other even when we disagree, but I don’t think that is it, because the favorite food group there is beer which is widely available. I think it is because the current folks who have more gray hair than any other color grew up together professionally in ways earlier generations could not. Conferences have proliferated in the past 50 years (probably too much, says crabby old man on the porch). Hundreds of interactions throughout adulthood have brought our admittedly small community closer personally. We know about the various activities of each others’ kids (and dogs, of course), the annoying parts of where we work, and the rest of the minutiae that is the foundation for human relationships. Don’t get me wrong, we still vehemently disagree on all kinds of scientific issues, but we can do so agreeably. I think that knowing the other person as a human, not just as a speaker or originator of some theory you abhor, makes that the discussion more pleasant. Anonymity does not need to be complete for it to be sufficiently appealing to our inner troll.
Before I close, in a rare example of my keeping my promises, check out the return of the Free Radicals column, “Random Connections: Spices and Interfaces,” as well as the articles by some of our new Contributing Editors. We will keep rolling them out over the next few issues. Enjoy.
Until next time, be safe and happy.
Published by: The Electrochemical Society (ECS) 65 South Main Street Pennington, NJ 08534-2839, USA Tel 609.737.1902, Fax 609.737.2743 www.electrochem.org
Editor: Rob Kelly
Contributing Editors: Christopher L. Alexander, Chris Arges, Scott Cushing, Ahmet Kusolgu, Donald Pile, Alice Suroviec
Director of Publications: Adrian Plummer Director of Community Engagement: Shannon Reed
Production Editor: Kara McArthur
Graphic Design & Print Production Manager: Dinia Agrawala
Staff Contributors: Frances Chaves, Genevieve Goldy, Mary Hojlo, Christopher J. Jannuzzi, John Lewis, Anna Olsen, Jennifer Ortiz, Beth Schademann, Francesca Spagnuolo
Advisory Board: Brett Lucht (Battery), Dev Chidambaram (Corrosion), Durga Misra (Dielectric Science and Technology), Philippe Vereecken (Electrodeposition), Jennifer Hite (Electronics and Photonics), Mani Manivannan (Energy Technology), Cortney Kreller (High-Temperature Energy, Materials, & Processes), John Weidner (Industrial Electrochemistry and Electrochemical Engineering), Jakoah Brgoch (Luminescence and Display Materials), Hiroshi Imahori (Nanocarbons), James Burgess (Organic and Biological Electrochemistry), Robbyn Anand (Physical and Analytical Electrochemistry), Ajit Khosla (Sensor)
Publications Subcommittee Chair: Colm O'Dwyer
Society Officers: Turgut Gür, President; Gerardine Botte, Senior Vice President; Colm O’Dwyer, 2nd Vice President; James (Jim) Fenton, 3rd Vice President; Marca Doeff, Secretary; Elizabeth J. Podlaha-Murphy, Treasurer; Christopher J. Jannuzzi, Executive Director & CEO
Statements and opinions given in The Electrochemical Society Interface are those of the contributors, and ECS assumes no responsibility for them.
Authorization to photocopy any article for internal or personal use beyond the fair use provisions of the Copyright Act of 1976 is granted by The Electrochemical Society to libraries and other users registered with the Copyright Clearance Center (CCC). Copying for other than internal or personal use without express permission of ECS is prohibited. The CCC Code for The Electrochemical Society Interface is 1064-8208/92.
ISSN : Print: 1064-8208 Online: 1944-8783
The Electrochemical Society Interface is published quarterly by The Electrochemical Society (ECS), at 65 South Main Street, Pennington, NJ 08534-2839 USA. Subscription to members is part of membership service. © Copyright 2022 by The Electrochemical Society. *“Save as otherwise expressly stated.”
The Electrochemical Society is an educational, nonprofit 501(c)(3) organization with more than 8,500 scientists and engineers in over 75 countries worldwide who hold individual membership. Founded in 1902, the Society has a long tradition in advancing the theory and practice of electrochemical and solid state science by dissemination of information through its publications and international meetings.
Aftertwo years and seven months, the Society has returned to hosting in-person technical conferences. The 241st ECS Meeting took place from May 29 to June 2 in beautiful Vancouver, British Columbia, Canada. How exciting it was to be back together again after such a very long time apart!
For 120 years—yes, the Society is now 120 years old— ECS meetings have been the premier technical conferences in the electrochemical and solid state fields, showcasing the visionary work of students, faculty, researchers, practitioners, current Nobel laureates, and—I think it’s a safe bet to say— future Nobel laureates as well. The Vancouver meeting carried forward ECS’s legacy of outstanding technical content, offering 45 symposia with more than 2,500 abstracts, including 1,700 oral talks, and more than 400 posters, 400 digital presentations, and 1,000 student talks. A total of 66 countries were represented. It is clear to see that with numbers like these, ECS meetings are must-attend events if your interests are in the fields of electrochemistry and solid state science.
An exclusive reception for ECS members, held just before the Opening Reception, was new this year. We hosted this exceptional event to celebrate our return to in-person gatherings after the lengthy period since our community last convened. It was certainly special, and we look forward to repeating a members-only reception at future ECS meetings.
The ECS Journals Reception was another new feature added to the conference. ECS publications have enjoyed phenomenal growth and success across all indicators (submissions, readership, revenue, etc.) in recent years. This growth, coupled with the launch of ECS Sensors Plus and ECS Advances, was more than enough reason to celebrate. The event was also an opportunity to offer our sincerest thanks to the army of volunteers and staff who have made ECS a worldclass technical publisher for over a century.
In addition to these new features, the centerpiece of the 241st ECS Meeting—like at all Society meetings—was the outstanding technical content. We thank ECS’s technical divisions and sections for this. These meetings would be impossible to produce without their support. The divisions are ECS’s technical front line, creating the communities and content that make ECS the organization that it is today. They are also, with ECS sections, a vital source of financial support for our meetings. This year, the divisions and ECS Texas and Europe Sections provided nearly $50k in travel support to more than 100 student and early-career attendees.
A generous legacy from the estate of ECS Past President Theodore R. Beck made a new travel grant administered by the IE&EE division possible this year. Dr. Beck joined the Society in 1954 and was active in local section, division, and Society affairs throughout his distinguished career. He held positions with Kaiser Permanente, Boeing, and ultimately his own research consultancy, Electrochemical Technology Corp. Dr. Beck authored 77 technical papers and received 11 patents. We are honored and humbled that Dr. Beck remembered us this way. For years to come, his gift will enable students and early-career researchers to benefit from engagement with the Society, just as he did. What a fitting tribute from one of our own! Our sincerest thanks to Dr. Beck and the Beck Family for their enduring support.
Of course, what makes ECS meetings truly special are the impromptu, serendipitous conversations and connections that happen when meeting in person. As I left the President’s Reception on Wednesday evening, I was fortunate to engage in such a conversation with an ECS board member and an ECS early-career member. I learned that, while it’s entirely appropriate to rejoice at returning to in-person meetings, we also need to be mindful that a group of members, namely students and early-career members, has been waiting patiently for many years to take part in their first in-person ECS meeting. Many have completed the majority of their PhD programs without the benefit of meeting one-on-one with colleagues and forming the personal/professional networks vital to any career. I was so grateful that this member had the opportunity, and took the time, to share their eye-opening perspective with me. As we discussed options for how to address this issue at a future ECS meeting, the board member turned to me and said, “Well, you certainly don’t get that on Zoom!”
I could not agree more, and I look forward to seeing you not on Zoom soon!
PS: Of course, meeting in person an meeting online works
Christopher Jannuzzi
Solid-state batteries are currently a hot research topic with many in the field believing solid-state is the successor techno logy of lithium-ion. Most of this research is supported or conducted by commercial entities, especially in the context of electric vehicles.
Still, with (bulk) solid-state batteries a rather recent addition to the repertoire of most academic laboratories, there are hardly any standardized equipment or procedures to reliably benchmark new materials or fabrication procedures on a lab scale. Most academic research relies on homemade setups featuring “pellet-type” cells in which the components (anode composite, solid electrolyte, cathode composite) are layered successively and compressed into a pellet/cylinder (Figure 1). Although there are well-founded doubts about the scalability of this format, it has emerged as the most simple and straightforward for batteries based on mechanically soft solid electrolytes (e.g., sulfides, halides) that can be “cold-pressed” and do not necessitate heat treatment. Off-the-shelf setups for the fabrication and testing of pellet-type cells are beginning to appear on the market (by e.g. Sphere Energy or rhd intruments) for laboratories who opt not to invest in the development of homemade ones.
The name of the game is forming and maintaining good ionic contact between the different solid materials: solid electrolyte, electrodes and possibly carbon additives 1. The materials for the anode, electrolyte, and cathode should be carefully selected for (electro)chemical compatibility. A good intermixing and packing is critical which in turn favours small grain sizes in the μm range. To ensure fast ionic percolation a 1:1 for the solid electrolyte to active material is typically used. In a composite with carbon, the order in which the materials are mixed and the type of carbon (e.g., carbon fibers, carbon black, etc.) can play a critical role. In the case of carbon-free composites, the electronic conductivity is solely provided by the electrode, so a higher loading is typically used (e.g ~1:2 in favour of active material). Many mixing methods are possible, from simple manual co-grinding in mortar & pestle, to ball-milling, to suspension in liquid etc.
PRO TIP! In the case of incompatibility between solid electrolyte and electrode, protective coatings can be applied2. Coatings are routi nely applied to batteries based on thiophosphates that are unstable against oxidation in contact with layered cathodes (e.g. LiCoO 2). LiNbO 3 coatings on such layered materials negate this issue and are recently becoming commercially available.
Once the powders have been mixed together, it is all about pres sure! At this point we refer to “fabrication” pressure in the order of 100–1000 MPa, that is typically significantly higher than “operating” or “stack” pressure. The separator layer (purely solid electrolyte) is typically formed first by applying ~100MPa to simply shape a solid basis and attach it to the mantle of the pellet die. Then, the electrode composites are added in a similar fashion. If the anode is also a composite (e.g., LTO/SE/carbon) flip the setup to form this layer on the other side of the separator base.
Figure 1: cross-section of typical pellet-type cell. Can include temperature and pressure control, typically through heated mantles and piezoelectric sensors, respectively.
Once all the powder layers are in place it’s time to fabricate the battery by pressing. The electrodes and electrolyte are typically brittle and easily fracture and form porosity and inactive surfaces.
The pressure profile is critical, in particular the maximum pressure and the pressure ramp rates on both pressing and releasing. The result after pressure release is a crack-free composite multilayer pellet-type battery! If the anode were instead a metal (e.g., Li) or alloy (e.g., Li-In) it would be applied at this stage.
PRO TIP! Alloy anodes such as Li-In and Na-Sn, for lithium and sodium batteries respectively, operate at slightly higher voltage than the respective metal and can sustain much higher currents without electrodeposition issues, making them ideal for preliminary half-cell tests.
After fabrication, pressure continues to play a critical role during cycling (“stack pressure”). Most battery electrodes (e.g. LiCoO2) will expand and contract upon lithiation and delithiation, generating localized stresses. The result can be delamination (upon shrinkage) and cracking (upon expansion), both of which create dead surfaces and reduce the ionically active area between phases in the composite electrodes (Figure 2). Similar issues of contact loss arise upon discharging metallic anodes. Applied pressure helps to alleviate these so-called “chemo-mechanical” issues3 to a certain degree.
The exact amount of pressure that should be applied is still an open question and likely dependant on the chemistry, setup, and cycling parameters (e.g rate, state of charge). Reported values range from a few MPa to a few hundred MPa. Too little pressure is not enough to maintain sufficient contact. However, too much pressure can lead to rising overpotential, short circuits, and other issues. As such, it is imperative to not only apply pres sure but also to measure, control and optimize the amount. Most setups with such capabilities rely on piezoelectric sensors or calibrated torque screws.
The most advanced setups combine measurement and control capabilities to offer on-line pressure compensation during cycling and maintain a constant stack pressure.
With the rising interest in solid state battery research, it is imperative to standardize and properly report fabrication and testing parameters for solid-state cells. Such practices will ensure the reproducibility of results among different labs and speed up the industrial adoption of research breakthroughs into practical cells.
Figure 2: schematic of composite electrode microstructure upon cycling. Contraction and expansion of the active material leads to loss of contact with the solid electrolyte matrix unless compensated with stack pressure.
[1] Bielefeld, A.; Weber, D. A.; Janek, J. Modeling Effective Ionic Conductivity and Binder Influence in Composite Cathodes for All-Solid-State Batteries. ACS Appl. Mater. Interfaces 2020 , 12 (11), 12821–12833.
[2] Culver, S. P.; Koerver, R.; Zeier, W. G.; Janek, J. On the Func tionality of Coatings for Cathode Active Materials in Thiophos phate‐Based All‐Solid‐State Batteries. Adv. Energy Mater. 2019, 1900626, 1900626.
[3] Lewis, J. A.; Tippens, J.; Cortes, F. J. Q.; McDowell, M. T. Chemo-Mechanical Challenges in Solid-State Batteries. Trends Chem. 2019, 1–14.
Written in collaboration with Dr. T. Famprikis, Delft, the Netherlands.
VANCOUVER , BC l Canada
From May 29 to June 2, 2022, the 241st ECS Meeting convened in Vancouver, with 2,192 participants from 55 countries. This was the Society’s first hybrid meeting, with 1,641 in-person attendees and 550 digital participants. The meeting encompassed 351 sessions with 45 symposia; 66 countries were represented. A total of 2,515 abstracts were accepted, including 1,702 oral presentations, 400 posters, and 413 digital presentations, including 542 invited talks and 49 ECS award and keynote talks. Students presented 1,042 abstracts, with 672 oral talks and 225 posters. The digital content included 413 digital presentations, of which 145 were digital student presentations.
Over 600 members came together to celebrate reconnecting in person again at the first-ever ECS Members Only Reception. Colleagues and new peers networked while enjoying a raffle, food, and an open bar. Five members received prize giveaways:
• Lifetime Society Membership – Dr James Noël, Western University
• $250 Amazon gift card – Prof. Sangwoo Lim, Yonsei University
• $250 Amazon gift card – Mr. Michael Spencer, North Carolina State University
• Bose Noise Cancelling Headphones 700UC – Mr. Brahim Ahammou, McMaster University
• 5-Night Hotel Stay for the 243rd ECS Meeting in Boston – Ms. Nora Chelfouh, Université de Montréal
The event was such a success that it is planned again for the next ECS meeting.
Eric described the Society’s response to the crisis in Ukraine and explained that, although not a political organization, ECS extended complimentary membership and 2022 meeting registrations to constituents there.
Christopher Jannuzzi, ECS CEO and Executive Director, made introductory remarks. He welcomed event participants in Vancouver and online to the first in-person ECS meeting in over two years. He provided an overview of the week ahead and thanked symposia sponsors and exhibitors, and meeting attendees, digital participants, volunteers, and staff. He noted in particular the growth of institutional membership by 15 percent this year. Chris remarked on the importance of the divisions’ and sections’ contributions of nearly $50,000 in travel support to over 100 student and early career attendees. He announced a new travel grant this year made possible by a bequest from former ECS President Theodore Beck (1975–1976).
Eric Wachsman, ECS President, took the floor and described the resilience of ECS membership in meeting the challenges of the pandemic and in working together to meet the global challenges faced today. He thanked former ECS Presidents Christine Bock (2019–2020) and Stefan De Gendt (2020–2021), who, along with ECS volunteers and staff, produced three digital ECS meetings to keep us connected, and science moving forward, during the COVID-19 shutdown.
Eric thanked Waters | TA Instruments for sponsoring the Plenary Session and introduced the 241st ECS Meeting Lecture by Jeff Dahn, a highlight of the ECS Plenary Session. Jeff Dahn is Professor, Canada Research Chair, and NSERC/Tesla Canada Industrial Research Chair at Dalhousie University with dual appointments in the Department of Physics & Atmospheric Science and Department of Chemistry. In his talk, “Modern Lithium-Ion Batteries: More than One Million Miles and Possibly a Century of Life,” he explained advances since 2016 that have made it possible for modern lithium-ion batteries to power a vehicle more than a million miles and possibly have a century of life. This will result in the need for vast amounts of electrical energy storage in order to put more renewables on the grid. He described using electric vehicle batteries for energy storage in socalled “vehicle to grid” applications when they are parked, in which they rack up charge-discharge cycles even when not moving. A Q&A session moderated by Marca Doeff followed his talk.
ECS President Eric Wachsman introduces the Eagle Song Dancers at the Opening Ceremony. The 241st ECS Meeting Plenary Lecture was given by Jeff Dahn of Dalhousie University. The Members Only Reception prize giveaway: (from left to right) James Noël, Eric Wachsman (who announced the winners’ names), Brahim Ahammou, Nora Chelfouh, Sangwoo Lim, Michael SpencerThe Awards and Recognition Ceremony celebrated the achievements of today’s greatest researchers in electrochemistry and solid state science. Eric explained that, as this was the first in-person ECS Awards and Recognition Ceremony in several years, award winners from previous years would be acknowledged as well as 2022 award winners.
Eric recognized the Leadership Circle Award winners and thanked these institutional members for their Gold Level 25 years of dedication to ECS: Electrosynthesis Company Inc. and Scribner Associates Inc.
ECS Second Vice President and Publications Subcommittee Chair Gerri Botte presented the Norman Hackerman Young Author Award for the best paper published in the Journal of The Electrochemical Society by a young author or co-authors in the preceding volume year. Stefan Oswald (Technische Universität München) received the 2020 award for the paper, “Novel Method for Monitoring the Electrochemical Capacitance by In Situ Impedance Spectroscopy as Indicator for Particle Cracking of Nickel Rich NCMs: Part I. Theory and Validation.”
ECS President-elect Turgut Gür recognized and thanked past division chairs:
• Marca Doeff, Battery Division (2018–2020);
• Greg Jackson, High-Temperature Energy, Materials, and Processes Division (2017–2019);
• Slava Rotkin, Nanocarbons Division (2016–2020);
• John Staser, Industrial Electrochemistry and Electrochemical Engineering Division (2018–2020).
James Fenton, Secretary (2016–2020) and Stefan De Gendt, President (2020–2021), whose terms on the ECS board had ended, were also thanked.
Katherine Ayers and Kang Xu, members elected to the 2020 Class of Fellows of The Electrochemical Society, were introduced.
Two winners of the ECS Henry B. Linford Award for Distinguished Teaching were acknowledged. The award was established in 1981 to honor excellence in teaching in subject areas of interest to the Society. Arumugam Manthiram, University of Texas at Austin, received the award in 2020 and presented his award address in a June webinar that year. Martin Winter, Institute of Physical Chemistry at the Westfälische Wilhelms-Universität Münster, received the 2022 award. In his award address, “The Role of Electrochemistry in Battery R&D,” Winter emphasized the critical importance of encouraging and motivating young scientists and students to engage in electrochemistry and in particular in electrochemical energy storage. This is of utmost
importance to generate sustainability in the battery community, which will help guarantee workforce continuity in the global challenges of climate change and depletion of fossil fuels.
Two winners of the So ciety’s Vittorio de Nora Award were acknowl edged. The award was established in 1971 to rec ognize distinguished con tributions to the field of electrochemical engineer ing and technology. Hu bert Gasteiger, Technische Universität München, re ceived the 2020 de Nora Award and presented his talk at digital PRiME 2020. Robert Savi nell, Case Western Reserve University, received the 2022 award. His address, “Electrolytes: Discovery to Fundamentals, and Fundamen tals to Discovery,” delivered later in the week, discussed different types of electrolyte systems addressed by his research over the years. He described introducing the concept of a slurry electrode for the alliron flow battery. The slurry electrode/electrolyte system facilitated the design to separate power capacity from energy capacity. He also reviewed introducing the proton conducting high temperature poly mer membrane of PBI doped with phosphoric acid.
Martin Winter received the 2020 ECS Henry B. Linford Award for Distinguished Teaching and gave his address in a Webinar in June 2020.
Winners of the 241st Z01 General Student Poster Session Awards, as well as ECS society, division, and Leadership Circle awards were recognized, including those from years when in-person meetings were not held.
These division awards were given at the meeting. All are 2022 awards unless otherwise stated.
• Dielectric Science and Technology Division Thomas Callinan Award: Stefan de Gendt, KU Leuven and IMEC
• Electronics and Photonics Division Award: Eddy Simoen, IMEC
• Electronics and Photonics Division Award (2020): Andrew Steckl, University of Cincinnati
• Energy Technology Division Graduate Student Award sponsored by BioLogic: Grace Lindquist, University of Oregon
• Energy Technology Division Graduate Student Award sponsored by BioLogic: Zachary Schiffer, California Institute of Technology
• Energy Technology Division Graduate Student Award sponsored by BioLogic (2020): Lisa Housel, Stony Brook University
• Energy Technology Division Research Award: Vito Di Noto, Università degli Studi di Padova
• Energy Technology Division Research Award (2020): Paul Kenis, University of Illinois at Urbana-Champaign
• Energy Technology Division Supramaniam Srinivasan Young Investigator Award: James L. Young, National Renewable Energy Laboratory
• Energy Technology Division Supramaniam Srinivasan Young Investigator Award (2021): Iryna Zenyuk, University of California, Irvine
• Energy Technology Division Supramaniam Srinivasan Young Investigator Award (2020): Christopher Hahn, University of California, Berkeley
Arumugam Manthiram, recipient of the 2022 ECS Henry B. Linford Award for Distinguished Teaching, with ECS CEO Chris Jannuzzi(continued from previous page)
• Industrial Electrochemistry and Electrochemical Engineering Division Student Achievement Award: Matthew Liu, Stanford University
• Industrial Electrochemistry and Electrochemical Engineering Division New Electrochemical Technology (NET) Award (2021): Urban Electric Power
• Nanocarbons Division Richard E. Smalley Research Award: Prashant V. Kamat, University of Notre Dame
• Nanocarbons Division SES Young Investigator Award: Ardemis Boghossian, École Polytechnique Fédérale de Lausanne
• Organic and Biological Electrochemistry Division Manuel M. Baizer Award: R. Daniel (Dan) Little, University of California, Santa Barbara
• Organic and Biological Electrochemistry Division Manuel M. Baizer Award: Kazuhiro Chiba, Tokyo University of Agriculture and Technology
• Physical and Analytical Electrochemistry Division David C. Grahame Award (2021): Bruce Parkinson, University of Wyoming
The Z01 General Student Poster Session presented 92 posters. The session’s award winners are listed here (only corresponding authors are included).
• 1st Place: $1,500 cash award: Raunaq Bagchi, University of Toronto
“Effect of Surface Chemistry and Morphology on PolyluminolCarbon Redox Active Composites”
• 2nd Place: $1,000 cash award shared by:
» Lena Viviane Buehre, Leibniz Universität Hannover
» Alexander McLeod, University of British Columbia “Electrolysis Reference Electrode Methodology and Electrochemical Modeling Applications”
The following members served as judges for the 241st ECS Meeting Z01 General Student Poster Session.
• Alice Suroviec, Berry College, Z01 Lead Symposium Organizer
• Stefan De Gendt, IMEC
• David Hall, University of Cambridge
• Hemanth Jagannathan, IBM Corporation Research Center
• Bernardine Rinkel, University of Cambridge
Chris Jannuzzi opened the meeting with preliminary remarks and thanked the meeting sponsors:
• Waters | TA Instruments for the Plenary Session;
• Arbin Instruments for the meeting lanyards;
• Pine Research Instrumentation for the Student Mixer;
• Physics World for the exhibitor lounge;
• Department of Materials Science and Engineering at National Tsing Hua University and E-One Moli Energy Canada for the afternoon coffee breaks.
President Eric Wachsman thanked all the past presidents and especially Stefan De Gendt for shepherding the Society during the pandemic. He said that 2021 was a remarkable year for ECS in many
critical ways. The Society minimized the financial impact of COVID, found ways to convene and connect our community, dealt with remote operations, launched new initiatives vital to our future success, and significantly increased the Society’s net assets. The Society also prepared the launch of two new Gold Open Access publications, ECS Advances and ECS Sensors Plus. After lunch, the minutes from the June 2021 business meeting were approved. Secretary Marca Doeff introduced the new 2022–2023 officers: President Turgut Gür, Stanford University; 3rd Vice President Jim Fenton, University of Central Florida; and Treasurer Elizabeth Podlaha-Murphy, Clarkson University.
Marca described the Society’s pivot to digital meetings, welcoming more than 7,700 meeting attendees to the two 2021 virtual biannual meetings. Among the 120 symposia, the Society received over 4,000 abstracts from 70+ countries. The symposia funding program awarded over $101,000 in speaker support. ECS also sponsored other conferences, notably SOFC XVII which was attended by nearly 400 attendees from 30 countries. The Society looks forward to the 242nd ECS Meeting in Atlanta; the 243rd ECS Meeting in conjunction with SOFC XVIII in Boston; and the 244th ECS Meeting in Gothenburg.
ECS membership dropped from 8,000+ to roughly 6,900 individual members due to the pandemic and not having in-person meetings. However, over one-third of ECS members are now students and early-career researchers. Student chapters have grown to 113 around the world.
ECS’s support of research included:
• Five ECS Toyota Young Investigator Fellowships, totaling $250,000;
• Four ECS Summer Fellowships, totaling $20,000;
• Divisions awarded over $19,235 in biannual meeting travel grants.
Significant progress was made by ECS journals, which received over 4,547 manuscript submissions, up 23 percent from 2020. Just over 2,000 journal articles were published and nearly 7 million articles and abstracts were downloaded in 2021—more than a 62 percent increase over 2020. Interface celebrated its 30th anniversary. The digitization project is complete and all Interface editions are now in the ECS Digital Library on IOP Science. ECS Transactions published its 100th volume, “IV Congreso Colombiano de Electroquímica.” The 4th Edition of Electrochemical Systems by John Newman and Nitash P. Balsara was published, the first title released under a revised book program launched by the Society and Wiley Publishing, building on our longstanding joint imprint. There were 642 open access papers published in 2021 and 31 percent of all content downloaded last year was open access.
Gessie Brisard delivered the treasurer’s report, citing revenues of $8.3M in 2021, including investment and real estate income. Program services expenses were about 35 percent under budget. Net assets increased by about $3M. Interest, dividends, and realized gains on investments also yielded gains.
Incoming ECS President Turgut Gür was introduced. He thanked Eric for his service, and the extraordinary efforts and contributions of the ECS staff and its leadership team. Turgut outlined areas where members have much to offer in effecting solutions to global challenges and pursuing exciting new directions. Turgut noted that ECS has been his professional home since 1973. Then the meeting adjourned.
More than 600 students and early-career professionals mingled in a relaxed setting and enjoyed light hors d’oeuvres and refreshments at the Student Mixer. Pine Research Instrumentation, Inc. sponsored the event.
More than 600 students and early-career professionals attended the Student Mixer.
Our gratitude to the meeting symposia sponsors and exhibitors, whose support and participation directly contributed to the success of the meeting. Thank you for developing the tools and equipment driving scientific advancement, sharing your innovations with the electrochemical and solid state communities, and providing generous support for the 241st ECS Meeting.
Meeting exhibitors displayed their products and services in the Exhibit Hall and Digital Exhibitor and Vendor Guide (DEVguide). Viewers can scan the latest electrochemistry and solid state science products with ease as the DEVguide navigates seamlessly on any platform.
Platinum Waters | TA Instruments Gold Physics World
Silver Arbin Instruments Pine Research Instrumentation Bronze Molicel Department of Materials Science and Engineering, National Tsing Hua University
Silver NAURA-Akrion, Inc.
Bronze Department of Industrial Engineering of the Università degli Studi di Padova Hydro-Québec ProSys, Inc.
Contributing Comet, Inc. Mattson Technology, Inc. Yield Engineering Systems, Inc.
Admiral Instruments Arbin Instruments attocube systems AG
BASi
BioLogic USA
Cougar Creek Technologies, LLC
De Nora easyXAFS
El-Cell GmbH
The Electrochemical Society Gamry Instruments
Harvard Bioscience IOP Publishing IVIUM
Maccor, Inc
Metrohm USA
PalmSens BV Systems
Pine Research Instrumentation
Royal Society of Chemistry
Scribner
Spectro Inlets ApS
JES is the flagship journal of The Electrochemical Society. Published continuously from 1902 to the present, JES remains one of the most highly cited journals in electrochemistry and solid state science and technology. of The Electrochemical Society
ECS Sensors Plus is a one-stop shop journal for sensors. This multidisciplinary, Gold Open Access journal provides an international platform for publishing high-quality impactful and promoting scholarly communication and among scientists, engineers, and technologists primary interests and a multidisciplinary, Gold Open Access Advances communication-
With more than half of 2022 behind us, and the balance of the year shrinking at what seemings to be an accelerated rate, the successes of the ECS Publications portfolio continue. Not only do our readership and impact continue to increase, but we are meeting these
We launched the ECS Publications webinar series to increase our engagement with and support of the author community. If you missed the first two, “Diversity in Publishing Science” and “How to Boost Citations: Tips for Researchers,” be sure to check them out. They can be watched via the ECS webinar series archive. We have more webinars planned for 2022, so stay tuned!
As of June, in 2022 ECS has published more than 1,200 peer reviewed journal articles, 1,800+ proceedings manuscripts, more than 2,500 meeting abstracts, two full issues of Interface magazine, and has launched two new gold open access journals, ECS Sensors Plus and ECS Advances. We continue to see growth in readership and impact, with downloads of our content surpassing 4.16 million and the Clarivate Journal Citation Report Impact Factor increasing for both JES and JSS, to 4.386 and 2.483 respectively—a 22% increase in aggregate! The growth and effort continue to be a testament to the commitment and dedication of the ECS volunteers and staff, and the amazing and impactful work of the electrochemical and solid state scientific community.
With the 241st ECS Meeting in Vancouver being the first in-person ECS meeting in over two years, we were excited to host two ECS Publications events onsite at the meeting. One was the ECS Meet the Editors, where editors of our publications team scheduled time at the ECS exhibit booth to meet and network with the community and answer questions about the journals and the ECS editorial process. The second was the Joint Journal Reception, an invitation-only event, where the ECS leadership celebrated editors, editorial advisory members, and reviewers of ECS journals for their commitment and hard work which is essential to maintaining ECS’s reputation as a world class publisher of scientific content.
The ECS Publications family continues to forward the ECS mission and is reaching new heights!
milestones while remaining rooted in our commitment to the ECS communication pillars of Connection, Empowerment, Acceleration, Engagement, and Championship.
Brett Lucht – At the May 2, 2022 ECS Publications Subcommittee Meeting, as recommended by the Editor-in-Chief and Technical Editor, the committee approved renewing Dr. Lucht’s term as Associate Editor for the Batteries and Energy Storage topical interest area. His threeyear term ends on June 30, 2025.
Charles Hussey – As recommended by the Editor-in-Chief and Technical Editor, the ECS Publications Subcommittee, at their May 2 meeting, approved the renewal of Dr. Hussey’s term as Associate Editor for the JES Electrochemical/Electroless Deposition topical interest area. His three-year term ends on December 31, 2025.
Rohan Akolkar – During their May 2 meeting, the ECS Publications Subcommittee, at the recommendation of the Editor-in-Chief and Technical Editor, approved the renewal of Dr. Rohan Akolkar’s term as JES Associate Editor for the Electrochemical/Electroless Deposition topical interest area. His three-year term ends on August 31, 2025.
David Cliffel – On June 1, the ECS Technical Affairs Committee, at the recommendation of the ECS Publications Subcommittee, voted to reappoint Dr. Cliffel as Technical Editor of the Physical and Analytical Electrochemistry, Electrocatalysis, and Photoelectrochemistry topical interest area for JES. He will serve the journal for a three-year term, until August 14, 2025.
Betar M. Gallant, Yayuan Liu, Jacob S. Spendelow, and William Tarpeh received the 2022–2023 ECS Toyota Young Investigator Fellowships for projects in green energy technology. The fellowship program is a partnership between The Electrochemical Society and the Toyota Research Institute of North America (TRINA), a division of Toyota Motor Engineering & Manufacturing North America,
Inc. (TEMA). Through this program, ECS and Toyota promote innovative and unconventional green energy technologies born from electrochemical research—and encourage young professionals and scholars to pursue battery and fuel cell research. This is the eighth year that the fellowships have been awarded. Since its inception, the program has awarded more than $1.4M in research funding to 28 young investigators (including the 2022–2023 recipients).
Betar M. GallantMassachusetts Institute of Technology
“Correlating Organic Phase Partitioning and Coulombic Efficiency in the Li Solid Electrolyte Interphase”
Betar Gallant is Associate Professor in the Department of Mechanical Engineering and ABS Career Development Chair at the Massachusetts Institute of Technology (MIT). Her pioneering research methodologies illuminate fundamental insights into chemical dynamics and material properties at the solid electrolyte interphase of lithium and beyond-lithium metal anodes.
Prof. Gallant completed her SB (2008) and SM (2010) in Mechanical Engineering at MIT. Over the summer of 2009, she served as Energy Technology Program Specialist at the US Department of Energy, co-leading the development of the Presidential Initiative RE-ENERGYSE (Regaining our Energy Science and Engineering Edge) to strengthen energy and STEM education in public schools. Prof. Gallant returned to MIT to complete her PhD (2013) with Yang Shao-Horn as advisor. Her postdoctoral research at the California Institute of Technology from 2013 to 2015 was co-advised by Nathan S. Lewis and Julia R. Greer. In 2016, she joined MIT as Assistant Professor in the Department of Mechanical Engineering and held the Esther and Harold E. Edgerton Career Development Chair until 2021, when she assumed her current position. Prof. Gallant has received awards that include the 2021 ECS Battery Division Early Career Award; 2021 NSF CAREER Award; 2020–2021 Scialog Fellow in Negative Emissions Science and 2019 in Advanced Energy Storage; 2019 Ruth and Joel Spira Award for Distinguished Teaching at MIT; 2019 Army Research Office Young Investigator Award; and 2016 MIT Bose Research Fellowship. The author of 50 articles (h-index 22), Prof. Gallant holds one patent with six applications pending. She is on the Early Career Advisory Board of Batteries & Supercaps and is faculty advisor for the ECS MIT Student Chapter.
Yayuan Liu
Johns Hopkins University
“Redox-Tunable Lewis Bases for Electrochemical Carbon Capture”
Yayuan Liu is Assistant Professor in the Department of Chemical and Biomolecular Engineering and an associate faculty member in the Department of Materials Science and Engineering at Johns Hopkins University. Her research group works on developing efficient, economical, and sustainable carbon capture technologies critically needed to tackle climate change. Her ECS Toyota Young Investigator Fellowship research focuses on developing redox-active Lewis
base sorbents whose CO2 affinity can be modulated by applying electrochemical potentials to enable modular carbon capture systems using renewable electricity.
Prof. Liu earned her BS in Materials Science and Engineering in 2014 from Nanyang Technological University and received the Lee Kuan Yew Gold Medal for academic achievement. She completed a PhD in 2019 at Stanford University under the guidance of Prof. Yi Cui in the Department of Materials Science and Engineering, supported by the Stanford Graduate Fellowship. Prof. Liu completed her postdoctoral training at the Massachusetts Institute of Technology, working with Prof. T. Alan Hatton in the Department of Chemical Engineering. Her research has garnered multiple awards, including the American Chemical Society (ACS) Division of Inorganic Chemistry Young Investigator Award and Materials Research Society (MRS) Graduate Student Gold Award; and she was a Forbes “30 Under 30” science honoree. The author of 70 articles (h-index 57), she holds three patents. She serves as Associate Editor for Materials Today Energy
Jacob Spendelow is a Scientist at the Los Alamos National Laboratory (LANL). His research focuses on fundamental and applied electrochemical science associated with energy devices such as fuel cells and electrolyzers. This work has included analysis of electrocatalytic processes on model surfaces, development of novel catalysts and catalyst supports for oxygen reduction and oxygen evolution reactions, and design of improved electrode structures and transport media for fuel cells and electrolyzers.
Dr. Spendelow received a BS (2002) and MS (2003) in Chemical Engineering from Case Western Reserve University. He completed a PhD at the University of Illinois at Urbana-Champaign in 2006 with funding from a National Science Foundation Graduate Research Fellowship. Andrzej Wieckowski and Paul Kenis were his advisors. He joined LANL as a Director’s Postdoctoral Fellow in 2006 and became a staff member in 2008, working with the fuel cells team. In addition to his research work, Dr. Spendelow served for several years as a science advisor and program manager at the Department of Energy Fuel Cell Technologies Office, where he managed R&D projects related to polymer electrolyte membranes, catalysts and supports, and reversible fuel cells. He is the recipient of the 2019 Hydrogen and Fuel Cell Technologies Office Annual Merit Review Fuel Cell R&D Award. The co-author of more than 40 technical publications (h-index 30) and one book chapter, he has two patent applications pending. Dr. Spendelow is a member of the US DRIVE Fuel Cell Technical Team. ECS Toyota Young Investigator Fellows
William Tarpeh is Assistant Professor of Chemical Engineering at Stanford University. The Tarpeh Lab develops and evaluates novel approaches to resource recovery from “waste” waters at several synergistic scales: molecular mechanisms of chemical transport and transformation; novel unit processes that increase resource efficiency; and systems-level assessments that identify optimization opportunities. His ECS Toyota Young Investigator project focuses on designing solute-selective membranes for lithium and other critical battery materials (e.g., nickel, cobalt).
Dr. Tarpeh completed his BS in Chemical Engineering at Stanford in 2018. His MS (2013) and PhD (2017) in Environmental Engineering at the University of California, Berkeley were supported by an NSF Graduate Research Fellowship, Ford Foundation Predoctoral Fellowship, and UC Berkeley Chancellor’s Fellowship. Kara Nelson supervised his PhD research. From 2017 to 2018, he conducted postdoctoral training at the University of Michigan in environmental engineering. He joined the faculty at Stanford in 2018. His research has garnered awards that include the 2022 Dreyfus Foundation Teacher-Scholar Award. He is a member of the Bouchet Honor Society, and was included in the 2019 C&EN Talented 12; 2019 Environmental Science & Technology Letters Early Career Scientists list; 2019 Forbes “30 Under 30” science list; and 2016 NBCBLK “28 Under 28” African-American Innovators. He is the author of 26 articles and three patents.
semiannually to present their research progress. Their findings are published open access in a relevant ECS journal, and within 24 months of the end of the research period, presented at an ECS meeting. Toyota may choose to enter into a research agreement to continue working with the Fellows after their fellowships end. Toyota Young Investigators Fellowships
ECS gratefully acknowledges 2022–2023
• Timothy (Tim) Arthur, Principal Scientist, Materials Research Department
• Hongfei Jia, Senior Manager, Research, Lab M3
• Rana Mohtadi, Principal Scientist, TRINA
• Charles (Chip) Roberts, Principal Scientist, Toyota Motor North America R&D
• Ryuta Sugiura, Director, Material Research, Toyota North America
• Elizabeth Biddinger The City College of
• John T.
• Gang Wu of
The request for proposals for next November 2022. The January 31, 2023.
Candidate interviews take place in February and March of 2023. The Fellows’ names are announced in July/August 2023. Consult the ECS website for more information and to submit a proposal.
“Enabling and Implementing a Sustainable Circular Economy Using Selective Membranes”
Mohammadreza Nazemi, PhD, received The Electrochemical Society’s prestigious 2022 ECS Colin Garfield Fink Fellowship to support his research project, “Electrochemical Redox Processes for Wastewater Treatment and Resource Recovery Using Single Atom Catalysts.” The fellowship provides research support for the months of June through August.
Nazemi is currently a Postdoctoral Associate in the Department of Chemical and Environmental Engineering at Yale University. His work focuses on developing an electrochemical system composed of single–atom metal catalysts to degrade a wide range of organic pollutants and recover valuable resources (nitrogen-based compounds) from complex wastewater matrices. He said, “I am very excited to receive this fellowship and so grateful to ECS for continuing to support members’ professional growth. The fellowship enables me to lead and explore a new research topic, which is invaluable experience in project management, budgeting, and timely deliverables for my future independent career.”
Dr. Nazemi completed his PhD at the Georgia Institute of Technology in May 2020 with Professor Mostafa A. El-Sayed
At Georgia Tech, he worked as a Graduate Research Assistant
Applications for the 2023 ECS Colin Garfield Fink Fellowship open on September 15, 2022, and are due by January 15, 2023. Candidates for the $5,000 award are postdoctoral scientists or engineers and ECS members. Recipients submit a brief resume or abstract of their fellowship
in the Woodruff School of Mechanical Engineering (August 2015–May 2020), then as a Postdoctoral Fellow in Chemistry and Biochemistry (June 2020–August 2021). He then joined Professor Jaehong Kim’s research group at Yale.
Technology co-invented by Dr. Nazemi was recently licensed by Hydrofuel Inc. to complete a commercial application and demonstration of the micro-ammonia production system (MAPS). This electrochemistry-based technology produces pure ammonia from atmospheric nitrogen, water, and renewable electricity.
Notable awards Dr. Nazemi has received include the 2021 American Chemical Society Physical Chemistry Division Young Investigator Award and the 2018 Amazon Catalyst at ECS award. At Georgia Tech in 2020, he was the Technology Innovation: Generating Economic Results Class of 2020 Fellow, and received the Georgia Research Alliance Award and Georgia Tech-Oak Ridge National Lab Seed Grant Award. Dr. Nazemi is first author of 12 articles (h-index 10). CRC Press published his book, Photo-electrochemical Ammonia Synthesis: Nanocatalyst Discovery, Reactor Design, and Advanced Spectroscopy, in 2022. An active member of ECS, Dr. Nazemi has served as student member of the Society’s Individual Membership Committee and officer of the ECS Georgia Tech Student Chapter (2017–2020).
research at the end of the award period, for publication in The Electrochemical Society Interface.
Learn more at www.electrochem.org/fink-fellowship
The following students received 2022 ECS Summer Fellowships, which support students from June through August pursuing work of interest to the Society. To qualify, the recipients must be ECS members enrolled in a college or university. At the end of the award period, recipients submit a report on their fellowship project for publication in The Electrochemical Society Interface
Maha Yusuf received the 2022 Edward G. Weston Fellowship to pursue her project “Operando and In-Situ Characterization of FastCharging Degradation Modes in Li-Ion Batteries Using High-Resolution Neutron Imaging.” She is a PhD candidate in Chemical Engineering at Stanford University with support from a Schlumberger Faculty for the Future Award (2018–2023) and Stanford DARE (Diversifying Academia Recruiting Excellence) Fellowship (2020–2022). Prof. Michael F. Toney and Dr. Johanna L. Nelson Weker at SLAC National Accelerator Laboratory advise her research, which uses imaging diagnostic tools to understand the failure mechanisms of lithium-ion batteries during extreme fast charging. Maha has won awards that include the 2022 American Chemical Society CAS Future Leader; 2020 Stanford Distinguished Student Energy Lecturer; and 2020 and 2021 ECS and American Institute of Chemical Engineers Travel Grants. She holds an MS in Chemical Engineering from Stanford (2017) and BE from the National
University of Sciences and Technology, Pakistan (2013). Prior to attending Stanford, Maha worked on oil and gas rigs as a drilling engineer in Colombia. She has published six articles (h-index 2) and holds two patents.
Qiming Liu received the 2022 Joseph W. Richards Fellowship for “Functional Composites for High-Performance Electrocatalysis.” He is currently pursuing a PhD in Chemistry at the University of California, Santa Cruz, under the supervision of Dr. Shaowei Chen. His research focuses on single-atom catalysts toward energy conversion, ligand functionalization of nanoparticles, and ultrafast synthesis of electrocatalysts. Qiming has also received the 2022 International Precious Metals Institute Student Award and a 2021 Sigma Xi Grant in Aid of Research. He has published 27 articles (h-index 9) and has one patent pending. Qiming received his BE in Materials Chemistry in 2018 from Central South University, China.
Sudhan Nagarajan’s 2022 F. M. Becket Fellowship project is “Understanding Cathode Electrolyte Interphase (CEI) Chemistry on the Li-Ion Battery Cathodes Operated at Extreme Environment.” He is a PhD student in the Department of Mechanical Engineering at Wayne State University advised by Prof. Leela Arava. Sudhan’s current research focuses on understanding the structure-property relationships of potential cathode materials using advanced synchrotron spectroscopy probes and microscopy investigation for next-generation alkali metal ion and metal batteries. Previously at Clarkson University, he was actively involved in research for high energy and high power beyond Li-ion battery chemistries. Sudhan is an active user of the Brookhaven, Argonne, and Berkeley National Laboratories’ scientific facilities. His work has been acknowledged with the 2021 Wayne State University Thomas Rumble Scholar Award; participation in the 23rd National School on Neutron and X-Ray Scattering, Argonne and Oak Ridge National Laboratories (July 2021); and the 2017 FAER McAfee Scholar Award. The author of 11 articles (h-index 7), he completed a Bachelor of Technology at the Central Electrochemical Research Institute, Tamil Nadu in 2017.
Rui Jia will research “Electrochemical ResistivePulse Sensing of Extracellular Vesicles” with support from the 2022 H. H. Uhlig Fellowship. Her goal is to develop an electrochemical resistive-pulse (ERP) sensing technique to directly detect single extracellular vesicles released from a specific cell and analyze reactive oxygen and nitrogen species (ROS/RNS) in the vesicles. She is pursuing a PhD at the CUNY Graduate Center with Dr. Michael V. Mirkin as advisor. Her experiment will take place in the Department of Chemistry and Biochemistry at Queens College – CUNY. Rui’s research interests include the development of novel nanosensors, electrochemical analysis, and bio-electrochemistry. She has received the CUNY 2022 Rose K. Rose Dissertation Award, has published nine articles (h-index 6), and holds one patent. Rui completed a BS at Nanjing University in 2016.
2023 ECS Summer Fellowship applications open on September 15, 2022, and are due by January 15, 2023. Learn more at https:// www.electrochem.org/summer-fellowships.
The Electrochemical Society and NAATBatt International (NAATBatt) are coordinating an effort to develop educational resources to address the battery industry’s critical workforce training needs. Since its inception in 1902, ECS has led the world in electrochemistry, solid state science and technology, and allied subjects. In particular, ECS members include internationally recognized luminaries in battery research, from the Nobel Prize–winning inventors of the lithium-ion battery, to the highest-cited authors in “beyond lithium-ion” research. NAATBatt is a not-for-profit trade association of companies, associations, and research institutions devoted to promoting the commercial interests of its members by supporting developments in the science of, and markets for, advanced electrochemical energy storage technology.
Through its Education Committee for Clean Energy Storage, NAATBatt recently conducted a survey to better understand the existing skills gaps in the battery industry and to identify future needs for skilled workers in the battery industry across manufacturing, applications, and ancillary industries. The survey results will guide
activities, policies, incentives, and tools for workforce development and training for the battery industry in various regions of the United States. The survey was sent to the NAATBatt membership and yielded many important results. The vast majority of respondents (90%) found that there were a limited number of applicants with required skills for recent postings, and roughly half (44%) were not able to find local applicants with the skills they needed.
With their aligned technical scopes and complementary memberships, ECS and NAATBatt are uniquely poised to address the identified workforce development gaps. NAATBatt, with its focus on commercialization and manufacturing, brings industry perspective and understanding of workforce development needs. ECS, heavily steeped in academics and research, represents the global community of scientists, engineers, and educators to develop and deliver targeted, industry-specific curricula to address these identified workforce development needs.
The initial rollout of courses is actively being defined by a taskforce composed of ECS and NAATBatt volunteers and ECS staff. This is the next critical step in a long development and launch process. Check the News section of the ECS website and the next edition of Interface to stay abreast of this exciting effort.
The Electrochemical Society was privileged to take part in the symposium and celebration commemorating John Goodenough on the momentous occasion of his 100th birthday on July 25, 2022. The University of Texas at Austin Cockrell School of Engineering, where Prof. Goodenough continues to engage in his groundbreaking research, hosted the truly spectacular event.
ECS President Turgut Gür and Past President Eric Wachsman led the ECS delegation, which included Society board members Gerri Botte (ECS Senior Vice President), Shirley Meng (ECS Battery Division Chair), and Christopher Jannuzzi (ECS Executive Director/ Chief Executive Officer). At the event, the Society was proud to make two special announcements in recognition of Prof. Goodenough’s incredible career and achievements.
The first is the release of a combined JES and JSS Focus Issue in Honor of John Goodenough: A Centenarian Milestone. In addition, the ECS Board of Directors recently founded the John B. Goodenough Award of The Electrochemical Society, a tribute to his distinguished contributions to the fundamental and technological aspects of electrochemical materials science and engineering. The winner of the inaugural Goodenough Award—the first new Society-level award in many years—will be announced in May 2023 at the 243rd ECS Meeting in Boston, MA. It was an honor for The Electrochemistry Society to present John with a bound copy of the focus issue and a commemorative scroll announcing the Goodenough Award during his celebration!
Please join us in sending John our heartfelt wishes for continued good health and productivity. We thank him for his groundbreaking advancements to the fields of electrochemistry and solid state science, which enable the solutions to the grand challenges facing the planet. Learn more about John’s involvement with the Society and groundbreaking achievements.
The ECS Board of Directors met on June 2, 2022, in conjunction with the 241st ECS Meeting held in Vancouver, British Columbia. It was the board’s first in-person meeting since October 2019, although several board members and staff joined remotely.
ECS President Eric Wachsman called the meeting to order, and thanked the board for their continued leadership, support, and dedication to ECS through the challenging times we have faced together as an organization. He also remarked how important—and inspiring—it is to meet face-to-face again.
ECS Secretary Marca Doeff then presented the previous board meeting minutes and had the pleasure of welcoming newly elected division chairs and ECS Board of Directors members: Uroš Cvelbar (DS&T division), Maria Inman (IE&EE division), and Jeff Blackburn (NANO division). Congratulations to Uroš, Maria, and Jeff!
Next, ECS Treasurer Gessie Brisard gave a brief update on the state of the Society, highlighting the strong year ECS had in 2021, despite the myriad difficulties ECS and the world faced. In financial terms, 2021 was a very successful year for the Society. Bolstered by dramatically reduced operating costs, substantially increased revenues from publications operations, and the outstanding performance of our investment portfolio, ECS’s net assets increased by over $3M in 2021. Also contributing to the financial success of our operations was our rapid transition to successful digital meetings, which significantly limited our COVID-related losses. Such financial results are only possible if the Society is functioning well, and most importantly, our community is meaningfully engaged. Clearly, the 2021 results indicate that this is very much the case!
The board also heard important updates from the Institutional Engagement, Individual Membership, Education, Audit, Technical Affairs, Nomination, and Honors and Awards Committees, and the Council of Past Presidents.
Two main strategic initiatives emerged from the meeting. The first deals with membership and finding ways to lower the barriers to joining ECS. The challenge we are trying to address is that engagement with ECS—in terms of articles published and downloaded, number of meeting attendees registered, grant and award nominations received, to name a few—is up dramatically, and has been for years. However, membership numbers are flat or declining. This begs the question, how can we structure membership so that it is attractive and accessible to the larger cross section of the engaged electrochemical and solid state community, while at the same time offer a suite of benefits that ECS can provide at scale as membership grows? The Individual Membership Committee and associated staff will begin drafting a plan to meet this initiative’s goals to share at the next board meeting.
The other major strategic initiative is an effort for ECS to partner with other groups (such as the US Department of Energy and NAATBatt International) to address the critical workforce training needs of the battery industry. For more on this effort, please see the article entitled “ECS Battery Workforce Development” on page 24.
With the meeting’s close, board members Peter Mascher (DS&T), Shrisudersan Jayaraman (IE&EE), and Hiroshi Imahori (NANO) completed their terms. Many thanks to Peter, Sudha, and Hiroshi for their many years of service and dedication to the board and Society.
The board congratulated and sincerely thanked ECS officers Gessie Brisard (Treasurer) and Eric Wachsman (President) for their truly inspired leadership. The COVID-19 pandemic affected their entire terms as ECS officers. Their guidance and unwavering commitment helped the Society successfully endure the challenges we faced together. We wish all the best to Gessie and Eric!
The board reconvenes at the 242nd ECS Meeting in Atlanta, GA, on October 13, 2022.
The 7th edition of Next Generation Electrochemistry (NGenE) took place at the University of Illinois at Chicago (UIC) June 6–10, 2022. The meeting was in person for the first time since 2019, after two years of online programming due to the COVID-19 pandemic. Showcasing the growing diversity of our scientific community, 36 advanced graduate students and postdocs and 10 world-renowned experts gathered from institutions all over the US to discuss research frontiers in electrochemistry.
The theme of NGenE 2022 was “Electrochemistry for Decarbonization.” Lectures by the NGenE faculty provided a highlevel overview of the current body of knowledge, which they used to highlight critical gaps preventing transformative advances. The talks were structured to promote vigorous discussion driven by the participants, who gladly obliged. The program’s specific focus spanned different applications of electrochemistry targeting the wholesale reduction of emissions, such as direct conversion of CO2, new technologies with low carbon intensity, and the water-energy nexus. The lectures also discussed the most modern approaches to understanding electrochemistry, including cutting-edge instrumentation, the rising tide of machine learning, and operando and automated experimentation. A demonstration of cutting-edge tools of in-situ electron microscopy at UIC complemented the lectures. Grouped in teams, the students developed a document
and presentation laying out what they perceived as the most crucial question facing future generations of electrochemists. NGenE pursues training goals beyond pure science, so the group exercise was structured as an opportunity to practice soft skills to distill and communicate important concepts. During the week, participants networked through social events, such as the Mid-America Section Welcome Reception, hosted by the newly created ECS Mid-America Section. The meeting included a poster session and a discussion of careers in electrochemistry with electrochemists in different paths, which included NGenE alumni.
The NGenE organizing team is already working toward and looking forward to NGenE 2023. They seek input from the community to shape the critical features of our program, which focuses on fundamental aspects of electrochemical science. Email the Program Director Dr. Jordi Cabana with your suggestions. George Crabtree is Supporting Director. Next Generation Electrochemistry is supported by the National Science Foundation through award XC1661629. BioLogic USA sponsors the event, which is endorsed by The Electrochemical Society and the Materials Research Society.
The Winter 2022 issue of Interface will be guest edited by the Luminescence and Display Materials Division’s Eugeniusz Zych 2022 will also include reports from ECS Summer Fellows, a celebration of the Electrodeposition Division’s 75th
Anniversary, an article about the invention of the transistor for its 75th anniversary, columns from our new contributing editors, as well as The Chalkboard, Free Radicals, and Looking at Patent Law, and the latest people, student, and society news.
George Crabtree (University of Illinois at Chicago and Argonne National Laboratory) delivered the NGenE 2022 inaugural lecture. NGenE 2022 participants on the grounds of the University of Illinois at ChicagoDinia Agrawala joined ECS in August 2007 as the Interface Production Manager; her current title is Graphic Design and Print Production Manager. Dinia is responsible for the layout, design, and production of Interface and provides designs and design consultation for other ECS publications; she also prepares all collateral and conference materials, and works with printers and mailers to determine the best delivery method and timing of all materials. She participates in the ECS biannual meetings and supports the registration desk.
Dinia is originally from Germany, where she completed her BA and master’s degrees in education at the University of Potsdam, with a double major in geography and physical education. After some initial volunteer work in the United States, she enrolled at Mercer County Community College, where she completed her associate’s degree in computer graphics. Before joining ECS, she worked in private industry, designing conference, marketing, and promotional materials, including creating and implementing a new company branding effort that incorporated all marketing and promotional materials as well as website design.
Dinia and her husband met and fell in love as pen pals— corresponding across the Atlantic between Germany and New Jersey. They have two sons who are in college discovering their interests. She is a nature lover who enjoys traveling, photography, surf-fishing, hiking, biking, kayaking, and camping with her family. “Dinia’s support and expertise in graphic design and print production are great assets to the organization,” said Shannon Reed, Director of Community Engagement. “Her willingness to take on new projects and dedicate time to learning new techniques continues to boost the look of the ECS brand.”
Cody Sullivan joined ECS in May as the Publications Coordinator of Journal Operations. Cody has a background in journalism, both broadcast and print. Most recently, Cody was an editorial assistant for a peer-reviewed medical journal, where he liaised with authors and peer reviewers to facilitate the peer-review process.
Cody is very excited to be a part of ECS. He is passionate about writing, editing, and helping scientists share their important research. In his free time, he enjoys photography, reading, and cooking.
“Cody joins ECS at a time of growth where his experience with scientific journals and excitement to support the advancement of the ECS mission is welcomed and critical to our continued growth,” said Adrian Plummer, Director of Publications. “Our team welcomes Cody, and is happy that he chose to join us on this journey.”
The Electrodeposition Division (ELDP) launched the Early Career Forum (ECF) to bring together PhD/MSc students, postdocs, and starting assistant professors or young professionals from all over the world doing research in electroless and electrochemical deposition and related fields. Today’s early career researchers are tomorrow’s experts in their respective fields. Some are in the process of writing their BSc or MSc thesis or PhD dissertation. Sometimes the research work tends to be intricate and lacks the right idea or approach to answer a particular question and achieve the desired progress. It helps to exchange knowledge, discuss ideas, and develop new strategies with other researchers at this point. The ECF aims to be a platform for the regular exchange of ideas, including short talks and open discussion sessions, allowing the members to benefit from the experience of other early career researchers. The format is similar to the ECS Student Chapters, but on an international level.
ECF members met in person at the 241st ECS Meeting to discuss current challenges and to identify other interested early career researchers. In this way, ECF served to facilitate communication and networking.
ECF thrives on the participation of early career researchers. We are looking for people who are interested in participating in the organization and providing content for our events. If you are interested in being a part of the ELDP Early Career Forum or want to learn more about it, do not hesitate to contact Dr.-Ing. René Böttcher
ELDP is hosting a centennial symposium, E04 – 100 Years of the Electrodeposition Division: Past, Present, and Future, at the 242nd
ECS Meeting in Atlanta. ECF is organizing a part of the centennial symposium to showcase contributions from outstanding early career researchers involved in cutting-edge research across a broad range of areas of active electrodeposition research. ECF also organized the ELDP Centennial Celebration Logo Design Competition. All ELDP division members were invited to participate and submit ideas for a “100 Years of the ECS Electrodeposition Division” logo design. The ELDP Executive Committee selected the best submission. The logo will feature prominently at all ELDP symposia at the meeting.
The Energy Technology division (ETD) has long held its annual business luncheon at the spring ECS meeting. At this event, the ETD Executive Committee typically discusses the division’s state of health, major initiatives, new members, committees, and other division matters. However, the event’s main purpose is to publicly honor the recipients of the division’s three spring awards—the ETD Graduate Student Award Sponsored by BioLogic, Supramaniam Srinivasan Young Investigator Award, and the most senior award, the ETD Research Award. Though these awards have continued to be given every year, unfortunately, the COVID-19 pandemic prevented the division from coming together to properly recognize and honor the deserving winners.
The ETD business luncheon took place in person for the first time in three years at the 241st ECS Meeting. The event was well attended, with approximately 50 division members joining the celebration. Almost all the past three years’ winners were present to receive their awards and address
the division’s members. In attendance were Grace Lindquist (2022), Zachary Schiffer (2022), and Charles Wan (2021) for the Graduate Student Award; Dr. James Young (2022) and Prof. Iryna Zenyuk (2021) for the Srinivasan Award; and Prof. Vito di Noto (2022) and Prof. Paul Kenis (2020) for the Research Award. These awardees represent the absolute best that the division has to offer. It was the Society’s honor to hold this event in person and give these individuals the recognition they deserve.
Please remember to nominate our colleagues for these awards in 2023. The submission deadline is September 1!
ELDP Early Career Forum members connect with other early career researchers over sushi at the 242nd ECS Meeting in Vancouver. Grace Lindquist, University of Oregon, accepts the ETD Graduate Research Award Sponsored by BioLogic from ETD Chair William Mustain Prof. Vito di Noto from the Università degli Studi di Padova delivers his 2022 ETD Research Award address.At its business lunch at the 241st ECS Meeting in Vancouver, the IE&EE division acknowledged division award winners.
Matthew Liu received the 2022 Student Achievement Award. He is a PhD student in the Chemical Engineering Department at Stanford University and a NASA Space Technology Graduate Research
Fellow. As a member of William Tarpeh’s lab, Liu researches electrochemical reactive-separation processes to recover ammonia from nitrogen-rich wastewaters.
Urban Electric Power received one of the 2021 Industrial New Electrochemical Technology (NET) Awards for their work in developing sustainable energy technologies with low carbon footprints.
A special panel discussion titled “Advanced Electrolysis Systems for Renewable Energy Conversion and Storage” took place on May 30 at the 241st ECS Meeting. The panel was of particular interest to the ECS Energy Technology Division and the ECS Industrial Electrochemistry and Electrochemical Engineering Division. Dr. Hui Xu of Giner, Inc. moderated the discussion, which featured panelists Grigorii Soloveichik of the US Department of Energy; Cortney
Mittelsteadt of Plug Power Inc.; Meng Tao of Arizona State University; and James Fenton of the University of Central Florida. Panelists discussed how electrolysis can be used as a means of renewable energy storage, the grandest challenges for the integration of electrolyzer technology with renewable energy like solar and wind, and the most viable and immediate market or end users for green H2 and other green chemicals.
(Left to right) IE&EE Division Chair Shrisudersan Jayaraman and IE&EE Division Student & Early Career Awards Chair Elizabeth Biddinger present the division’s Student Achievement Award to Matthew Liu (Left to right) IE&EE Division Secretary/Treasurer Paul Kenis and Chair Shrisudersan Jayaraman present the division’s NET award to Urban Electric Power, represented by Gautam Yadav, Director of Advanced Battery Development.Y. Shirley Meng, Chair University of Chicago
Brett Lucht, Vice Chair
Jie Xiao, Secretary
Jagjit Nanda, Treasurer
Doron Aurbach, Journals Editorial Board Representative
James Noël, Chair
Western University
Dev Chidambaram, Vice Chair
Eiji Tada, Secretary/Treasurer
Gerald Frankel, Journals Editorial Board Representative
Uroš Cvelbar, Chair
Jožef Stefan Institute
Sreeran Vaddiraju, Vice Chair
Zhi David Chen, Secretary
Thorsten Lill, Treasurer
Peter Mascher, Journals Editorial Board Representative
Natasa Vasiljevic, Chair University of Bristol
Luca Magagnin, Vice Chair Andreas Bund, Secretary Antoine Allanore, Treasurer Takayuki Homma, Journals Editorial Board Representative
Qiliang Li, Acting Chair/Vice Chair George Mason University
Vidhya Chakrapani, 2nd Vice Chair
Zia Karim, Secretary
Erica Douglas, Treasurer
Fan Ren, Journals Editorial Board Representative Jennifer Bardwell, Journals Editorial Board Representative
William Mustain, Chair University of South Carolina
Katherine Ayers, Vice Chair
Minhua Shao, Secretary
Hui Xu, Treasurer
Xiao-Dong Zhou, Journals Editorial Board Representative
Sean R. Bishop, Chair Sandia National Laboratories
Cortney Kreller, Sr. Vice Chair Xingbo Liu, Jr., Vice Chair
Teruhisa Horita, Secretary/Treasurer Xiao-Dong Zhou, Journals Editorial Board Representative Industrial Electrochemistry and Electrochemical Engineering
Maria Inman, Chair Faraday Technology, Inc.
Paul Kenis, Vice Chair
Elizabeth Biddinger, Secretary/Treasurer
John Harb, Journals Editorial Board Representative
Rong-Jun Xie, Chair Xiamen University
Eugeniusz Zych, Vice Chair
Dirk Poelman, Secretary/Treasurer Kailash Mishra, Journals Editorial Board Representative
Jeff L. Blackburn, Chair National Renewal Energy Laboratory Ardemis Boghossian, Vice Chair Yan Li, Secretary Hiroshi Imahori, Treasurer Francis D’Souza, Journals Editorial Board Representative
Sadagopan Krishnan, Chair Oklahoma State University
Song Lin, Vice Chair
Jeffrey Halpern, Secretary/Treasurer Janine Mauzeroll, Journals Editorial Board Representative
Andrew Hillier, Chair Iowa State University
Stephen Paddison, Vice Chair Anne Co, Secretary Svitlana Pylypenko, Treasurer David Cliffel, Journals Editorial Board Representative
Jessica Koehne, Chair NASA Ames Research Center
Larry Nagahara, Vice Chair
Praveen Kumar Sekhar, Secretary Dong-Joo Kim, Treasurer Ajit Khosla, Journals Editorial Board Representative
Jie Xiao , Electrochemical Society Fellow (2020) and Battelle Fellow at the Department of Energy (DOE)’s Pacific Northwest National Laboratory, has received the 2021 Ernest Orlando Lawrence Award. The award recognizes mid-career scientists who have made exceptional contributions in research and development that support the DOE and its mission to advance the national, economic, and energy security of the United States. Dr. Xiao was honored for “significant scientific contributions in integrating materials science and electrochemistry across scales to advance both the state of science and the state of technology in emerging electrochemical energy storage systems.”
A founding member and current chair of the ECS Pacific Northwest Section, Dr. Xiao is the Secretary and former Treasurer of the Battery Division. She served on the Institutional Engagement Committee from 2018 to 2021. She received the Battery Division Technology Award in 2020.
Her research focuses on addressing scientific challenges to enable new technology to bring about improved batteries for electric vehicles—batteries that last longer and are less expensive and lighter than those available today. Her research thrusts encompass both the practical applications and the fundamental study of energy-related materials and systems, spanning microbatteries for acoustic fish tags to advanced battery and flow battery technologies for vehicle electrification and stationary applications. She is an affiliated professor and a UW-PNNL Distinguished Faculty Fellow in the Materials Science & Engineering Department at University of Washington.
Dr. Xiao has published more than 100 peer-reviewed journal papers (h-index 72; >21,225 citations) and 2 book chapters. She has been a top 1% highly cited researcher in materials chemistry continuously since 2017. She also holds 12 patents in the field of energy storage with another 11 patents application pending. Three of her patents have been licensed to industry companies.
We are very pleased to share that our colleague, Prof. James (Jamie) Noël, was named a 2022 Faculty Scholar at Western University. For a number of years after he completed his PhD in 2000 at the University of Manitoba, Prof. Noël was a Research Scientist and leader of his own research projects in Prof. David Shoesmith’s group at Western University. Independently, he developed electrochemical-neutron reflectometry techniques to study the influences of hydrogen on passive oxide films, and jointly led projects on nuclear waste container corrosion funded by the US Department of Energy. From 2016, when Prof. Noël assumed leadership of the Western University group, through his 2021 promotion to Associate Professor, he made outstanding research advances recognized by election as a 2022 Fellow of The Electrochemical Society and by receiving the 2018 ECS Canada Section R.C. Jacobsen Award
The Office of the President of Western University administers the Faculty Scholar Awards, which recognize significant recent scholarly achievements. Recipients are all-around scholars with strong international presences. They receive CAD $15,000 for scholarly activities each year of the two-year award.
Prof. Noël and his co-researchers have made extraordinary strides toward the grand global challenge of establishing a means
for safe and permanent disposal of high-level nuclear waste. To tackle this multifaceted challenge, Noël established and leads novel cross-disciplinary collaborations with academic, industrial, and government partners in Canada—most notably the Nuclear Waste Management Organization (NWMO) of Canada—and around the world—in particular, Sweden, Finland, and Switzerland. An especially novel aspect of this research is a comprehensive attempt to determine the influences of microbial effects within geologic materials on corrosion processes. The research’s implications are potentially important not just for nuclear waste containers, but also for the corrosion of a wide range of buried industrial installations. Noël also collaborates with 18 other researchers to develop nextgeneration anti-corrosion coatings for protecting structures ranging from ships, industrial plants, and bridges, to microelectronic circuits and nanoparticles for cancer treatment. He is an enthusiastic and committed contributor to training students and researchers in class and through research mentorship, as demonstrated by his students’ numerous awards. His trainees routinely secure careers in industry or academia. ECS members are familiar with Noël’s tireless efforts over the last 12 years to develop and deliver highly popular short courses on the fundamentals of electrochemistry. His exceptional research and teaching accomplishments exemplify the characteristics of an impactful all-around researcher. He highly deserves the Western University Faculty Scholar Award.
Noam Eliaz has been appointed 11th Dean of The Iby and Aladar Fleischman Faculty of Engineering at Tel Aviv University. His tenure began on July 1, 2022. Prof. Eliaz is a full professor, Director of the Biomaterials and Corrosion Laboratory, and the founding first Chair of the Department of Materials Science and Engineering at Tel Aviv University. He received the 2021 Electrodeposition Division Research Award, and he has chaired the Research Award Review Subcommittee since 2022.
“I am looking forward to working with our terrific faculty, administrative staff, technical staff, students, and alumni, along with our partners in industry and government, to bring the faculty to even greater heights and increase its global impact. Engineering at Tel Aviv University has a culture that supports interdisciplinary collaboration and innovation and is committed to excellence in both research and education. I invite anyone who wishes to be part of this exciting journey to join us and help build the future of engineering as we tackle the diverse set of grand challenges and opportunities in front of us,” said Prof. Eliaz.
The 2022 Gordon Research Seminar (GRS) and accompanying Gordon Research Conference (GRC) on Aqueous Corrosion were held July 9–15, 2022 at Colby-Sawyer College in New London, NH. The GRS was entitled “Design of Modern Prediction Tools and Sustainable Corrosion Protection Strategies,” with Oumaima Gharbi (CNRS- Sorbonne Université) and Saba Esmaeely (Det Norske Veritas) as Chairs. The GRS participants were graduate students, postdoctoral associates, and other scientists with a similar level of experience. After a keynote address by Prof. Jenifer Locke (Ohio State University), three lecture sessions consisting of twelve talks and two poster sessions consisting of 65 posters provided an opportunity for networking, discussion of new ideas, and planning for collaborations. The 2024 seminar will be co-chaired by Evangelia Kiosidou (Ohio State University) and Michael Strebl (FriedrichAlexander Universität Erlangen-Nürnberg).
The GRC was entitled “Precise Measurement, Mechanistic Understanding, and Enhanced Avoidance of Corrosion,” with Jamie Noël (Western University) as Chair and Mary Ryan (Imperial
College) as Vice Chair. The meeting was a great success, with 20 excellent talks stimulating vigorous discussion periods with broad participation among the 125 attendees. Three students (Sam Inman [University of Virginia], Ryan Katona [Sandia National Laboratories] and Brianna Rector [Western University]) from the GRS were selected by their peers to give short lectures at the GRC.
A large group hiked Mt. Kearsarge, as shown in the accompanying photo. During Thursday afternoon, the Rest of the World Team defeated the United States Team 3-2 in a tightly contested soccer match. In addition, Bill Eggers (BioLogic USA) and Jeff Binns (Nuclear Waste Management Organization) tied for first place in the annual golf competition, thus sharing the coveted Mike Graham Trophy. This tie was the first in the history of the competition and was soon followed by a tie in the election for the next Vice Chair, so Jenifer Locke and Arjan Mol (Delft University of Technology) will be Co-Vice Chairs for the 2024 conference, for which Mary Ryan will serve as Chair.
ECS Emeritus Member Ashok Vijh was named Honorary Fellow of the Canadian Academy of Engineering (CAE) in June 2022. Honorary Fellows are academy members whose peers nominate them for honorary status in recognition of exceptional achievement.
Dr. Vijh is Maître-de-Recherche at the Institut de Recherche d’Hydro-Québec and served as Invited Professor at the Institut National de la Recherche Scientifique of Université du Québec (1970–2020). His research has advanced electrochemical technologies that include fuel cells, advanced batteries, hydrogen electrolyzers, and photoelectochemical solar devices as well as for treatment of cancerous tumors. His work has been recognized by the award of over 60 distinctions. Among them, he has received the 2012 Diamond Jubilee Medal and 2002 Golden Jubilee Medal from Her Majesty Queen Elizabeth II. The Canada Council for the Arts awarded him the 1987 Izaak Walton Killam Memorial Prize. He is a Fellow of the Royal Society of Canada (RSC); former president of the RSC Academy of Science; Officer of the Order of Canada and Order of Québec; and Knight of the Order of Montréal. He has published more than 400 refereed papers and eight books on various areas of interfacial electrochemistry.
With deep sadness we inform the ECS community that Prof. Eliezer Gileadi, one of the greatest electrochemists of his generation, Fellow of the ECS (1997) and winner of the Olin Palladium Award (2003) and the Henry B. Linford Award for Distinguished Teaching (2008) passed away on May 31, 2022.
Eliezer was born in Budapest, Hungary, on April 15, 1932 and immigrated to Israel together with his family in February 1940, at the age of 8, escaping Nazi Germany. In 1951 he obtained his bachelor’s and master’s degrees from Hebrew University, Jerusalem, specializing in physical chemistry. He earned his PhD under the supervision of Prof. Brian E. Conway at the University of Ottawa, specializing in electrochemistry. Later, Eliezer worked as a Senior Research Associate and a Fuel Cells team leader in John Bockris’s lab at the University of Pennsylvania. A long-standing member of the ELDP Division, Eliezer joined ECS in 1965.
Throughout his career, Eliezer won numerous prizes and recognitions, including Fellow of the Electrochemical Society and Fellow of the AAAS, the International Society of Electrochemistry, and the International Society for Pure and Applied Electrochemistry. He was a Fellow of the Royal Society of Canada and a member of its Academy of Sciences. In 2014, he received the Gold Medal of the Israel Chemical Society.
His contribution to Israeli society was exceptional. In 1994 he established the Gileadi Program, which provided over 500 university research positions to first-class immigrant scientists from the former Soviet Union. That program was later extended to the Kamea Program. The highly successful Gileadi and Kamea Programs, which
Dr. Vijh, who joined ECS in 1968, is a member of the Society’s Physical and Analytical Electrochemistry Division and Canada Section. He received the Canada Section’s 1973 W. Lash Miller Award and 2018 Electrochemical Award (the latter was the first time the award had been given in eight years).
transformed science in Israel, were highlighted in a study published in Science.
Since joining Tel Aviv University in 1966, Eliezer became a recognized leader in the domestic and international electrochemistry communities. He educated generations of world-renowned electrochemists and influenced the community with his widely consulted publications, including more than 200 scientific papers, three textbooks, and numerous book chapters. He received several patents on a wide spectrum of electrochemical applications.
Gileadi developed the combined electrosorption isotherm, which provided the first understanding of the effect of molecular size on electrode kinetics. Measuring Tafel slopes with unprecedented accuracy, Gileadi could establish the temperature dependence of the transfer coefficient, a central question in electrode kinetics. He also demonstrated that Grothuss-type hopping conductivity could occur with halide ions in liquid and solid bromine and iodine, showing for the first time that unusual conductivity mechanisms can involve ions other than protons. His long list of achievements also includes the theory of microelectrode assemblies, electro-deposition of active metals in non-aqueous solutions, and corrosion in non-aqueous media. He also developed new methods for studying adsorption isotherms using electrochemical quartz crystal microbalance (EQCM) in gas and liquid phases.
Eliezer Gileadi will be greatly missed. He was a wonderful person, always willing to listen, and give advice and help.
The ECS and the entire community of Israeli scientists mourn the loss of a great scientist. He has been and will be forever considered “the father of Israeli electrochemistry.” May His Memory Be for a Blessing.
This notice was contributed by Prof. Noam Eliaz; Prof. Ehud Keinan, on behalf of the Israel Chemical Society; and Prof. Diana Golodnitsky, on behalf of the Israel Electrochemical Society.
Ashok Vijh was elected an Honorary Fellow of the Canadian Academy of Engineering in June.The ever-expanding number of publications in the science community has motivated many investigations on methodologies to assess the impact of the papers and of researchers as authors, with the tradeoff between quality vs. quantity not far behind.1-5 These discussions are enabled by the readily accessible relevant data, which together have renewed the interest in the field of scientometrics. In the 1950s, one of the early researchers of scientometrics, Derek J. de Solla Price, discussed quantifying the quality of research and measuring scientific progress for fields and individuals, including evaluation of his projections for the number of research journals and total articles, namely, the “size” of science.6,7 With the availability of numerous online databases, along with growing interest in journal impact factors and in citation metrics, came debates on indicators used for citation analysis and metric comparison,5,8-11 authorship criteria,12-14 and hyper-publishing.1,2, 12 While some of these studies have started with simple relationships between h-index and citations for numerous disciplines, some other investigations have focused on indepth analysis to discuss the limitations of the h-index, and or of any single metric for that matter, as a means to measure scientific output or to evaluate the quality of a researcher’s work.
This article provides a simple analysis of h-index vs. citations for the field of electrochemistry based on the total publication infometric of ECS fellows selected based on their available Google Scholar profiles. Providing a critical review of the h-index is beyond the scope of this article, yet with the availability of online bibliographic information and publication databases, such investigations could be undertaken in the future.
In 2005, J. E. Hirsch introduced an easily computable h-index in his work to assess nominally the quality of a researcher’s output, “An Index to Quantify an Individual’s Scientific Research Output.”8 h-index is defined as the number of papers with a citation number of at least h. Since then, it has been pervasively adopted by major citation databases (e.g., Google Scholar, Web of Science, and ResearchGate). But, like any such simplified quantification, its ability to reflect the true measure of the productivity of a scientist/researcher has fueled controversies as to its true capability, especially its reliance on a single measure of the number of publications and citations with equal footing for each such entry. Even Hirsch himself noted3 that “a single number can never give more than a rough approximation to an individual’s multifaceted profile, and many other factors should be considered in combination in evaluating an individual,” and discussed the influence of affecting/skewing topical areas, self-citations, and especially the number of co-authors (i.e., the misrepresentation of contribution) in more detail.3,8 He further noted that “it may be useful in comparing different individuals to normalize h by a factor that reflects the average number of coauthors.”8 Nevertheless, the h-index, in its originally proposed simple form, remains the default metric today.
A rather less discussed aspect of Hirsch8 is the relationship between the total number of citations, Nc , and the h-index:
Fig. 1. An illustration of a hypothetical citation distribution for publications. The area under the curve yields the total number of citations, whereas the area of the blue box, h2, is the citations from the papers that directly contributed to the h-index.
Nah c = 2 (1)
The proportionality constant a varies between 3 and 5, based on empirical findings, and exhibits a dependence on the field of study (e.g., physics, mathematics).3,8,11 It can also be represented graphically (Fig. 1), where h2 is the area representing a fraction of total citations in a citation-publication distribution curve. Using mathematical analysis, this relationship was shown to hold in general (i.e , as a “rule of thumb”), except when biased by a few papers or books with significantly higher citations.11
It would be of interest to understand what proportionality constant (a) is, and if the applicability of the relationship holds for the field of electrochemistry. To enable an accurate sampling of data and cumulative impacts, a set of established researchers in the field, namely, Fellows of the Electrochemical Society (to be referred herein
as “ECS fellows”) is used.a Their citation information is retrieved from their available profiles in Google Scholar, which include total citations, Nc; h-index, hi; and papers with at least 10 citations, i10.
To focus on the overall correlations, author names are used as randomized index variables and the selected subsets of correlations are plotted in Fig. 2.b
Figure 2a shows a wide distribution of total citations for over 200 ECS Fellows based on their bibliographic information. Considering the varying scientific age of fellows, a large spread is not surprising; yet even for fellows from similar cohorts (year inducted), variations exist. Some of these variations could also be related to the differences in subtopical research categories (even within electrochemistry).
The dark bars in Fig 2a also show the share of citations for each author by their papers that contribute to their h-index, h2 (h-squared). To examine this contribution visually, hi2 is plotted in log scale against total number of citations, Nc, for each author (Fig 2b). In this plot, the values from the last five years are also included in gray squares, a
Fig. 2. (a) Distribution of total citations for ECS fellows and contributions from the papers that belong to h-index (dark blue) and i10index (light blue). The rest of the bar (gray) represents the citations from the rest of the publications. The same data is plotted in log-log scale in terms of (b) h2 i vs. total number of citations, Nc, where each circle represents an author, and its color intensity is mapped to the year of fellowship. Gray squares represent the value pairs from the last 5 years. The lines represent different ratios (slopes). (c) h2 i vs. total number of citations, Nc, multiplied by 0.25 based on the best-fit match for proportionality factor (r-square: 0.95, purple line). The values are retrieved from Google Scholar (May 2022).
a Correlations among metrics are obtained after creating a summary of the bibliographic records for ECS fellows by matching their name and affiliation as listed on the ECS website with the information from their individual Google Scholar profiles (if available), which is readily accessable and provides a recognized database of up-to-date citations. The data are retrieved by Python script Scholarly (10.5281/zenodo.5764801).
b At this point, it must be noted that this article is an exercise on h-citation correlations in an effort to determine the proportionality constant (a) and share observations in the field of electrochemistry. This article is not a guidance on the ECS fellowship criteria, nor does it provide causations for such correlations or compare fellows.
record also available in Google Scholar. Overall, one can observe a strong correlation (r2 = 0.95) which suggests that the following relationship between Nc, hi holds with a proportionality factor, a = 4 (line in Fig 2c):
NhcihN ic ==4025 22 or. (2)
This means that approximately 25% of all the citations of an author (“ECS fellow”) come from their papers that directly contributed to their h-index (h2). In other words, the h-index appears to grow with the (square root) of total citations () hN ic 2 ∝ , rather than with the number of publications. While this correlation confirms the
validity of the h-Nc relationship with a proportionality factor for a representative community of authors, any further interpretation would require additional analysis and considerations.
In some ways, this relationship only illustrates the simplicity of the h-index, which can also be seen as one of its limitations. For example, this analysis does not reveal much about the discussion of quality vs. quantity without additional correlations on the number of publications, and time-dependent changes in these correlations. Moreover, one needs to account for the total research output, as well as the number of authors per publication, or whether the author is the first or the senior author for each publication, to obtain more insightful correlation with respect to the scientific contribution or impact of each author. In addition, a quick examination of the numbers revealed some ECS fellows who spent a majority of their career in industry, where their accessible publication output is relatively reduced (e.g., due to a higher number of patents than journal articles), which could skew the citations or h-index, especially because not all publication databases register citations to patents. Thus, even from a visual analysis of these simpler metrics, several factors stand out that render the suitability of h-index or citations questionable for comparisons across fields or affiliations (e.g , academic vs. industry researchers). Because the publication infometrics of ECS fellows clearly cut across h-indexes (Fig 1a), this adds weight to the validity of the observed correlations (Fig 2b-c), which, however, merely provides a quick snapshot of the publication system and should still be interpreted with reservations.
In fact, in an effort to overcome h-index’s possible shortcomings, many studies in the last decade have either proposed new metrics, by accounting for factors such as highly cited papers or excess citations,3,11,15 and adjusting for co-authors,5,8 or examined correlations among many indicators using bibliographic records,3,9,16 with some laying the mathematical foundations for these statistical observations.5,8-11,15,17 Some other recent reports noted the misleading nature of using single values for research assessment and called for a broader range of metrics and distribution of citations.18,19 Further, studies show authorship criteria vary widely across fields and geographic regions,13,14 which make such assessments highly challenging.
A conceptually related discussion is the journal impact factor (JIF), which also uses citations and publications, albeit for evaluating the “impact” of journals, not researchers. The JIF is the ratio of total citations to the total number of “citable articles” within a time period, which usually varies within a few years. While a comparison of these methods is beyond the scope of this article, it is worth noting the discrepancy between the time periods used to evaluate a researcher’s output/impact (“scientific age”) and the shorter time periods used in journal metrics, which in fact leads to another debate. Consequences of such overlooked effects have been discussed by Januzzi and Taylor in their article in Interface, “Quantifying Quality.”4 They note that “The frequency with which research published in JES is cited in patent literature, coupled with its long cited half-life metric, supports the assertion that content published in the journal has a high degree of utility as well as a long period of relevance […] Therefore, rather than relying on a single such measure, it is important to consider a wide array of metrics in order to form a holistic understanding of the overall quality and prestige of a given journal.”
Consequently, as the renewed interest in scientometrics intersects with the growing field of electrochemistry and the availability of data and subsequent analysis, critical evaluations of the publication system, and discussions on assessing the impact of research (quality vs. quantity) are likely to continue. This article should be viewed not as a critical analysis of the subject matter, but a humble thought experiment to stimulate the conversation on infometrics in the field of electrochemistry.
© The Electrochemical Society. DOI: 10.1149/2.F02223IF
Ahmet Kusoglu, Staff Scientist, Lawrence Berkeley National Laboratory Education: PhD (University of Delaware), Post-doctoral research at LBNL on fuel-cell membranes’ durability and performance.
Research Interests: Ionomers, Polymer Electrolytes, Mechanochemistry, StructureProperty Relationships, Fuel Cells and Electrolyzers.
Pubs + Patents: >70 peer-reviewed journal papers, 2 book chapters, >25 invited talks and tutorials at international meetings, academia, and industry forums.
Work Experience: Communications Director of the MillionMile Fuel Cell Truck (M2FCT) Consortium, and investigates data visualization and data-driven stories on science, energy, and environmental topics, including hydrogen and electrochemistry.
Awards: Srinivasan Young Investigator Award and ECS Toyota Young Investigator Fellowship.
Work with ECS: Contributing Editor, ECS Interface; ECS member for 10+ years; Member-at-large, Energy Technologies Division (ETD), past member and ex-chair of the committee for research awards; Symposium co-organizer for Polymer Electrolyte Fuel Cells.
Website: https://kusoglulab.lbl.gov https://orcid.org/0000-0002-2761-1050
1. U. Sandström and P. van den Besselaar, PLOS One, 11, e0166149 (2016)
2. V. Larivière and R. Costas, PLOS One, 11, e0162709 (2016)
3. J. E. Hirsch, Proc Natl Acad Sci U S A, 104, 19193 (2007)
4. C. Jannuzzi and E. J. Taylor, Electrochem So. Interface, 29, 8 (2020)
5. L. Bornmann and H. D. Daniel, EMBO Rep, 10, 2 (2009)
6. A. E. Jinha, Learned Publishing, 23, 258 (2010)
7. E. Orduña-Malea, J. M. Ayllón, A. Martín-Martin, and E. D. López-Cózar, Scientometrics, 104, 931 (2015).
8. J. E. Hirsch, Proc Natl Acad Sci U S A, 102, 16569 (2005).
9. M. Schreiber, C. C. Malesios, and S. Psarakis, Res Eval, 20, 397 (2011).
10. M. V. Dodson, Biochem Biophys Res Commun, 387, 625 (2009)
11. A. Yong, Not Am Math Soc, 61 (2014).
12. J. P. A. Ioannidis, R. Klavans, and K. W. Boyack, Nature, 561, 167 (2018).
13. G. S. Patience, F. Galli, P. A. Patience, and D. C. Boffito, bioRxiv, 323519 (2018)
14. H. Sauermann and C. Haeussler, Sci Adv, 3, e1700404 (2017).
15. C. T. Zhang, PLOS One, 4, e5429 (2009).
16. L. Egghe, Scientometrics, 69, 131 (2013).
17. Z. K. Silagadze, Acta Phys. Pol., B, 41, 2325 (2010)
18. E. Callaway, Nature, 535, 210 (2016)
19. R. Van Noorden, Nature, 540, 325 (2016)
In other words, what makes a given dish delectable to the palate? In my own forays into the kitchen, some culinary creations have turned out exceptionally well while others were less successful. Yet, the raw materials that went into these dishes were not drastically different, except perhaps for the relative proportions and the spices. I posit that the key lies in the complex interfaces between the dish constituents; these interact in a manner that we don’t yet fully understand.
Interestingly, interfaces are at the core of many of the processes and technologies that we study in our R&D profession. These include heterogeneous catalysis, adhesion, lubrication, etc. Indeed, all electrochemistry occurs at the interface; the double layer provides an electrostatic transition between contacting phases (e.g., solid/liquid) in electrochemical systems. Methods that provide selective and sensitive insights into interfacial processes, however, have many challenges. The advent of nanotechnology, our ability to manipulate tools at a nanoscopic level (e.g., scanning probe microscopies) or study processes at a single-molecule level have all opened new vistas on interfacial processes. Similarly, in operando experimental strategies have revolutionized our ability to watch interfacial events as they unfold in real time.
Nonetheless, we are still far short of being able to “dial in” the synthesis of a given material possessing a targeted set of desired attributes. For example, the notion of splitting water into H2 and O2 using sunlight and a semiconductor has had unlimited allure to many scientists around the globe. However, finding a magic bullet material to split water that is efficient, stable, and cost effective is still an elusive target. It would have been incredibly powerful to be able to custom design an active material for this application, one that has an appropriate bandgap to capture a sizable portion of the solar spectrum, generates a high enough voltage to split water, and is able to do this over many years of operation. Instead, we rely on painstaking trial and error methods and iterative synthesis-testing-optimization cycles much like a drug discovery exercise.
Combinatorial methodologies come to mind here and have indeed been deployed with varying degrees of success in the solar water splitting problem.
Interfaces continue to hold many “secret sauces” that remain to be unraveled. For example, in the case of semiconductor/ electrolyte interfaces, we talk about “surface states” that either serve to mediate charge transfer or act as carrier-killing trap sites. Similarly, we talk about “active sites” on a catalyst
surface. What exactly are these entities in a chemical sense? What is the secret sauce that makes copper oxide such an exquisite surface to drive the electroreduction of a very inert molecule such as CO2? It is not a surprising fact that much of the corpus of knowledge on industrial catalysis is buried in the patent literature.
Perhaps no other example illustrates the importance of interfaces as well as the formation of semiconductor/metal and semiconductor/semiconduction junctions. Early models of such contacts were modeled on the premise of abrupt junctions with negligible chemical interaction between the junction components. Now we know that not to be the case. For example, in a metal/silicon contact, interfacial metal silicide formation has been invoked to explain many nuances of the experimentally observed interfacial electrostatics. The formation and manipulation of junctions is a closely guarded secret in the microelectronics industry with much money and competitive advantage riding on this accumulated, practical knowledge.
Going back to the gastronomic metaphor, similar recipe secrets are kept by restaurants and the food/beverage industry— Coca-Cola comes to mind here! In this vein, I doubt if even the most talented chef can precisely quantify (rationalize?) the effect of every spice or trick that s/he has acquired along the journey to whip up delicious dishes in the kitchen. On the other hand, is this truly needed as long as s/he can assure the quality of the end product? In a similar vein, one can argue that the lack of intimate interfacial knowledge has certainly not hampered industrial efforts to finely optimize catalytic processes such as petroleum refining or ammonia production. There is something to be said for the paradigm: “I don’t care why or how it works as long as it works!”
Paul Cooper and Vistasp Karbhari taste-tested the above and provided invaluable feedback.
Stay tuned.
© The Electrochemical Society. DOI: 10.1149/2.F03223IF
Krishnan Rajeshwar, Distinguished University Professor of Chemistry and Biochemistry, The University of Texas at Arlington (UTA).
Education: PhD in solid state chemistry (Indian Institute of Science, Bangalore).
Research Interests: Span a broad spectrum in solid state chemistry, materials chemistry, and energy R&D.
Work Experience: After a postdoctoral stint at Colorado State University, Fort Collins, CO, joined the faculty of the
Department of Chemistry and Biochemistry at UTA.
Pubs + Patents: >400 papers in peer-reviewed journals, two monographs, and many chapters. This body of work has been cited ~22,000 times for an h-index of 70.
Honors and Awards: Include the ETD Research Award, Electrodeposition Division Award, Named to Stanford University’s World’s Top 2% Scientists list, ranked #95 in the energy category.
Work with ECS: Editor-in-Chief, ECS Journal of Solid State Science and Technology, Past president of The Electrochemical Society, Past editor of The Electrochemical Society Interface, ECS Fellow. https://orcid.org/0000-0003-4917-7790
In this installment of the ‟Looking at Patent Lawˮ articles, we present a case study of a patented invention of an o-ring sensor (SMART-OTM ) for detecting crevice corrosion in seawater piping systems used for submarine seawater cooling systems. This invention aligns with several divisions of The Electrochemical Society (ECS), including Corrosion (CORR), Industrial Electrochemistry and Electrochemical Engineering (IE&EE), and Sensor (SENS).
Recall from our previous article,1 the prosecution history (i.e., examination record) of a patent application is publicly available in the file wrapper of the United States Patent and Trademark Office (USPTO) Patent Application Information Retrieval (PAIR) system.2 With the USPTO PAIR system as the primary source of information for this case study, we illustrate the prosecution “events” encountered during the examination of US Patent No. 5,865,971; “Sealing Ring with Electrochemical Sensing Electrode.”3 The ‘971 patent issued on February 2, 1999 with inventor Mahendra K. Sunkara. Prof. Sunkara is currently a Professor of Chemical Engineering, University Scholar and Director of the Conn Center for Renewable Energy Research (Conn Center) at University of Louisville. At the time of the invention, Dr. Sunkara was employed by Faraday Technology, Inc. The assignee of the patent at the time of issue was Faraday Technology, Inc.
Prof. Sunkara is a long-time member of the ECS and a regular attendee at ECS biannual meetings. Other members of the
SMART- OTM development team are also long-time members of the ECS. The development team included Prof. Patrick J. Moran of the US Naval Academy and Drs. Maria Inman and E. Jennings Taylor of Faraday Technology, Inc. Dr. Ashwani Rawat was a PhD candidate working on-site at Faraday while a student at the University of Central Florida. Prof. Vimal Desai was his advisor. Other members of the development team included Mr. Robert Kain and Mr. Richard Hays of the Naval Surface Warfare Center (NSWC), Carderock Division. Funding for the activity was provided via a Small Business Innovation Research (SBIR) contract from the Office of Naval Research (ONR). Technical oversight for ONR was provided by Dr. A. John Sedriks. Dr. Sedriks is the author of the seminal book Corrosion of Stainless Steels, which was sponsored by the ECS.
The SMART-OTM sensor is illustrated in Fig. 1. The sensor consists of two platinum wire electrodes stitched into an o-ring. The wires are not in electrical contact and serve as counter electrode (CE) and reference electrode (RE). The SMART-OTM sensor is inserted into a flange joint to provide a seal similar to a normal o-ring. Slits in the o-ring provide contact with solution ingress into the seawater side of the flange, as illustrated in Fig. 2. The pipe flange serves as the working electrode for conducting electrochemical impedance measurements. The feasibility of the SMART-OTM sensor for detecting crevice corrosion at the laboratory scale and in flanges was demonstrated using simulated seawater at Faraday.4 Subsequently, the SMART-OTM sensor was validated in piping loops using actual seawater at the Laque Center for Corrosion in Wrightsville Beach and the Naval Research Laboratory (NRL) in Key West, Florida (Fig. 3).5,6 A typical electrochemical impedance response with a decrease in polarization resistance (Rp) indicative of crevice corrosion is shown in Fig. 4. Visual inspection of the flange joint correlated the decrease in Rp with crevice corrosion (Fig. 5). The integrity of the SMARTOTM sensor was demonstrated under high-pressure conditions.
Fig. 1. Schematic illustration of the SMART-OTM corrosion sensor. Fig 1. Schematic Illustration of the SMART-OTM Corrosion Sensor.The ‘971 patent abstract generally describes the invention as follows:
“A sealing ring containing an electrode for sensing electrochemical conditions within a fluid handling system, in particular, the occurrence of crevice corrosion, has a body formed of a continuous loop of an electrically insulating fluid-impervious material having a surface which can form a fluid-tight seal with elements of a fluid handling apparatus, an electrode embedded within the body and electrically insulated from electronic conductive contact with those portions of the surface of the body that contact the fluid handling apparatus, and channels extending between the electrode and the surface of the body of the sealing ring to permit electrochemical contact between the electrode and the fluid within the system. The sealing ring also includes electronically conductive wires to connect the embedded electrode to external electrical devices, e.g., electronic measuring equipment.”
This article summarizes the prosecution history of Patent Application No. 08/822,706 (filed on March 21, 1997), leading to US Patent No. 5,865,971. In Table I, we list the patent applications related to Patent Application No. 08/822,706. Provisional Patent Application No. 60/013,859 was filed on March 21, 1996. A claim is not required in
a provisional patent application7 and a provisional patent application expires one year from its filing date.8 The SMART-OTM development team used the provisional patent application as a placeholder while additional experimental activities progressed.
Patent Application No. 08/822,706 was filed within one year of Provisional Patent Application No. 60/013,859 filed on March 22, 1996. Consequently, the priority date for Patent Application No. 08/822,706 is the filing date of the provisional patent application: March 21, 1996.9
In the “Brief Description of the Prior Art,” the patent application notes that electrochemical measurements of seawater piping systems would be of value in the detection of hidden corrosion before catastrophic failure occurs. These measurements require an electrochemical sensing electrode in contact with the solution within the piping system. The solution contacts could be provided by a simple through-wall fitting that incorporates an electrochemical sensing electrode.
The patent application notes several challenges with throughwall fittings. For submarines and nuclear reactors, through-wall fittings could compromise the integrity of the seawater piping loops. Furthermore, since crevice corrosion is a localized phenomenon occurring between the o-ring and the sealing surface of the flange, through-wall fittings with electrochemical sensors are probably not
US Provisional 60/013,859 Sealing Ring with Electrochemical Sensing Electrode Mar. 22, 1996 Expired
US Utility 08/822,706 5,865,971 Sealing Ring with Electrochemical Sensing Electrode Mar. 21, 1997 Issued
close enough to the area where crevice corrosion occurs. Currently, the only reliable means of detecting crevice corrosion is draining of the piping loop and disassembly of the flange joint to permit visual inspection. In addition to being costly and resulting in significant down-time, the disassembly and re-assembly of the flange joint has been observed to itself cause crevice corrosion in a large number of the re-assembled flanges. Consequently, the patent application notes the continued need
“…for a simple means of inserting an electrode into a sealed fluid handling system, e.g., a piping system, in close proximity to locations in the piping system that are subject to crevice corrosion.”
The patent application summarizes the invention as addressing the need
“…by the sealing ring of this invention which comprises a body comprising a continuous loop of an electrically insulating fluid-impervious material, the body of the sealing ring having a surface with at least a portion thereof adapted to form a fluid-tight contact with a fluid-confining surface of a fluid-containing apparatus, and having embedded within the body of the ring an electrode electrically insulated from electronic conductive contact with those portions of the surface of the body that contact the fluid-confining surfaces, the sealing ring being provided with channels between the electrode and the exterior surface of the body of the sealing ring to permit electrochemical contact between the electrode and the fluid within the system and electronically conductive means to connect the electrode to external electrical equipment.”
FIG. 1 and FIG. 2 from the patent application are reproduced in Fig. 6. FIG. 1 from the patent application illustrates a fluid coupling 101 of the type wherein the sealing ring of the invention may be advantageously employed. A first fluid conduit 102 having a flange 104 and a second conduit 103 having a flange 105 are coupled by bolting the flanges 104 and 105 together with a plurality of bolts 108,
Fig 6. Figures Illustrating the Corrosion
of which two are shown. A sealing ring 110 according to the invention is clamped between the faces 106 and 107 of the flanges 104 and 105 FIG. 2 from the patent application shows an elevational crosssection of the fluid coupling of FIG. 1 from the patent application. The sealing ring 110 has a body 112 having a surface 114 and is formed of an electrically insulating fluid-impermeable resilient material suitable for forming a fluid-tight seal where the surface 114 comes into contact with the flanges 104 and 105. An electrode 116 is positioned within the body 112 of the sealing ring 110 in spaced relationship with the surface 114 of the sealing ring 110 Accordingly, the electrode 116 is not in electronic conductive contact with the flanges 104 and 105 of the fluid coupling 101. In such a fluid coupling 101, crevices, indicated generally by reference numeral 117, are necessarily formed where the sealing ring 110 contacts the faces 106 and 107 of the flanges 104 and 105. In order to monitor the electrochemical condition of the metal surface in the crevices 117, channels 118 are formed in the sealing ring 110 to permit ionic conductive contact between the electrode 116 and the crevice regions 117. The channels 118 may be open or they may be filled with an ionically conducting solid such as an ion exchange resin. If the channels are open, they become filled with the fluid within the fluid handling system of which the fluid coupling 101 is a part. In either case the electrode is in ionic conductive contact with the fluid in the crevices 117 and the adjacent metal parts of the fluid coupling that may be subject to crevice corrosion. The electrode 116 is connected to external monitoring equipment through electronically conductive lead 120 which is also insulated from contact with the metal flanges of the fluid coupling 101 by insulation 122
If the flanges 104 and 105 are not compressed too closely together, the lead 120 can be led out between the flanges 104 and 105 as shown in FIG. 1. However, one skilled in the art will understand that if the flanges 104 and 105 have to be compressed too closely together for convenient egress of the lead 120 and insulation 122, one or both of the faces 106 and 107 of the flanges 104 and 105 may be grooved or recessed to make room for the lead 120 and insulation 122, or the lead 120 with insulation 122 may be brought out through a hole made in one of the flanges.
The external monitoring equipment, which does not form a part of this invention, may be of any type which can indicate the presence of crevice corrosion in crevices 117. For example, the electrode 116 may be an electrochemically inert electrode (e.g., a platinum, titanium, or carbon fiber electrode), and an alternating potential of varying frequency can be imposed between the electrode 116 and the metal portions of the fluid conduit 110 in order to measure the complex impedance of the electrical circuit, including the crevice region 117 of the fluid coupling 101. Variations in the complex impedance may then be used to assess the corrosion status of the metal surface in the region of crevices 117 as is conventional in the art.
FIG. from patent application illustrating the flange joint with the SMART-O crevice corrosion sensor. Taylor and InmanFig 7. Figures 15, 16, and 17 from the ‘971 Patent Illustrating the SMART-OTM Crevice Corrosion Sensor.
FIGS. 15–17 from the patent application are reproduced in Fig. 7. FIG. 15 shows a plan view of a sealing ring 610 according to this embodiment of the invention, while FIGS. 16 and 17 (from the patent application) show cross-sections of the ring 610. The sealing ring 610 has a body 612 having a surface 614. A reference electrode 616 is embedded within the body 612 spaced from the surface 614 so that it is electrically insulated from any electronic conductive contact with a metal or other electronic conductor that may contact the surface 614. A counter electrode 617 is also embedded in the body 612 of the sealing ring 610 separated from the reference electrode 616 and insulated from electrical contact with the reference electrode 616, and also spaced from the surface 614. Multiple slits 618 are formed in the body 612 extending from the surface 614 to the electrodes 616 and 617. The reference electrode 616 and the counter electrode 617 each extend around a different part of the circumference of the sealing ring 610, and each electrode is exposed in the slits 618 in its part of the circumference. The slits 618 may be open or filled with an ionically conducting solid medium. The slits 618 are shown in the drawings as having a substantial width relative to the cross section of the o-ring, in order to illustrate the structure of the ring. Slits of such relative dimensions are useful. However, the slits 618 may also be very narrow, such as those made, for example, by slicing slits in an integral o-ring with a sharp instrument such as a knife or the like. All that is required is that the slits 618 provide a means for establishing ionic conductive contact between the electrode and the fluid in contact with the surface 614 of the o-ring 610. Thus, any slit having a width sufficient to allow access of the surrounding fluid to the electrode is suitable.
Similarly, the electrodes 616 and 617 are shown, for convenience in illustration, as having a substantial gauge and cross section. However, the electrodes 616 and 617 can be made of any gauge of wire, provided that a useful electrical contact between the electrode and the ionically conductive fluid in contact with the surface 614 of the o-ring 610 is established. Accordingly, the slits 618 provide an ionically conductive path between both the reference electrode and the counter electrode and the fluid in the crevices associated with the sealing ring and adjacent metal surfaces. Electronically conductive leads 620 and 621 connect the electrodes 616 and 617 to external measuring equipment (not shown). Short lengths of insulating tubing 622 and 623 (e.g., heat-shrinkable tubing of poly(tetrafluoroethylene) (PTFE)), are applied to the portions of the electrode leads 620 and 621 adjacent to the o-ring 610 in order to insulate the leads from possible contact with the adjacent metal parts of a fluid handling apparatus. The use of slits as the means for access of fluid to the electrodes 616 and 617 allows a convenient method of preparing the rings of the invention by first molding a solid o-ring, then forming discontinuous slits, such as those illustrated at 618, in the o-ring 610, and embedding the electrodes 616 and 617 in the body 612 of the ring 610 by threading the electrodes through the solid portions separating the slits (e.g., using a needle to draw the electrode wire through the body 614 of the o-ring 610).
In order to establish a filing date, a utility patent application must include:
1) Specification10
“…a written description of the invention, and the manner and process for making it…to enable any person skilled in the art…to make and use [the invention]
2) Minimum of one claim11
“…particularly pointing out… the subject matter…as the invention…”
FIG. 7. Figures 15, 16, and 17 from the ‘971 patent application illustrating the flange joint with the SMART-OTM crevice corrosion sensor.
“…where necessary for understanding the subject matter…to be patented…”
In order to maintain the filing date, the following additional criteria are required:
1) Filing fee in accordance with the current USPTO fee schedule13
2) Inventor oath or declaration asserting14 a. The patent application was authorized by the inventor(s), b. The inventor(s) believe he/she is the original inventor or they are the original joint inventors.
The patent application was filed on March 21, 1997. The patent application included:
1) specification, 2) claims, 3) drawings, 4) inventor oath/declaration, and 5) filing fee.
As summarized above, the specification included a description of the prior art, problems within the prior art, and a summary of the invention describing various embodiments of the invention addressing the prior art problems. Additionally, the patent application also included drawings illustrating the “elements” and various embodiments of the subject invention. The utility patent application contained claims directed toward a sealing ring.
The patent application included an oath/declaration signed by each of the inventors.15 The oath/declaration included an assertion by the inventors stating,
“…we declare that we are the original, first and joint inventors of the subject matter which is claimed in the patent application…and understand the contents of said specification, including the claims.”
The oath/declaration also included an acknowledgement that each inventor was aware of the penalties for a false statement,16
“I further declare that all statements made herein of my own knowledge are true and that all statements made on information and belief are believed to be true; and further that these statements are made with the knowledge that willful false statements and the like so made are punishable by fine or imprisonment, or both, under Section 1001 of Title 18 of the United States Code, and that such willful false statements may jeopardize the validity of the application or any patent issuing thereon.”
Importantly, the “named inventors” must be correctly represented on a US patent application.17 Specifically, inclusion of a colleague as a co-inventor who did not participate in the conception of the invention is known as a misjoinder and may invalidate an otherwise valid patent. Similarly, exclusion of a co-inventor who participated in the conception is known as a nonjoinder and may invalidate an otherwise valid patent. If an inventor is erroneously omitted or erroneously included as an inventor, the misjoinder/nonjoinder may be corrected and the patent remains valid.18
The filing of the patent application met the requirements to both establish and maintain a filing date and thereby avoided being abandoned.
The USPTO began publishing patent applications eighteen months after their priority date for patent applications filed on or after November 29, 2000. Since the subject patent application was filed before this date it therefore was not published.
Inventor Sunkara was employed by Faraday Technology, Inc. at the time of the invention. Consequently, the patent application was assigned to Faraday.19 The patent application included a statement asserting “small entity” status as the assignees were either a small business or a nonprofit university.20 The small entity status entitled the applicants to reduced filing, issue, and maintenance fees. In addition, the applicants appointed Thomas R. Boland of the law firm Vorys, Sater, Seymour and Pease as power of attorney
“…to prosecute the application identified above and to transact all business in the United States Patent and Trademark office connected therewith…”
The work leading to the subject invention was supported with funding from the Office of Naval Research. As stipulated in the BayhDole Act, the patent application included the following statement21
“The experimental work leading to this invention was funded in part by the Office of Naval Research SBIR Phase II Contract #N00014-94-C-0136.”
Regarding march-in rights, a key policy objective of the Bayh-Dole Act is22
“…to ensure that the Government obtains sufficient rights in federally supported inventions to meet the needs of the Government and protect the public against nonuse or unreasonable use of inventions…”
To our knowledge, the government has never exercised Bayh-Dole march-in rights in any invention.
The applicants submitted an “Information Disclosure Statement” (IDS) to the USPTO with the patent application. The IDS included prior art references as required by the “Duty of Candor.” The “Duty of Candor” requires that the inventor(s) submit an IDS within a reasonable time of submission of the patent application disclosing23
“…to the Office [USPTO] all information known to that individual to be material to patentability…”
The “Duty of Candor” is specific to any existing claim and requires that the IDS be continually updated while the claim is pending. The “Duty of Candor” ceases only when the claim is allowed and the patent issue fee is paid.
The “Duty of Candor” extends to any individual associated with the filing of the patent application, including:
1) Inventor(s),
2) Patent Counsel, or
3) Persons who are substantially involved in the preparation or prosecution of the patent application.
Substantial involvement in the preparation of the patent application could include technical assistants, collaborators, or colleagues. Substantial involvement would generally not extend to clerical workers. Furthermore, the inclusion of a reference in an IDS24
“…is not taken as an admission that the reference is prior art against the claims.”
If a finding of a violation of the “Duty of Candor” resulting in “inequitable conduct” regarding any claim in a patent is determined, then all the claims of the subject patent are rendered invalid.25 Finally, in spite of the requirement of the “Duty of Candor,” the applicant is cautioned not to “bury” the examiner with a long list of non-material references in hopes that the examiner will not notice the relevant material references.26 The specific guidance from the USPTO is to27
“…avoid the submission of long lists of documents if it can be avoided…If a long list is submitted, highlight those documents which have been specifically brought to the applicant’s attention and/or are known to be of most significance.”
The applicants hired a professional to search for relevant prior art to include in the IDS. In addition, the IDS included a “Statement of Relevancy” of each of the prior art references. While such a description is not required, the applicants felt the “Statement of Relevancy” was beneficial to assist the examiner in reviewing the prior art references. The “Statement of Relevancy” is reproduced in Table II. With the prior art search and analysis, the applicants were confident they could appropriately draft claims that would pass examination by the USPTO and provide the required business protection. Claim 1 from the patent application is reproduced herein.
Claim 1. A sealing ring for use in fluid-containing apparatus comprising:
(a) a body comprising a continuous loop of an electrically insulating fluid-impervious material, said body having an exterior surface, at least a portion of said exterior surface being adapted to contact a fluid-confining surface of a fluidcontaining apparatus and form a fluid-tight contact with said fluid-confining surface of said fluid-containing apparatus;
(b) an electrode, integral with said body, and electrically isolated from said portion of said exterior surface adapted to contact said fluid-confining surface of said fluid-containing apparatus; and
(c) means for providing electronic electrical contact between said electrode and an external electrical device;
Taylor and Inman(d) said body being provided with channels extending between said electrode and said exterior surface to permit electrochemical contact between said electrode and said fluid in said fluidconfining apparatus.
On March 17, 1998, the USPTO issued a non-final office action (NF-OA) rejecting all the claims in the patent application based on “indefiniteness”28
“…as being indefinite for failing to specifically point out and distinctly claim the subject matter which applicant regards as the invention.”
More specifically,
“Claim 1 is vague and indefinite with respect to whether the applicant is instantly claiming the sealing ring or the apparatus utilizing the sealing ring, from the instant claim as worded. It appears that applicants are claiming the fluid containing apparatus to which the sealing ring is adapted…”
Since Claim 1 was the sole independent claim and claims 2 through 14 were dependent claims, all of the claims were rejected.
Interestingly, the USPTO rejection was not based on patentability matters; that is, lack of novelty29 or obviousness.30 We attribute this “soft” rejection as due to the applicants’ use of a patent professional to search the prior art and the inclusion of the “Statement of Relevancy” of the prior art.
The applicants were given a three-month period to respond without paying additional late response fees.
(b) an electrode, integral with said body, and electrically isolated from said portion of said exterior surface adapted to contact said fluid-confining surface of said fluid-containing apparatus; and
(c) means for providing electronic electrical contact between said electrode and an external electrical device;
(d) said body being provided with channels extending between said electrode and said exterior surface to permit electrochemical contact between said electrode and said fluid in said fluidconfining apparatus.
The USPTO approved the amended Claim 1 and issued a notice of allowance on July 21, 1998. Since independent Claim 1 was allowed, all the dependent claims were similarly allowed. The patent application issued as US Patent No. 5,586,971 on February 2, 1999 after payment of the issue fees.
On June 17, 1998, the applicants responded within the three-month period with an amended Claim 1. The amended Claim 1 is reproduced herein with the inserted text depicted in [brackets].
Claim 1. A sealing ring for use in fluid-containing apparatus[, said sealing ring] comprising:
(a) a body comprising a continuous loop of an electrically insulating fluid-impervious material, said body having an exterior surface, at least a portion of said exterior surface being adapted to contact a fluid-confining surface of a fluidcontaining apparatus and form a fluid-tight contact with said fluid-confining surface of said fluid-containing apparatus;
In this installment of our “Looking at Patent Law” series, we present a case study of the prosecution of U.S. Patent No. 5,865,971; “Sealing Ring with Electrochemical Sensing Electrode.” This invention aligns with several divisions of The Electrochemical Society (ECS), including Corrosion (CORR), Industrial Electrochemistry and Electrochemical Engineering (IE&EE), and Sensor (SENS). The ‘971 patent issued on February 2, 1999 with inventor Mahendra K. Sunkara. The assignee of the patent at the time of issue was Faraday Technology, Inc. The case study begins with a brief synopsis of the background of the invention followed by 1) summary of key drawings and the specification of the invention, 2) inventor assignment and power of attorney designations, 3) submission of the Invention Disclosure Statement (IDS) and associated Duty of Candor, 4) summary of the non-final office action (NF-OA) and rejection, and 5) applicant response and allowance of the patent application. The case study illustrates the use of a professional search of the prior art and the applicant providing a summary of the prior art in a “Statement of Relevancy.” The “Statement of Relevancy” allowed the applicants to provide a convincing summary of the prior art to the USPTO and to craft well-defined claims, avoiding rejections based on lack of novelty and obviousness. With this case study, we hope to de-mystify the patent prosecution process and better prepare electrochemical and solid-state scientists, engineers, and technologists to interact with their patent counsel regarding their inventions. © The Electrochemical Society. DOI: 10.1149/2.F04223IF II. Statements of Relevancy
PATENT
3,094,337
3,788,654
4,090,029
4,453,723
4,779,903
5,090,871
5,121,929
5,246,235
5,248,080
(continued on next page)
Discloses a seal ring having anti-extrusion elements, e.g., made from a thermosetting material.
Discloses a multiple-hardness o-ring wherein a molded ring of nitrile rubber is provided with a hardened skin to increase the exterior hardness and improve the compression set characteristics.
Discloses a liquid-tight conduit connector incorporating an o-ring sealing element.
Discloses an elastomeric sealing ring incorporating a circular spring within a bore of a circumferential tubular insert.
Discloses a sealing ring for forming a pipe joint comprising an outer ring having an inner sealing ring embedded in an inward-facing groove in the outer ring.
Discloses a junction assembly with leak detecting means wherein a flanged hydraulic joint is provided with inner and outer o-ring seals, and a cavity between the seals in monitored to detect leakage.
Discloses a gasket with an encased load sensor which provides an electrical signal representative of the force applied to the gasket to an external monitor.
Discloses a seal having an embedded wire that contacts an external surface to provide a signal when the seal has become worn to a specified depth.
Discloses a metal sealing ring for connecting metal pipes wherein the ring has a meltable coating on its surfaces that are intended to engage the ends of the pipes.
E.Jennings Taylor, Founder of Faraday Technology, Inc.
Research Interest: Faraday Technology, Inc. is a small business focused on developing innovative electrochemical processes and technologies based on pulse and pulse reverse electrolytic principles. Patent Background: Taylor leads Faraday’s patent and commercialization strategy and has negotiated numerous patents via field of use licenses as well as patent sales. He is admitted to practice before the United States Patent & Trademark Office (USPTO) in patents cases as a patent agent (Registration No. 53,676). Member of the American Intellectual Property Law Association (AIPLA).
Pubs & Patents: Numerous technical pubs and presentations, inventor on 40 patents.
Work with ECS: Member for 42 years, ECS Fellow. Website: http://www.faradaytechnology.com/ https://orcid.org/0000-0002-3410-0267
Maria Inman, Research Director, Faraday Technology, Inc.
Patent Background: Inman serves as principal investigator on project development activities and manages the company’s pulse and pulse reverse research project portfolio.
Pubs & Patents: In addition to technical pubs and presentations, she is competent in patent drafting and patent drawing preparation. She is an inventor on seven patents.
Work with ECS: Member for 25 years. Serves ECS as a member of many committees.
Awards: ASTM member Website: http://www.faradaytechnology.com/ https://orcid.org/0000-0003-2560-8410
1. E. J. Taylor and M. Inman, Electrochem. Soc. Interface, 26(4), 57–61 (2017)
2. USPTO Patent Application Information Retrieval (PAIR) https:// portal.uspto.gov/pair/PublicPair
3. M. K. Sunkara,”Sealing Ring with Sensing Electrode” U.S. Patent No. 5,865,971 issued February 2, 1999.
4. M. E. Inman et. al., “Detection of Crevice Corrosion under an O‐ring by Polarization Resistance Measurements using Electrodes Embedded in the O‐ring,” Corrosion/’97, NACE International, New Orleans, LA (1997).
5. M. E. Inman et. al., “Detection of Crevice Corrosion in Natural Seawater using Polarization Resistance Measurements,” Corrosion/’97, NACE International, New Orleans, LA (1997).
6. M. E. Inman, E. J. Taylor, D.L. Myers, P. J. Moran, R. M. Kain, and R. A. Hays, “Crevice Corrosion Detection and Monitoring Inside Pipe Flanges”, U.S. Navy and Industry Exchange, Louisville, KY (1998).
7. 35 U.S.C. §111(b)(2) Provisional Application/Claim.
8. 35 U.S.C. §111(b)(5) Provisional Application/Abandonment.
9. 35 U.S.C. §119(a) Benefit of Earlier Filing Date; Right of Priority.
10. 35 U.S.C. §112(a) Specification/In General.
11. 35 U.S.C. §112(b) Specification/Conclusion.
12. 35 U.S.C. §113 Drawings.
13. https://www.uspto.gov/learning-and-resources/fees-andpayment/uspto-fee-schedule#Patent%20Fees
14. 35 U.S.C. §115(b)(1)(2) Inventor’s Oath or Declaration/ Required Statements.
15. 37 CFR 1.63 Inventor’s Oath or Declaration.
16. 18 U.S.C. §1001Statements or Entries Generally.
17. E. J. Taylor and M. Inman, Electrochem. Soc. Interface, 26(2), 45–47 (2017)
18. Manual of Patent Examination Procedure (MPEP) §1481.02 Correction of Named Inventor.
19. 35 U.S.C. §261 Ownership; Assignment.
20. 37 CFR §1.27(a)(3)(ii)(A) Definition of small entities and establishing status as a small entity to permit payment of small entity fees; when a determination of entitlement to small entity status and notification of loss of entitlement to small entity status are required; fraud on the Office.
21. 35 U.S.C. §203 March-in Rights.
22. 35 U.S.C. §200 Policy and Objective.
23. 37 CFR §1.56(a) Duty to Disclose Information Material to Patentability.
24. Riverwood Int’l Corp. v. R.A. Jones & Co., 324 F.3d 1346, 135455, 66 USPQ2d 1331, 1337-38 (Fed Cir. 2003).
25. Manual of Patent Examination Procedure (MPEP) §2016 Fraud, Inequitable Conduct, or Violation of Duty of Disclosure Affects All Claims
26. R. B. Taylor, “Burying,” Mich Telecomm & Tech Law Rev, 99, 19 (2012).
27. Manual of Patent Examination Procedure (MPEP) §2004.13 Aids to Comply with Duty of Disclosure.
28. 35 U.S.C. §112(2) (pre-AIA) Specification.
29. 35 U.S.C. §102(a) (pre-AIA) Conditions for Patentability; Novelty and Loss of Right to Patent..
30. 35 U.S.C. §103(a) (pre-AIA) Conditions for Patentability; Nonobvious Subject Matter.
TaylorOver the past 30 years Interface has highlighted the technological and theoretical advancements in electrochemistry with the motivation to inform the community at large. Each issue has a scientific theme and related notable articles that are focused on this theme. The first article is usually an overview of the articles to come and describes the motivation of the selected theme and provides background to readers on the topic.
I was personally introduced to Interface as a graduate student soon after becoming an ECS member in 2014. I remember receiving the magazines in the mail and at the time I was very much new to the field of electrochemistry. Despite this, I found myself extremely interested in the reading material—even though in many cases I understood only approximately 20% of what I was reading. I am inclined to believe that this is the case for many readers who are new to the field, as well as readers who specialize in a particular aspect of electrochemistry that the current theme does not address. For example, it may be difficult for someone specializing in corrosion to fully grasp the innovation or the creative achievements detailed in articles pertaining to biological sensors. As such, I tasked myself with developing a way in which we could describe highly technological innovations within electrochemistry in an inclusive way that would
facilitate the development of newcomers to the field and promote interdisciplinary involvement. At the same time, we would like to continue to highlight the vast ways in which electrochemistry advances society and addresses global issues.
When I think back to what inspired me most about the field of electrochemistry, it is without a doubt the wide reach of its application—from healthcare to transportation to space and beyond. Electrochemistry truly is everywhere, and I thought it would be great if we could display its wide reach through “Electrochemistry in Action.” The goal of this column is to acknowledge novel contributions that advance particular fields while engaging a new audience through detailed and easy to understand descriptions of advances in electrochemistry. Each installment will include a topic area such as electrochemistry in archaeology, steel manufacturing, water treatment, etc. and will describe a novel or unconventional application of electrochemistry. We believe that, in doing so, not only will “Electrochemistry in Action” inform readers but perhaps it will even inspire creativity and broaden interests in the field.
Sources for the column will be found through web-based searches, colleague interactions, and nominations from the ECS community. If you have an idea for an electrochemistry in action topic, please send it to Christopher L. Alexander (clalexa2@usf.edu) with the subject line “Electrochemistry in Action.” Within the email, please include a brief justification for the nomination.
ECS Interface is proud to introduce a new feature page that focuses on the award-winning research of ECS graduate students. We all know that the beginning and execution of most brilliant ideas start in the hands of graduate students, even if the principal investigator gets the headline. This feature page is intended to let students and post-docs write a primary-author perspective on their field, their
work, and where they believe things are going. The reports will be written for a general audience and in a tutorial style. The hope is to provide a concise summary that can introduce any of our readers to cutting-edge science coming directly from the bench top.
The feature will include one or two short perspectives per quarterly publication, rotating among the 13 electrochemistry and solid state science divisions, including Battery, Corrosion, Energy Technology, and Industrial Electrochemistry/Electrochemical Engineering.
I am currently writing this article for ECS Interface during back-toback weeks with several days of 100°F weather in Chicago during the month of June. Unusual weather events seem to be the norm today and for the foreseeable future. However, the most concerning aspect of climate disruption is perhaps the unpredictable nature of a warming climate. I never imagined that San Francisco would be engulfed by a shroud of orange due to wildfire smoke. The Electric Reliability Council of Texas (ERCOT) did not think a winter storm in early 2021 could cover the whole state, making power unreliable for days and resulting in more than 240 deaths. In 2017, the temperatures in Phoenix got so high that planes could not take off from the airport as the density of the air was too low. Climate change, or what I like to call Climate Disruption1—a term I have adopted from my former colleague Seth Darling at Argonne National Laboratory because industrialization has changed Earth’s climate in a fairly short time— is becoming front and center in our daily lives.
I suppose none of this information is new to the readers of ECS Interface. Many ECS members work on electrochemical science and technologies with a mission to develop and proliferate these technologies to electrify vehicle transportation, stationary power, and, more recently, industrial processes. It is halfway through 2022 and there is widespread consensus among many climate scientists that countries such as the United States need to reduce greenhouse gas (GHG) emissions by 50% by 2030 and achieve net zero emissions by 2050. There is much concern that as a society we are not moving fast enough to meet these goals. It is especially difficult to make a focused effort given all the other challenges of the day—such as high inflation and a war in Ukraine. However, it isn’t all doom and gloom and there is progress to celebrate: several major automobile manufacturers have pledged to have all of their vehicles be electric within the next 10 to 20 years, the US government is investing in several regional hydrogen hubs to help accelerate adoption of carbon neutral hydrogen as an energy vector and feedstock for decarbonizing various sectors of the US economy (e.g., ammonia production and steel refining), and many global companies are looking at ways to reduce their GHG footprint. Given all these changes taking place, it is fair to ask educators if we are doing enough to prepare a future workforce that is adept in electrochemical technologies to meet this technological sea change.
I completed my Bachelor of Science in Chemical Engineering in 2005. During my undergraduate education, many of the practical examples conveyed to teach thermodynamics, reaction kinetics, and transport phenomena hailed from downstream processing of hydrocarbon feedstocks for the manufacture of commodity chemicals and fuels. It is almost unthinkable that graduating chemical engineers today (and in the past) would not be proficient in calculating the number of theoretical trays for distillation column using McCabeThiele analysis or sizing up a plug flow reactor to meet the production of a given chemical. Considering the pressing global challenges surrounding climate disruption, I often ask myself as an educator “is it better to teach students to size up a distillation column or an electrolyzer?”
A recent story in the New York Times2 on batteries for the auto industry stated the following: “One thing is certain: It’s a great time to have a degree in electrochemistry. Those who understand the properties of lithium, nickel, cobalt and other materials are to batteries what software coders are to computers.” Given the role that electrochemistry will play in arriving at a more sustainable society, it seems justified to add a core electrochemistry (or electrochemical engineering) course to the undergraduate chemical engineering curriculum. However, adding any core course to a curriculum is a challenging proposition, as most programs run about 125 to 135 hours to earn a Bachelor of Science degree and any course addition is only possible by removing another course. Many chemical engineering departments were faced with a similar challenge in the past decade when it came to adding more process safety to the curriculum—which was advocated by many industrial companies. Several departments have added a process safety course or combined another core course with process safety. That said, sometimes it is argued that elements of process safety should be covered in all core courses so that the concepts of process safety can be reinforced multiple times over the years, rather than students getting a single dose of the concepts which are susceptible to fade from memory. I would contend that electrochemistry principles should follow the same playbook: being sprinkled across the curriculum in all core courses, starting from thermodynamics and ending in capstone courses in process design and in unit operations laboratory.
Figure 1 conveys a Greek temple structure that represents the chemical engineering undergraduate curriculum. The foundation to the structure is composed of math, chemistry, and physics. The floor on the top of the foundation is mass and energy balances and introduction to computational techniques. The foundation and floor support four columns in parallel that represent the heart of chemical engineering: thermodynamics, transport phenomena, reaction kinetics, and process control. The roof of the temple represents the capstone courses that bring it all together: unit operations laboratory and process design.
The text boxes adjacent to right of the temple in Fig. 1 present some electrochemistry concepts that are suitable for a core chemical engineering curriculum. I suggest that thermodynamics should be the first course in the curriculum to introduce electrochemistry concepts, as it is often taken by most students in the second semester of their second year or first semester of the third year. In thermodynamics, and in other core theory courses, basic electrochemistry material can be covered in one to two weeks’ worth of class time. A thermodynamics course can introduce the relationship between potential and the change in Gibbs free energy, calculating cell voltages, activity coefficients for electrolytes, and Pourbaix diagrams. The latter is a good example for teaching students about corrosion and material selection to potentially prevent corrosion. It is important to note that Pourbaix diagrams are another type of phase diagram that can complement conventional phase diagrams based upon pressure and temperature that are taught in thermodynamics. A mass transfer course, which is part of the transport phenomena sequence, should
introduce Faraday’s law of electrolysis, ionic conductivity of an ideal electrolyte from a Nernst-Planck framework, limiting currents, and potentially the Cottrell equation. Mathias and co-workers illustrated nicely how the low limiting current density of lithium-air batteries (<10 mA cm-2) makes them prohibitive to be used in a vehicle application, as over 1000 square meters need to be packed into a module conducive for a vehicle.3 So although lithium-air can achieve energy density values that are much higher than today’s lithium-ion batteries, their limiting current needs substantial improvement to make them practical. Educating chemical engineers who are capable of these types of “back of the envelope” calculations is part of the goal of introducing electrochemical science and engineering into the chemical engineering curriculum. A reaction kinetics course would present Butler-Volmer kinetics, and its simplified version, the Tafel equation, and Tafel plots. Students should feel confident in converting net rate laws to current density expressions. Prescribing fundamental electrochemistry content for the final theory course, process control, is difficult, but the course is amenable for showing how process control strategies can benefit electrochemical processes integrated with intermittent energy sources. For example, energy extracted from solar and wind can rapidly diminish if a passing storm blocks sunlight or the wind dials down. The drop in these renewable energy sources can be problematic for meeting energy demand. Control systems that deal with these random events and rapidly ramp up electrochemical energy conversion technologies to satisfy energy demands is vital for further penetration of solar and wind as our society’s energy sources. Process control also could benefit electrosynthesis processes that may have varying feedstock compositions or environmental factors (e.g., outside temperature).
With respect to the capstone courses in chemical engineering, the unit operations laboratory affords undergraduate chemical engineering students hands-on experience collecting current and/or voltage data points of operating electrochemical cells and relating these values to the production rate or separation rate of chemicals and expended energy for making the chemicals or performing the separation. It is important in engineering education to balance theory courses with hands-on, experiential learning. The electrochemical engineering elective I have taught over the past six years to senior undergraduate and graduate students has included an experimental project.
Students are assigned into teams of three to four people and perform experiments over two to three class periods. Previous experimental modules include a low-temperature proton exchange membrane (PEM) fuel cell, an ammonia-copper redox flow battery, a membrane capacitive deionization unit, and a rotating disk electrode for the oxygen reduction reaction. These experimental modules also ask the students to use electrochemical impedance spectroscopy to diagnose ohmic, charge-transfer, and diffusion-related resistance values within the cell. The laboratory assignment requires a written report and oral presentation and requires students to apply electrochemistry theory to interpret and analyze experimental results. As part of my NSF CAREER project, I plan to develop an electrochemical hydrogen pump for the unit operations laboratory so that a large contingent of graduating chemical engineering seniors can experience working with an electrochemical system. Electrochemical hydrogen pumps are captivating because they perform gas compression without any moving parts.4 They are also effective for purifying hydrogen from steam–methane reforming (SMR) effluents and could be a key technology in deriving blue hydrogen.5 Finally, a senior process design course should task students to realize a chemical process that integrates more than one electrochemical unit. One example could entail the use of electrodialysis for deionizing brackish water to be fed into a PEM water electrolyzer for green hydrogen production. This hydrogen can then be used in downstream processes to produce ammonia via Haber-Bosch or steel manufacturing. A technoeconomic analysis assignment can be devised to compare that process against a chloro-alkali electrolyzer that produces a caustic solution stream (e.g., NaOHaq) that is subsequently fed into an alkaline water electrolyzer for green hydrogen production.
One problem with integrating electrochemical processes in senior design courses is the lack of standard electrochemical units in process simulators such as Aspen HYSYS. The Aspen Custom Modeler can allow more advanced users to develop electrolysis units to be deployed in process flows.6 Making electrolyzers (e.g., water, carbon dioxide, carbon monoxide, and chlor-alkali), electrochemical pumps (hydrogen and CO2), and electrodialysis units standard modules in commercial process simulator software packages will help produce the next generation of graduates who are adept in designing more sustainable chemical processes that use electrochemical units.
In closing, The Electrochemical Society is at the forefront of the science and technology that is critical to addressing climate disruption. The race to decarbonize requires an army of college graduates with a basic proficiency in electrochemistry. In 2018, there were fewer than 1,100 doctoral degrees awarded in chemical engineering in the United States while there were over 11,000 degrees conferred for a bachelor’s degree in chemical engineering in the United States. Of the individuals with an advanced degree in chemical engineering, a small subset of them have been exposed to electrochemical science and engineering. Imagine if we could increase the number of chemical engineering graduates who are knowledgeable about electrochemistry by 10x. Such a substantial change is possible by adopting the core tenets of electrochemical science and engineering in the chemical engineering curriculum.
C. G. Arges acknowledges support from the National Science Foundation (Award # 2143056) for this work. I appreciate Professor Mike Janik at Penn State for our thoughtful discussions on this topic.
© The Electrochemical Society. DOI: 10.1149/2.F05223IF
Christopher G. Arges, Associate Professor of Chemical Engineering, Institutes of Energy and the Environment, Pennsylvania State University
Education: BS (University of Illinois at Urbana-Champaign), MS (NC State), and PhD (Illinois Institute of Technology) in Chemical Engineering; Postdoc, Pritzker School of Molecular Engineering. Research Interests: Polymer electrolyte
membranes, block copolymer self-assembly, separations, fuel cells. Work Experience: Associate Professor in Chemical Engineering at Penn State since August 2021, Assistant Professor in Chemical Engineering at Louisiana State University (2016 to 2021).
Pubs + Patents: 55 peer-reviewed publications, h-index 26, 45 invited talks, 3 ECS Interface articles, 6 papers in Journal of the Electrochemical Society
Honors & Awards: NSF Faculty Early Career Development Program (CAREER), ECS Toyota Young Investigator Fellowship, 3M Non-Tenured Faculty Award.
Work with ECS: 12 years of ECS membership, Chair of IE&EE Student Affairs Committee.
Website: https://sites.psu.edu/arges/, https://www.ionomersolutions.com https://orcid.org/0000-0003-1703-8323
1. S. B. Darling and D. L. Sisterson, How to Change Minds About Our Changing Climate, The Experiment, New York (2014).
2. J. Ewing and I. Penn, The New York Times (2021). https:// www.nytimes.com/2021/02/16/business/energy-environment/ electric-car-batteries-investment.html
3. F. T. Wagner, B. Lakshmanan, and M. F. Mathias, J Phys Chem Lett, 1, 2204–2219 (2010)
4. K. Fishel, G. Qian, G. Eisman, and B.C. Benicewicz, in High Temperature Polymer Electrolyte Membrane Fuel Cells, Q. Li, Editor, p. 527–540, Springer International Publishing (2016).
5. G. Venugopalan, et al., ACS Energy Lett, 7, 1322–13297 (2022).
6. M. Sánchez, E. Amores, D. Abad, L. Rodríguez, and C. Clemente-Jul, Int J Hydrog Energy, 45, 3916–3929 (2020).
The polysulfide shuttle effect is one of the major challenges to be overcome before widespread commercialization of Li–S batteries can be realized. Despite decades of research, the fundamental understanding of lithium polysulfide reaction pathways and their interaction with the cathode surface are still not well established. To this end, researchers from Wayne State University have reported on the use of in-situ atomic force microscopy–based scanning electrochemical microscopy (AFMSECM) coupled with Raman spectroscopy at spatiotemporal resolution to provide nanoscale–resolved information regarding polysulfide reaction pathways in Li–S batteries. This study indicates that the Li2S2 intermediate species forms readily on carbon surfaces but is less likely to form on polar host substrates such as nickel. The authors suggest that Li2S4 reduction reactions lead to the deposition of Li2S2 in addition to Li2S on carbon, whereas the reduction of Li2S4 leads to direct Li2S deposition on Ni surfaces. The increased understanding of fundamental Li–S battery redox processes presented in this work will help researchers to consider and identify sulfur host materials that can alleviate polysulfide shuttling issues in future studies.
From: N. K. Thangavel, K. Mahankali, and L. M. R. Arava1, J. Electrochem. Soc., 169, 060501 (2022).
Prediction of the corrosion rate of buried carbon steel pipelines and canisters is of utmost importance to many applications, such as the production and distribution of oil and gas and nuclear fuel storage. Calculation of a corrosion rate in soil is not always straightforward due to complex environments, with many factors influencing the corrosion rate in soil: temperature, pH, chloride, moisture, etc. The effectiveness of conventional statistical methods for prediction of corrosion rate is limited by the large amount of experimental data needed. Other applications have similarly complicated environments for prediction of corrosion rates, and more broadly there has been significant research into applying machine learning (ML) approaches to this challenging problem. In this paper, researchers at Sungkyunkwan University investigated two approaches—a statistical method, response surface methodology (RSM), and an ML method, artificial neural networks (ANN)—for prediction of corrosion rates of carbon steel in soil considering the influence of three environmental factors, temperature, pH, and chloride concentration. For this specific use
case, they found that both methods showed promise: ANN, for its intrinsic ability to capture and process non-linearity in the data, and RSM, for its explainability of the prediction.
From: N.T. Chung, S.-R. Choi, and J.-G. Kim, J. Electrochem. Soc., 169, 051503 (2022).
Lithium metal anodes with good cycle life are desired to increase the energy density of Li batteries. However, dendrite formation is a persistent problem for their development. This is because dendrites can cause short circuits, but also the fresh Li surface area of dendrites results in excessive solid electrolyte interphase (SEI) formation, consuming materials in the cell. A multi-institution team of researchers in China have developed a non-linear phase-field model with ButlerVolmer kinetics to understand dendritic growth pattern selection and to clarify the electrolyte composition near growing dendrites. In a phase-field model, an order parameter is used to identify which phase is present at any spatial point—for example the Li metal electrode or the liquid electrolyte. This simplifies the work of tracking a moving interface and lends itself to the case of dendritic growth. The researchers studied the evolution of a Li interface using two types of surface perturbation: a sinusoidal interface and a planar surface with artificial noise. They observed that enrichment of the cation concentration occurred at dendrite tips due to electromigration, and that an increase in the applied electric potential gradient increased the growth velocity of the leading tip.
From: H. Jing, H. Xing, et al., J. Electrochem. Soc., 169, 032511 (2022).
Excess illicit drug consumption or dependency can detrimentally impact the user’s mental wellbeing and overall health, as well as the wellbeing of others. Methamphetamine is the most manufactured amphetamine-type stimulant due to its relatively simple synthesis process. Chromatographic techniques are commonly used in forensic analysis of methamphetamine. In a recent report, researchers from the Universiti Sains Malaysia and the Prince of Songkla University of Thailand investigated electrochemical techniques as alternative methods for methamphetamine analysis. With differential pulse voltammetry, glassy carbon electrode modified with graphene oxide (GO) was found to have the highest sensitivity in methamphetamine direct oxidation. Under optimized GO loading, buffer and pH, and other analytical conditions, this method showed a bilinear calibration curve in the concentration ranges
of 1−40 μM and 40−120 μM, respectively, with good precision and accuracy. The limit of detection was determined to be 0.3 μM
Under a controlled lab setting, residual methamphetamine on four household surfaces, glass, stainless steel, plastic, and varnished wood, was successfully determined with recoveries greater than 82%. These results demonstrated the potential and warrant further development of this electrochemical technique as a rapid, on-the-spot drug detection tool.
From: K. Lee, K. Saisahas, A. Soleh, et al., J. Electrochem. Soc., 169, 056514 (2022).
Porous Hard Carbon as High-Performance Electrode Material for Supercapacitors: Towards Sustainable Approach
Electrochemically accessible high surface area carbon is critical for improving the energy density of supercapacitors and batteries. With the emphasis on using circular economy principles in synthesizing materials, focus has turned toward biomaterials as the source for high surface area carbons. A research team from the Hindustan Institute of Technology and Science in Chennai, India and Dongguk University in the Republic of Korea have been successful in using coco peat as the source for creating porous hard carbon. The team followed the approach of alkali treatment at high temperature to carbonize the biomaterial. The team carried out detailed morphological characterization using SEM, HRTEM, and BET. This confirmed the presence of meso and micro pores, with a resulting BET area of 838 m2/g. FTIR and Raman spectroscopy were used to understand the surface chemistry, and these indicated the presence of oxygenated surface-active groups. The resulting nonFaradaic and Faradaic capacitance was quantified through cyclic voltammetry. Analysis of the scan rate dependence of the peak current helped confirm the surfacebound nature of the electroactive species. The researchers carried out capacitive cycling studies and found a capacity loss of 4% with 5000 cycles.
From: V. Ragupathi, R. Praneash KB, et al., ECS J. Solid State Sci. Technol., 11, 041010 (2022).
Tech Highlights was prepared by Joshua Gallaway of Northeastern University, Mara Schindelholz of Sandia National Laboratories, David McNulty of University of Limerick, Zenghe Liu of Abbott Diabetes Care, Chock Karuppaiah of Vetri Labs, and Donald Pile of EnPower, Inc. Each article highlighted here is available free online. Go to the online version of Tech Highlights in each issue of Interface, and click on the article summary to take you to the full-text version of the article.
Lithium-ion batteries (LIBs) have become ubiquitous; many of us regularly carry more than three to power our personal electronic devices. The history of the development of LIBs has been covered in other reviews, and there are regular updates on the technology and science of the individual components of LIBs. While portable electronics are perhaps the most widespread application of LIBs, the weight and volume advantages of this battery technology have enabled many other technologies that impact our lives.
This issue of Interface features three examples of technologies that have or will impact our lives that have relied on the advances in battery technology provided by LIBs. The three articles each describe broad areas that have been impacted by the advantages of LIBs. These topics are human health, traction, and aviation. Each of these areas have and will impact our lives, and there are multiple examples where LIBs have enabled the application or process that is highlighted.
The first article, written by Esther Takeuchi, David Bock, Lisa Housel, Amy Marschilok, and Kenneth Takeuchi, describes impacts of lithium-based battery technologies on human health. Biomedical devices can provide health benefits to patients; however, these devices must be provided with power. This contribution covers the history and the chemistry that have enabled the power and the use of multiple biomedical devices, including pacemakers and defibrillators. The weight and volume advantages of these batteries have in some cases been necessary for the clinical adoption of the health technology, and in others have dramatically reduced patient impacts (e.g., via lower weight or smaller incisions). Where rechargeability of the device is desired, the volume, weight, and charging time advantages of LIBs have made and will continue to make them an attractive technology for applications in human health.
The second article, written by Dennis Corrigan, focuses on electric vehicles and traction batteries. Electric vehicles (EVs) are not a new concept, having been first introduced over 100 years ago. This article describes some of the history and motivation of EV battery development. Various rechargeable battery technologies have been demonstrated in traction vehicles over time; however, the energy and power density advantages of LIBs have provided performance options that are not available with previous battery technologies. Thus, LIBs have become the current state-of-the-art for traction applications. Corrigan also details the present status and requirements for EV batteries. He concludes with a discussion of the future prospects and chemistry of EV batteries.
The third article, written by Thomas Barrera, James Bond, Marty Bradley, Rob Gitzendanner, Eric Darcy, Michael Armstrong, and Chao-Yang Wang, covers LIB impacts in aviation. Aviation has even more sensitivity to weight than traction batteries, with size, weight, and safety critical for incorporating new aviation technologies. LIBs have already been used to power electronics in aircraft and are expected to even replace conventional jet fuels as the main power source for aviation. This article highlights upcoming levels of electrification for aircraft, and the battery performance considerations in meeting these applications. As safety is especially critical in aviation, safety requirements and the safe design of aviation LIBs are also discussed. LIBs are expected to contribute to future opportunities in the electrification of aviation.
There are many other applications and technologies with which we interact that are or will be enabled by LIBs. The exceptional volumetric and gravimetric energy density of this technology makes it attractive for numerous applications, and improvements in the LIBs will likely see them further adopted into more and more use cases. Now it is 30 years since the commercial introduction of LIBs, and their production and use is not only increasing but is accelerating. The next 30 years will likely see even more new technologies which impact our lives and are enabled by future generations and improvements in battery technologies.
© The Electrochemical Society. DOI: 10.1149/2.F07223IF
Gary M. Koenig Jr., Associate Professor of Chemical Engineering, University of Virginia
Education: BS (The Ohio State University) and PhD (University of Wisconsin-Madison) in chemical engineering.
Research Interests: Include synthesis and processing of energy storage materials, batteries for transportation and stationary applications, electrochemical phenomena and systems, and materials characterization.
Work Experience: Postdoctoral researcher in the Electrochemical Energy Storage Group at Argonne National Laboratory, and subsequently initiated a research group as a professor at University of Virginia.
Honors and Awards: Fulbright Research Fellow, Jefferson Scholars Foundation Hartfield Excellence in Teaching Award, University of Virginia Alumni Board of Trustees Teaching Award, NSF CAREER Award
Website: https://engineering.virginia.edu/faculty/gary-koenig https://orcid.org/0000-0002-7172-7819
Nian Liu, Assistant Professor of Chemical and Biomolecular Engineering, Georgia Tech
Education: BS (Fudan University), PhD (Stanford University).
Research Interests: The Liu Laboratory at Georgia Tech is broadly interested in the development of novel materials, devices, and methodologies for electrochemical applications that address global energy challenges.
Pubs + Patents: >60 publications that have been cited over 26,000 times, with an h-index of 60; Web of Science™ highly cited researcher.
Honors and Awards: Recipient of the ECS Daniel Cubicciotti Award, American Chemical Society (ACS) Division of Inorganic Chemistry Young Investigator Award, Chemistry of Materials Up-and-Coming Researcher, and Jim Pope CREATE-X Faculty Fellowship.
Website: https://liu.chbe.gatech.edu/ https://orcid.org/0000-0002-5966-0244
The ECS News homepage is your source for ECS, community, and industry news. Get an overview of everything going on—all in one place.
Follow the ECS News publications category to stay on top of announcements like Calls for Papers, recently published focus issues, technical editor job openings, ECS journal updates, and much more!
Webinars reach the global science community. Connect with community and be up-todate on your field’s latest trends via webinar announcements like the ongoing ECS Webinar Series.
Securing a fellowship or award offers monetary for pursuits. Don’t miss these Be informed on upcoming deadlines and the latest and their work here!
There’s a lot going on in the meetings world, from calls for papers and presentations at upcoming ECS meetings, to grants to facilitate travel to ECS meetings, sponsorship and vendor opportunities, and other exciting announcements. Stay ahead of the game with ECS News!
Take advantage of expert advice on the next steps in your career. Build success by using all the ECS Career Center resources. Job posters increase their postings’ exposure here. Check regularly!
Batteries have served as critical power sources for medical equipment and devices for over a century.1 Their advancement through new chemistry development and creative design has enabled breakthrough therapies, treatments, and diagnostics for a variety of medical conditions. The advent of battery-powered implantable devices ushered in an entirely new way to treat disease where the therapy is delivered by the device over long periods of time, in many cases over years, to treat a wide variety of medical conditions (Fig. 1). Batteries power state-of-the-art implantable pulse generators to address cardiac arrhythmias and to serve as neurostimulators for numerous applications, including deep brain stimulation treatments for disorders such as Parkinson’s disease, tremor, chronic pain, and seizures.2-4 The implantable cardiac pacemaker, defibrillator, and resynchronization devices enabled by new battery technologies revolutionized cardiac health care for patients, providing a treatment for heart disease and sudden cardiac death due to ventricular fibrillation.5,6 Small, implantable battery-powered pumps are used for controlled delivery of pain medication directly to the spinal cord through intrathecal drug delivery devices (pain pumps) for patients with chronic pain conditions.7 The impact of battery-powered implantable devices on human health is invaluable.
While the power demands, size, capacity, and configuration of the batteries vary based on the type of device and the therapy, there are common requirements for implantable batteries that aid human health. The batteries must operate effectively over many years to minimize surgical frequency, be safe during installation and use, and have predictable and reliable performance, low self-discharge, and high volumetric energy density for device miniaturization to maximize patient comfort. Thus, long-term stability during use, predictable performance, high volumetric energy density, and outstanding reliability are key characteristics that define successful systems for biomedical implants. In this review, the chemistries that currently power a variety of medical implantable devices are described. New frontiers and trends in battery research hold promise for improving patient care through use in implantable medical devices.
A brief history of the cardiac pacemaker is instructive, as that device provided the path for a wide variety of implantable devices for the treatment and management of human disease.8 The recognition that living tissue responds to electrical stimulation dates to the time of Galvani in the 1700s. Measurement of cardiac electrical signals and the development of the electrocardiograph took place in the 1800s. External cardiac pacemakers were first introduced in the early 1950s; the devices were large boxes filled with vacuum tubes that tethered the patient to a bed or a large cart with mobility confined to the length of an extension cord. The late 1950s and early 1960s are referred to as the “golden years” in the field of cardiac pacing. Remarkably, during this time, there were several important achievements in the field by multiple researcher teams working in different parts of the world. There are three achievements that particularly stand out: the demonstration of the first battery-operated wearable pacemaker (1957), surgical installation of the first totally implantable pacemaker (1958), and the first long-term correction of heart block with a selfcontained, implantable pacemaker (1960). These milestones opened the field for medical pacemakers and more broadly for implantable medical devices in general.
A key enabling technology for fully implantable electronic devices is the battery. The early batteries for the cardiac pacemaker were based on zinc/mercuric oxide primary cells or nickel cadmium rechargeable batteries.9 Mercuric oxide batteries avoided the need for the patient to charge the battery; but the batteries and the device were not fully sealed to allow any gas generated to escape. In contrast, the nickel cadmium battery was rechargeable, and the device could be sealed. However, the frequent need to charge the battery required patient diligence and was a constant reminder of their medical condition. In the early 1970s the introduction of lithium metal anode primary batteries for use in cardiac pacemakers was another significant milestone where the devices could last for years without the need for patient or physician intervention.
Over the next several years a number of lithium metal anode primary batteries were used in medical devices.9 These included solid cathode liquid organic electrolyte systems as well as liquid catholyte systems based on inorganic materials. Examples of the solid cathodes that were used include silver chromate, cupric sulfide, and vanadium pentoxide. The dominant liquid cathode of the time used thionyl chloride and a modification of that system employed a mixture of bromine and chlorine gas. Featured here are descriptions of the lithium-based systems that currently remain in widespread use.
In 1972 an implantable pacemaker powered by a lithium/iodine battery was placed in a patient; thus 2022 marks the 50th anniversary of the first implant of a lithium anode battery in a human.10 The lithium/iodine-polyvinylpyridine (PVP) battery was also patented 50 years ago for powering implantable cardiac pacemakers with power requirements in the microwatt range.11, 12 Since that time, it has remained a dominant battery for pacemakers due to its high safety, capacity, and reliability.13
The battery has an open circuit potential of 2.8 V and the overall discharge reaction is:9
Fig. 1. Implantable medical device applications each addressed by an implantable battery pulse generator shown schematically in gray.Theoretical Energy (high rate[26] 3.2[26]
The cathode in the cell consists of a conductive charge transfer complex formed via the reaction of iodine and poly-2-vinylpyridine (PVP). When the cathode material is added to the cell during fabrication, a thin layer of resistive LiI forms at the lithium anode surface, which serves as both the separator and the electrolyte.13 As the discharge reaction progresses, the LiI layer increases in thickness, increasing the cell impedance, but the cell is still capable of delivering current in the microampere range. The increasing resistance is in one sense advantageous as it results in a decrease in the loaded cell voltage, acting as a state-of-charge indicator for when the battery needs to replaced.9 A major benefit of the system is the high volumetric capacity of the cathode material (1041 mAh/cm3 , Table I), which enables a small battery size that is advantageous for implantation.
Because of the high energy density and low cost of the Li/I2 redox couple, as well as the stability of the solid state electrolyte interface, recent research has sought to adapt the chemistry for secondary batteries.14-19 The primary limitations for a rechargeable system are the sluggish reaction kinetics for the LiI to I2 conversion and the high impedance of the solid state electrolyte. Despite these challenges, self-forming Li/I2 batteries have recently been reported using 1:2 lithium iodide:3-hydroxypropionitrile (LiI(HPN)2) as a conductive additive. The studies demonstrate that the lithium anode and the iodine cathode can be generated in situ upon initial charging.20 Notably, the rechargeable system also demonstrates self-healing, where internal shorts are repaired during repeated cycling.21 Li/I2 batteries with liquid organic electrolyte are also an active area of research.22-25
An alternative battery for applications that require a mediumrate power source is an Li/CFx system. Use of CFx as a cathode material was first developed in Japan in the 1970s.27-28 CFx is the
notation for polycarbon monofluoride, where the carbon:fluorine ratio can be varied. Increasing the fluorine content improves the theoretical capacity of the material but diminishes the electrical conductivity, with C-F bonds having a more covalent nature as the C:F ratio increases.29,30 Values of x are typically around 1;31 at this stoichiometry the structure consists of fluorine atoms located between carbon layers, resulting in a CFCF stacking sequence. The material is prepared by direct fluorination of carbon sources such as graphite, petroleum coke, or carbon fibers. The overall discharge reaction is:
Li + CFx LiF + C (2)
The redox reaction progresses via insertion of solvated Li+ ions into the layered structure, forming an intermediate phase of lithium fluoride, solvent molecules, and graphite, which eventually decomposes into LiF and C.32 CFx has a remarkably high theoretical energy density of 2595 mWh/g when x=1, with a nominal cell potential of 2.8 V. Because the material is an insulator, the addition of carbon black additive to the cathode is required. The poor electrical conductivity is a shortcoming of the battery system, as well as only moderate rate capability and evidence of voltage delay during initial discharge.33
Recent work on Li/CFx batteries has probed the influence of electrolyte. It was demonstrated that the discharge voltage plateau could be elevated by incorporation of a high donor number solvent, which was hypothesized to decrease the energy barrier for breaking C-F bonds and thus decrease the reaction overpotential. Another recent report systematically studied the Li-salt concentration, the anion, and the solvent type on the operational voltage of Li/CFx batteries.34 Because the discharge proceeds via insertion of solvated Li+ ions, the electrolyte is a participating species in the electrochemical reaction and its properties impact the thermodynamic potential of the reaction. I. Characteristics
Lower Li-salt concentration and solvents with higher donor numbers offer a higher operational voltage (Fig. 2). The study provides new opportunities for improving the energy density of Li/CFx batteries via rational design of the electrolyte.
The discharge mechanism has also been revisited. Density functional theory calculations were used to probe the significant difference between the observed discharge voltage (2.5–2.8 V) and the theoretical voltage (4.5 V).35 The calculations demonstrated that a CFx-edge propagation discharge mechanism based on lithium insertion at the CF/C boundary resulted in an operation voltage which was consistent with the experimentally observed values. A multiscale investigation of the CFx discharge mechanism has also been conducted using an array of spectroscopy and microscopy techniques.36 It was found that Li starts to react to form LiF in the first 20% of the discharge, which continued to occur along with solvent insertion at a high depth of discharge (DOD). Notably, no lithium deposition or intercalation was observed throughout the complete reaction. These new findings may play an important role in guiding future work to improve the energy density and versatility of the cathode.
The power density of CFx has also been recently improved via engineering of three-dimensional porous fluorinated graphene microspheres which have significantly higher conductivity than conventional cathode designs.37 Remarkably, the material demonstrated a power density of >71,000 W/kg at a 50C rate, while maintaining a high energy density of 830 Wh/kg. These results pave a path forward for the potential use of CFx in high-rate implantable applications.
Batteries for implantable cardioverter defibrillators (ICDs) demand high power capability to provide rapid charging of capacitors that apply high energy shocks directly to the heart to interrupt ventricular fibrillation. Typically the battery must be able to deliver current pulses in the 2–3 A range in order to rapidly charge the device’s capacitors.38 Beyond the high power requirement, the battery must also be able to supply a lower current drain to power the heart-monitoring circuitry, must be of suitable size for implantation, and benefits from a voltage profile that enables a state-of-charge indication for determining the end of life (EOL) of the battery. The lithium/silver vanadium oxide (Li/SVO) battery, first implemented in the 1980s, is able to meet this set of requirements and still powers many ICDs today.
The SVO cathode material is prepared via a solid-state synthesis method and has a stoichiometry of Ag2V4O11 39,40 The overall discharge reaction is:
(3)
Through the addition of six moles of lithium, the material has a high theoretical specific capacity of 315 mAh/g. Besides the high capacity of the material, the structure and unique progression of the discharge mechanism are critical for its desirable electrochemical behavior. The material consists of edge- and corner-sharing vanadium oxide octahedra which comprise V4O11 slabs with silver ions between the layers, where the layered structure facilitates fast intercalation of Li+ ions during high-rate charging. As the material is initially discharged (<30% DOD), Ag+ ions are reduced to form Ag metal which significantly improves the electrical conductivity of the cathode and facilitates high-rate capability.41,42 From 30–50% DOD, the discharge process is dominated by reduction of V5+ to V4+, while at >50% DOD, indication of V4+ to V3+ reduction occurs. The multiple stages of silver and vanadium reduction result in a predictable stepped voltage profile useful for EOL indication.
In the decades since its initial implementation, multiple improvements to the system have further enhanced the battery for ICD applications, including cell design,43-35 optimization of the active cathode material,46,47 and modification of the electrolyte.48 An unpredictable life-limiting mechanism for the Li/SVO battery is the dissolution of the cathode in the electrolyte, which results in
deposits on the anode and an increase in the battery resistance. The silver vanadium phosphorous oxide (AgwVxPyOz) family of materials has been investigated and found to exhibit electrochemistry suitable for ICD applications while exhibiting reduced solubility compared to SVO.49-51
Notably, hybrid systems have been introduced where SVO and CFx are combined. The hybrid cathode utilizes the benefits of the SVO high rate and stepped voltage profile and the high capacity of CFx. A mixed version of the cathode is used in a device the size of a capsule which is ~8% of the volume of a traditional pacemaker.52 This new form factor allows the device to be implanted within the heart, eliminating the need for a subcutaneous pocket in the patient’s chest and the placement of leads to the heart, and reducing potential implant complications.52 A layered version of the SVO/CFx cathode has also been introduced for pacemakers as well as for implantable cardiac defibrillators.53 In this design, the SVO and CFx are placed on either side of a grid, allowing independent tuning of the SVO and CFx content.
Another battery chemistry for implantable medium- to high-rate applications is the manganese dioxide system, first commercialized in the 1970s.54,55 The battery has a high operating potential of ~3.0 V and a specific energy density of 230–270 mWh/g, depending on the discharge rate.26 The material also has a low rate of self-discharge, making it advantageous for implantable applications.9 The discharge reaction is:
(3)
In commercial systems, heat-treated electrochemically prepared MnO2 is used as the cathode, which contains 1x1 and 2x2 tunnels formed by edge-sharing MnO6 polyhedra as Li-ion intercalation pathways.56 Optimization of the heat treatment environment and atmosphere can be used to maximize the capacity retention.57
Several studies have probed the discharge mechanism and discovered that there are three stages of lithium intercalation into the material.58-60 Although the discharge profile of the battery is generally flat, the addition of chromium oxide and lead oxide additives can modulate the voltage profile to improve the EOL indication.61
An Li/MnO2 double-cell has also been described for use in highrate implantable cardiac defibrillator applications.61,62 In this design, two cells are connected in series from battery casing to battery casing to reduce volume while improving the high-rate performance. Recent work on the Li/MnO2 primary battery have focused on creating a hybrid battery through the addition of CFx to improve the specific energy of the system.63 The addition of CFx to MnO2 effectively suppressed self-discharge during storage at 45 °C.63
There is a subset of medical device implants with moderate to high power requirements that benefit from the use of rechargeable batteries to realize target lifetime and size. Secondary power sources for these devices satisfy the same overall requirements as primary batteries, including safety, reliability, high energy density, and low self-discharge. A notable example of devices that use secondary batteries are neurostimulators, as they often operate in the milliwatt power range where the use of a secondary battery allows a fully implantable device with acceptable lifetimes.
The introduction of lithium-ion rechargeable batteries has transformed portable electronics and has benefitted medical devices as well.64-68 Lithium-ion batteries initially used lithium cobalt oxide (LCO) cathode materials due to their high initial Coulombic efficiency and high cycle stability.69 Notably, higher energy density has been demonstrated through the use of layered metal oxides based on lithium nickel manganese cobalt oxide (LiNixMnyCozO2, NMC).70- 74 In these materials, the inclusion of a high Ni content increases both capacity
and operating voltage, Co functions to stabilize the layered structure for enhanced cycle life, and Mn improves the overall thermal and structural stability.72, 75-77 The significant research and development advances in lithium-ion rechargeable batteries are anticipated to continue to provide benefit for the medical device community.
There is a constant drive for medical devices to improve the patient experience by making them smaller and more reliable, with longer lifetimes. This set of needs has led to new research efforts in material, electrode, and cell designs in addition to development of predictive models to track power-source performance. The active research and development efforts of the battery community continue to lead to breakthroughs in the understanding and functional behavior of batteries for a variety of applications. The insights gained will provide benefit to future generations of batteries used by the medical community.
© The Electrochemical Society. DOI: 10.1149/2.F08223IF
Esther S. Takeuchi, SUNY Distinguished Professor and Knapp Chair in Energy and the Environment, Stony Brook University and Chair, Interdisciplinary Science, Brookhaven National Laboratory Education: BA in chemistry and history (University of Pennsylvania) and PhD in chemistry (The Ohio State University).
Research Interests: Electrochemical energy storage, Energy and the environment.
Work Experience: Director of the Institute for Electrochemical Energy Storage at Stony Brook University. Previously employed at Greatbatch Inc., her work was instrumental in the development of the battery for life-saving implantable cardiac defibrillators.
Patents: >150 patents.
Honors and Awards: Member of the National Academy of Engineering, the National Inventors Hall of Fame, and the American Academy of Arts and Sciences. Received the National Medal of Technology and Innovation, the European Inventor Award, and the NAS Chemical Sciences Award.
David Bock, Energy Storage Division of the Interdisciplinary Science Department at Brookhaven National Laboratory Education: BS (State University of New York at Geneseo) and PhD in chemistry (Stony Brook University).
Research Interests: Energy storage applications, including both primary lithium batteries and lithium-ion technology. Dr. Bock has recently been involved with the development of novel approaches for extreme fast charging and novel electrolytes, as well as the elucidation of battery degradation mechanisms. His research emphasizes using state-of-the-art tools, including operando X-ray characterization techniques, to provide mechanistic insight into electrochemical behavior.
Work Experience: Joined Brookhaven National Laboratory as a postdoctoral research associate before becoming a member of the scientific staff.
Pubs + Patents: >70 publications in the field.
Lisa M. Housel, Investigator, Materials Science Department, GSK Education: BS in Chemistry and Economics (Muhlenberg College) and PhD in Chemistry (Stony Brook University).
Research Interests: Understanding faradaic and degradation processes of (non)aqueous electrochemical energy storage systems using operando methodologies.
Work Experience: Assistant Scientist at Brookhaven National Laboratory and Affiliate Member of the Institute for Electrochemically Stored Energy.
Honors and Awards: 2020 ECS Energy Technology Division Graduate Student Award and 2021 NSLS-II Julian David Baumert PhD Thesis Award.
Amy Marschilok, Associate Professor of Chemistry and Co-Director of the Institute for Electrochemically Stored Energy at Stony Brook University.
Education: BA and PhD in chemistry (The University at Buffalo).
Research Interests: Electrochemistry as a diagnostic tool, operando investigations of batteries, and function of 2-dimensional materials.
Work Experience: Joint appointment at Brookhaven National Laboratory (BNL) as Manager of the Energy Storage and Energy Systems Divisions, and Deputy Director of the Center for Mesoscale Transport Properties, a DOE funded Energy Frontier Research Center.
Honors and Awards: Inaugural Cohort Member of the Oppenheimer Science and Energy Leadership Program, is a member of the User Executive Committee at NSLS-II, received the Woman of Distinction Award Education Category from the Girl Scouts, a Leadership Award from the YWCA, and the Visionary of the Year award while in industry.
Kenneth Takeuchi, SUNY Distinguished Teaching Professor at Stony Brook University and Scientist, Brookhaven National Laboratory Education: BS (University of Cincinnati) and PhD in chemistry (The Ohio State University).
Research Interests: The development of synthetic strategies yielding control and variation of crystallite size and nonstoichiometric chemical composition of inorganic materials and their utility towards energy storage.
Honors and Awards: Fellow, American Association for the Advancement of Science and the American Chemical Society; Responsible Care National Catalyst Award from the Chemical Manufacturers Association; NY regional recipient, U.S. Professor of the Year Award from the Carnegie Foundation for the Advancement of Teaching and the Council for Advancement and Support of Education (CASE); Stanley C. Israel Regional Award for Advancing Diversity in the Chemical Sciences; and SUNY Chancellor’s Award for Excellence in Teaching.
1. A. Wexler, J Hist Med Allied Sci, 72, 166–192 (2017).
2. A. H. Dabbour, et al., Front Neurosci, 15, 796203 (2021).
3. J. K. Krauss, et al., Nat Rev Neurol, 17, 75-87 (2021)
4. C. S. Louis, The New York Times (2014).
5. D. C. Bock, A. C. Marschilok, K. J. Takeuchi, and E. S. Takeuchi, Electrochim Acta, 84, (2012).
6. J. Gierula, M. F. Paton, and K. K. Witte, Future Cardiol, 17, 609-618 (2021)
7. R. Bolash, et al., Neuromodulation, 18, 150-156 (2015).
8. O. Aquilina, Images Paediatr Cardiol 8, 17-81 (2006)
9. B. B. Owens, Batteries for Implantable Biomedical Devices, Plenum Press, New York (1986).
10. Holmes, C. F., J Power Sources, 97/98, 739-741 (2001).
11. A. A. Schneider and J. R. Moser, US Patent 3,674,562 (1972).
12. Moser, J. R., US Patent 3,660,163A (1972).
13. R. J. Brodd, K. R. Bullock, R. A. Leising, R. L. Middaugh, J. R. Miller, and E. Takeuchi, J. Electrochem. Soc., 151, K1-K11 (2004).
14. W. Yourey, L. Weinstein, A. Halajko, and G. G. Amatucci, ECS Trans., 28, 159-165 (2010).
15. W. Yourey, L. Weinstein, A. Halajko, and G. G. Amatucci, Electrochim Acta, 66, 193-203 (2012).
16. L. Weinstein, W. Yourey, J. Gural, and G. Amatucci, JES, 155, A590-A598 (2020).
17. H. Wang, H. Li, B. Xue, Z. Wang, Q. Meng, and L. Chen, J. Am. Chem. Soc., 127, 6394-6401 (2005).
18. S. Shi, L. Xu, C. Ouyang, Z. Wang, and L. Chen, Ionics, 12, 343-347 (2006).
19. F.-C. Liu, Z. Shadike, F. Ding, L. Sang, and Z.-W. Fu, J Power Sources, 274, 280-285 (2015).
20. A. Abraham, M. R. Dunkin, J. Huang, B. Zhang, K. J. Takeuchi, E. S. Takeuchi, and A. C. Marschilok, MRS Commun, 9, 657662 (2019).
21. C. A. Stackhouse, et al., J. Electrochem. Soc., 168, 010519 (2021).
22. Z. Meng, et al., ACS Appl Mater Interfaces, 10, 17933-17941 (2018).
23. L. Qiao, C. Wang, and X. S. Zhao, ACS Appl Energy Mater, 41, 7012-7019 (2021).
24. P. Li, et al., Highly Thermally/Electrochemically Stable I-/I3Bonded Organic Salts with High I Content for Long-Life Li-I2 Batteries, Adv. Energy Mater, Ahead of Print.
25. Z. Cheng, et al., Nat. Commun, 13, 125 (2022).
26. D. Linden and T. B. Reddy, in Handbook of Batteries. D. Linden and T. B. Reddy, Editors, McGraw-Hill, New York (2002).
27. K. Watanabe and M. Fukuda, US Patent 3536532 (1970).
28. K. Watanabe and M. Fukuda, US Patent 3700502 (1972).
29. T. Nakajima, R. Hagiwara, K. Moriya, and N. Watanabe, J Electrochem Soc, 133, 1761-6 (1986).
30. T. Nakajima, A. Mabuchi, R. Hagiwara, N. Watanabe and F. Nakamura, J Electrochem. Soc 135, 273-277 (1988).
31. K. Guérin, et al., Chem Mater, 16, 1786-1792 (2004).
32. H. Touhara, H. Fujimoto, N. Watanabe and A. Tressaud, Solid State Ion, 14, 163-70 (1984).
33. S. S. Zhang, D. Foster, J. Wolfenstine, and J. Read, J Power Sources, 187, 233-237 (2009).
34. J. Jiang, et al., J. Power Sources, 527, 231193 (2022).
35. K. Leung, et al., Chem Mater, 33, 1760-1770 (2021).
36. B. Sayahpour, et al., Adv.Energy Mater, 12, 2103196 (2021).
37. Z. Luo, et al., ACS Appl Mater Interfaces, 13, 18809-18820 (2021).
38. E. S. Takeuchi and R. A. Leising, MRS Bull, 27, 624-627 (2002).
39. R. A. Leising and E. S. Takeuchi, Chem Mater, 6, 489-95 (1994).
40. R. A. Leising and E. S. Takeuchi, Chem Mater, 5, 738-42 (1993).
41. R. A. Leising, W. C. Thiebolt III and E. S. Takeuchi, Inorg Chem, 33, 5733-40 (1994).
42. E. S. Takeuchi and W. C. Thiebolt III, J. Electrochem Soc, 135, 2691-4 (1988).
43. E. S. Takeuchi, J Power Sources, 54, 115-119 (1995).
44. C. J. Post and E. S. Takeuchi, US Patent 5147737A (1992).
45. A. M. Crespi, S. K. Somdahl, C. L. Schmidt and P. M. Skarstad, J Power Sources, 96, 33-38 (2021).
46. X. Cao, L. Xie, H. Zhan, and Y. Zhou, Inorg, 44, 886-889 (2008).
47. E. S. Takeuchi, A. C. Marschilok, R. A. Leising and K. J. Takeuchi, J Power Sources, 174, 552-553 (2007).
48. H. Gan and E. S. Takeuchi, US Patent EP829911A2 (1998).
49. D. C. Bock, R. V. Tappero, K. J. Takeuchi, A. C. Marschilok and E. S. Takeuchi, ACS Appl Mater Interfaces, 7, 5429-5437 (2015).
50. D. C. Bock, A. C. Marschilok, K. J. Takeuchi and E. S. Takeuchi, J Power Sources, 231, 219-235 (2013).
51. D. C. Bock, K. J. Takeuchi, A. C. Marschilok and E. S. Takeuchi, Phys Chem Chem Phys, 17, 2034-2042 (2015).
52. P. Ritter, et al., EP Europace, 17, 807-813 (2015).
53. H. Gan, R. S. Rubino and E. S. Takeuchi, J Power Sources, 146, 101-106 (2005).
54. H. Ikeda, T. Saito and H. Tamura, Denki Kagaku Oyobi Kogyo Butsuri Kagaku, 45, 314-18 (1977).
55. K. Ikeda, M. Hara and K. Ikukawa, 1976-118327, 53042325, Japan (1978).
56. W. Bowden, et al., J Power Sources, 165, 609-615 (2007).
57. W. M. Dose and S. W. Donne, J Power Sources, 247, 852-857 (2013).
58. Y. Chang, et al., Electrochim Acta, 339, 135918 (2020).
59. J. C. Nardi, J Electrochem Soc, 132, 1787-1791 (1985).
60. T. Ohzuku, M. Kitagawa and T. Hirai, J. Electrochem Soc, 136, 3169-74 (1989).
61. J. Drews, R. Wolf, G. Fehrmann, and R. Staub, J Power Sources, 80, 107-111 (1999).
62. J. Drews, R. Wolf, G. Fehrmann, and R. Staub, J Power Sources, 65, 129-132 (1997).
63. Y. Chang, et al., Electrochim Acta, 320, 134618 (2019).
64. K. Mizushima, P. C. Jones, P. J. Wiseman, and J. B. Goodenough, Mater Res Bull, 15, 783-789 (1980).
65. J. Goodenough, K. Mizushima and T. Takeda, Jpn J Appl Phys, 19, 305-313 (1980).
66. A. Yoshino and K. Sanechika, Secondary Battery. Nov. 8, 1986.
67. A. Yoshino, K. Sanechika, and T. Nakajima, Secondary Battery. May 10, 1985.
68. A. Yoshino and K. Sanechika, K. Nonaqueous Secondary Battery. May 28, 1984.
69. L. Wang, B. Chen, J. Ma, G. Cui, and L. Chen, Chem Soc Rev, 47, 6505-6602 (2018).
70. F. Schipper, et al., J Electrochem Soc, 164, A6220-A6228 (2016).
71. C. Tian, et al., J Electrochem Soc, 165, A696-A704 (2018).
72. C. Tian, F. Lin and M. M. Doeff, Acc Chem Res, 51, 89-96 (2018).
73. M. D. Radin, et al., Adv Energy Mater, 7, 1602888 (2017).
74. D. Andre, et al., J Mater Chem A, 13, 6709-6732 (2015).
75. A. Manthiram, J. C. Knight, S.-T. Myung, S. Oh, and Y.-K. Sun, Adv Energy Mater, 6, 1501010 (2016).
76. S.-K. Jung, et al., Adv Energy Mater, 4, 1300787 (2014).
77. S.-M. Bak, et al., ACS Appl Mater Interfaces, 6, 22594-22601 (2014).
Electric vehicles (EVs) are not new; they date back to the 19th century, along with the invention of primary and secondary batteries and electric motors.1 In fact, batteries and EVs predate the invention of the internal combustion engine (ICE). Electric cars like the early EV shown in Fig. 1 were commonplace around 1900. They had a limited range on the order of 25 miles due to the modest 25 Wh/kg specific energy of rechargeable batteries in that era. However, EVs initially competed well commercially with ICE vehicles, partly because hand-cranked engines were inconvenient and unpopular. Ironically, it was the invention of the electric-starter motor powered by lead-acid batteries that enabled ICE vehicles to take over and dominate the automobile industry for the rest of the 20th century. The lead-acid battery business thrived with the massive growth of the world auto industry. Today, the lead-acid battery market has reached about $40B in revenue and continues to grow.2
In the last decades of the 20th century, there was renewed interest in battery-powered EVs due to resource issues with petroleum and environmental concerns about toxic air pollution and greenhouse gas emissions. The OPEC oil embargo in the 1970s and the California Zero Emission Vehicle (ZEV) mandate of 1990 were key drivers that motivated the development of battery EVs by automakers. A variety of higher energy–density battery technologies that were developed for EV applications are included in Table I. Despite impressive theoretical energy densities, most of these new technologies had serious practical deficiencies. Among the most promising was the nickel-metal hydride (NiMH) battery.3 It featured a specific energy of about 80 Wh/kg, more than twice the specific energy of modern lead-acid batteries with much improved specific power and cycle life. Utilizing the NiMH battery, General Motors (GM) developed the iconic EV1, a high-performance EV with a range over 100 miles that received considerable publicity and notoriety. However, EV1 production plans were scuttled with the cancellation of the ZEV mandate in 2002, which spawned various conspiracy theories, as highlighted in the film Who Killed the Electric Car?4 This movie included a mock trial of who was to blame. Lots of villains were named, but curiously the battery was declared “not guilty.” In fact, EVs were not commercialized at that time primarily because of deficiencies in batteries, especially their high cost.
The extensive development of EV prototypes in the 1990s resulted in a much more detailed understanding of the battery performance requirements for EVs.5 For example, it was learned that for modern EVs, the battery volumetric energy density is more important than the specific energy. The United States Advanced Battery Consortium (USABC), established in 1991 as a partnership of the “Big Three” US automakers that eventually included the US Department of Energy, developed detailed performance targets to guide development of advanced batteries aimed at commercialization of EVs.6 The 2020 update of the USABC requirements is summarized in Table II.
The USABC requirements envision a full battery pack system capable of providing power and energy for a commercially viable passenger car with a range of about 200 miles. With a vehicle energy consumption of about 225 Wh/mile, this would require a usable energy of 45 kWh. The battery also would need to provide about 100 kW of pulse discharge power to enable acceleration from 0 to 60 mph in 10 s or less. To utilize the efficiency advantage of regenerative braking, 50–100 kW of pulse charge power capability is also needed. As a practical matter, the battery pack must provide its power and energy at high voltage, within the voltage range of 220–420 V, for proper operation of practical electric motor drives. The power and energy are specified in the USABC requirements in terms of specific power (W/kg), power density (W/L), specific energy (Wh/kg), and volumetric energy density (Wh/L) derived from the EV power and energy requirements and the estimated mass and volume packaging constraints. To achieve the battery pack targets, the specific performance targeted for battery cells is higher. Taking into account an estimated 50% mass and volume packaging burden, battery cells at 350 Wh/kg and 750 Wh/L would be needed to achieve battery pack energy density targets of 235 Wh/kg and 500 Wh/L. EV commercialization also requires meeting life and cost targets. Consumers expect passenger vehicles to be capable of driving up to 150,000 miles over a 15-year lifespan. This requires the battery to be capable of providing 1000 full depth charge–discharge cycles that meet the rated energy and power requirements over a calendar life of 15 years. To ensure full performance capability over a 15year lifetime, the initial battery performance must exceed the power and energy requirements to accommodate degradation over time. Additionally, to be competitive with ICE vehicles, the electric power
Fig. 1. Early electric vehicle (left) typically with 20 mph top speed and 25 mile range and Tesla Model 3 EV (right) with 5-second acceleration to 60 mph and over 200-mile range. (Courtesy of National Museum of American History and Tesla Motors.)Practical Specific Energy (Wh/kg)
Practical Energy Density (Wh/L)
Lead-Acid 2.0 35 80 Low energy, short life
Nickel-Iron 1.2 45 55 Low energy, poor efficiency
Nickel-Cadmium 1.2 244 45 100 Low energy, toxic, high cost
Nickel-Zinc 1.6 372 60 110 Low energy, short life
Sodium-Sulfur 2.0 792 170 250 High temperature
Sodium-Nickel Chloride 2.6 787 125 190 High temperature
Zinc-Bromine 1.6 572 70 60 Toxic gases
Zinc-Chlorine 1.9 835 90 80 Toxic gases
Zinc-Air 1.3 1353 150 160 Low power, efficiency, life
Nickel-Metal Hydride 1.2 240 80 200 Low energy, cost
Pack Target
Cell Target
Discharge Power Density (W/L) 1000 1500
Specific Power (W/kg) 470 700
Specific Regen Power (W/kg) 200 300
Usable Energy Density (Wh/L) 500 750
Usable Specific Energy (Wh/kg) 235 350
Maximum Voltage (V) 420 Minimum Voltage (V) 220
Calendar Life (years) 15 15
Cycle Life (cycles) 1000 1000
Selling Price ($/kWh) 125 100
Pass Electrical Abuse Tests (overcharge, overdischarge, external shorting)
Pass Physical Abuse Tests (elevated temperature, water immersion, vibration, crush, drop, nail penetration)
train must be comparable in cost to the ICE drive train. The USABC targets a battery pack cost of about US$5600 or $125/kWh. No single battery technology has yet met all the requirements of Table II. The most difficult targets are energy density and cost. EV battery packs have not achieved an energy density of 235 Wh/kg and 500 Wh/L at a cost of $125/kWh. In addition to the EV performance, life, and cost targets, the most important requirement for EV batteries is safety.7 Safety is engineered at the vehicle, battery pack system, and battery cell level through detailed failure modes and effects analysis. The intrinsic safety of battery cells is confirmed through electrical and physical abuse testing included in Table II.
The development of high energy–density EV batteries has proven to be a very difficult problem for electrochemists. Moore’s Law exemplifies the dramatic exponential progress in semiconductor electronics, with a factor of two improvement every two years. Where is the Moore’s Law of batteries? Progress in battery energy
density has been slow by comparison, as shown in Fig. 2. It took over 100 years to achieve a single order of magnitude improvement in battery energy density. The breakthrough technology was the lithiumion (Li-ion) battery for which the 2019 Nobel Prize was awarded to ECS members Stan Whittingham, John Goodenough, and Akira Yoshino.8 The Li-ion battery was the enabling technology for many revolutionary portable electronic devices, such as cell phones and laptop computers.9,10 It is now also the enabling technology for the high-volume commercialization of EVs.10,11
Intercalation batteries such as nickel-metal hydride batteries and Li-ion batteries are particularly advantageous for EV applications. Their charge storage reactions involve simple ion insertion processes while avoiding complex chemistry and profound structural changes. This characteristic enables high utilization of active materials, high power capability, and long cycle life. Of the intercalation batteries, Li-ion batteries are superior to NiMH batteries for EV applications largely because of their three-fold higher cell voltage and comparable advantage in energy density. Li-ion batteries are highly superior to all the 20th century EV batteries in Table I, not just because of their superior energy density, but also other favorable characteristics that
include high power, high efficiency, and long life. Their gravimetric and volumetric energy density are approaching 300 Wh/kg and 750 Wh/L, respectively, and are continuing to improve, as shown in Fig. 2.
The Li-ion battery was first commercialized in the 1990s for portable electronics. However, there were early concerns about safety, with various incidents involving battery fires both in laptop computers and in EV prototypes. In place of a high heat–capacity aqueous electrolyte, they contain low heat–capacity electrolytes composed of flammable organic solvents. This focused strong attention on battery safety. Improved manufacturing and quality control with cleanroom manufacturing environments evolved to avoid premature failures from particulate shorts through thin separators. Advanced multilayer separators with protective coatings were also developed to improve safety and cycle life. Electronics technology was developed to balance the state-ofcharge in a battery pack to avoid the overcharge of individual cells. More thermally stable active materials were developed, including composite nickel-manganese-cobalt oxide and lithium iron phosphate cathode materials. Due to the development of alternative active materials, Liion batteries are not confined to one battery chemistry couple as are the other batteries in Table I. Liion battery technology is actually a family of battery chemistries with a variety of active materials.8-12 Cathode materials have evolved from the cobalt oxide cathodes initially commercialized to a wide variety of alternatives, some of which are illustrated in Fig. 3. Alternative anode materials include natural and synthetic graphite materials, hard carbons, lithium titanate, and silicon. It is the utilization of alternative higher energy materials as well as improved cell design and packaging into prismatic, cylindrical, and pouch cells that has enabled the continuous improvement in Li-ion energy density.9 Additionally, the development of improved cathode materials has also led to reduced costs through the reduction or elimination of cobalt, a highly expensive material with high cost volatility.
Li-ion battery technology can now meet most of the USABC targets for EV applications in Table II with the notable exceptions of specific energy, energy density, and cost. By 2020, there were Li-ion EV battery cells available with an energy density of
around 250 Wh/kg and 750 Wh/L, enabling production of EVs like the GM Chevy Bolt, Ford Mustang Mach-E, Fiat 500e, and the Tesla Model 3 (see Fig. 1) with excellent performance and a range of more than 200 miles. This range was achieved with 50 kWh battery packs that have a fully burdened specific energy of around 150 Wh/kg (40% less than the USABC target) by using clever engineering with tradeoffs in cost and vehicle features. Even higher range EVs have been developed with current battery technology. A long-range version of the Tesla Model 3 has been offered that uses an 80 kWh battery pack for a range of over 350 miles, albeit with a substantial cost premium. Over the past decade, there has also been a dramatic reduction in the cost of Li-ion batteries (see Fig. 4).2,13,14 Before 2025, battery cell and battery pack costs are projected to meet USABC targets of $100/kWh and $125/ kWh, respectively. EVs with a range of 200 miles were offered for a cost of under $40,000 in 2020. This was achieved with battery packs estimated to cost about $220/kWh, which translates to just over $10,000 for a 50 kWh battery pack using $150/kWh Liion cells.14
Ideally, we would like to package 100 kWh of battery on an EV to provide a range of 400 miles. Can battery electrochemistry advances yield the 400 Wh/kg needed to make that happen? Is there a higher energy–density successor to Li-ion batteries? Lithium and fluorine, sitting at the upper corners of the periodic table, could be combined electrochemically with a cell voltage of 5.88 V, yielding a battery theoretical specific energy of over 6000 Wh/kg, an order of magnitude higher than that of Li-ion batteries. However, given the extreme reactivity and safety issues with fluorine, this does not seem like a practical approach for now. Lithium-air (Li-air) batteries utilizing oxygen reactants rather than fluorine have been a focus of extensive R&D studies over the last decade.15 With a cell voltage of 3 V, these batteries have a very promising theoretical specific energy of about 2500 Wh/kg. However, Li-air batteries utilize fuel cell cathodes that burden them with additional cost, mass, volume, and complexity. They are also challenging to recharge and the energy efficiency on chargedischarge is low, on the order of 70% or less (Li-ion energy efficiency exceeds 90%). There are cycle life issues as well. Lithium-sulfur (Li-S) batteries have also received extensive R&D attention in the last decade.16 In addition to a theoretical specific energy of 2500 Wh/kg, they avoid the complications of a fuel cell cathode. In addition, Li-S batteries also have promise to be a lowcost technology because sulfur is a very inexpensive reactant. Prototype Li-S battery cells have been developed with a gravimetric energy density of about 500 Wh/kg, about double that of Liion batteries. However, the volumetric energy density does not show significant improvement over Li-ion batteries.
Furthermore, the complex sulfur reaction chemistry yields a multistep discharge curve around 2.2 V and poor charge-discharge energy efficiency as well as an inadequate cycle life. After a decade of intense R&D focus on these two promising battery couples, there is now skepticism about their practicality for EV applications in the short term.
Fig. Fig. 5. Global battery EV (BEV) and Plug-in hybrid EV (PHEV) sales and EV market share. (Courtesy of EV Volumes, reference 22.)Today, there is tremendous enthusiasm for solid state lithium batteries, as a most promising technology to succeed Li-ion batteries for EVs.17-19 This technology is very popular with the investment community seeking to invest in EV technology futures.17 Solid state batteries offer a pathway to replace graphite-based anodes with metallic lithium, with an order of magnitude higher specific capacity (mAh/g), leading to substantially higher gravimetric and volumetric energy densities. Theoretically, solid state batteries could reach 500 Wh/kg and 1000 Wh/L energy densities. Solid state batteries also have the potential to eliminate much of the fire safety issues of traditional Li-ion batteries based on flammable organic liquid electrolytes, which would be replaced with nonflammable solid ionic conductors. Additionally, higher temperature operation would be enabled, which could make battery pack thermal management systems smaller, simpler, and less expensive. While the potential benefits of solid state batteries are compelling, there are also R&D challenges that are somewhat daunting.17-19 Solid state ionic conductors are generally inadequate in ionic conductivity compared to existing liquid electrolytes used in Li-ion batteries. There are serious materials compatibility issues with existing solid state ionic conductors. There are issues related to interfacial contacts and dendrite penetration through phase boundaries. Manufacturing processes for electrode assemblies need extensive development. However, there is intense competition among dozens of well-funded R&D organizations to find the solutions to these problems to provide the next generation of batteries beyond Li-ion.17
While we continue to look for the “magic bullet” solution to achieve 500 Wh/kg energy density, the energy density of Li-ion batteries continues to increase and the cost continues to fall. Maybe the next-generation battery after Li-ion will actually be advanced Li-ion batteries. There is now intensive work on the development of Li-ion batteries utilizing dimensionally stable metallic lithium anodes with conventional Li-ion cathodes and liquid electrolytes that have the potential to push the specific energy to 500 Wh/kg this decade.20 This approach circumvents some of the key hurdles of solid state battery development, such as the low conductivity, materials incompatibility, and manufacturability issues associated with solid ionic conductors. There is a plethora of R&D approaches for higher energy density and reduced cost Li-ion batteries underway powered by massive industrial and government funding at battery companies, national labs, and universities. The Li-ion battery industry is a highly diverse international business with 2021 revenue exceeding $70B.2 Mostly spurred by demand from the rapidly growing EV industry, that revenue is expected to at least triple in the next decade.2
Li-ion batteries have stimulated the dramatic transformation now underway in the world auto industry.21,22 The EV market has grown by leaps and bounds over the last decade, as shown in Fig. 5. Plugin EVs (including mostly battery EVs but also plug-in hybrid EVs) captured over 8% of the global light–duty vehicle market in 2021. This a truly international transformation. In 2021, the EV market share was 3% in the United States, over 10% in China, and over 60% in Norway. Auto companies in the United States and Europe now talk about converting all small passenger cars to battery EVs. They claim cost parity for EVs with ICE vehicles is coming in the next several years when the cost of Li-ion battery packs is projected fall below $100/kWh. This seems a little curious since a 400-mile range vehicle requires a 100 kWh battery pack in a typical car. That would price the battery pack at $10,000, approximately double the cost of a typical ICE drive train. However, current EV offerings targeting a 200-mile range using 50 kWh battery packs could be cost competitive with ICE cars, albeit not with a range of 400 miles. EVs also have the additional value proposition of substantially lower energy costs over ICE vehicles. In the United States in 2022, the energy cost per mile to drive an EV with an energy consumption of 250 Wh/mile is less than 20% that of a 25-mpg ICE vehicle (using baseline electricity costs of 15 cents/kWh vs. $5/gallon gasoline). The consequent energy cost savings for the EV would be more than $15,000 over 100,000 miles of driving.
An intrinsic disadvantage of EVs historically has been the slow recharge of batteries in comparison to ICE vehicle refueling at the gas pump. Gas cars can be refueled in 5 minutes (at an energy transfer rate of several megawatts). Normal battery recharge takes hours or at least many minutes due to the rate limitations of battery cells. Li-ion batteries can be recharged to 80% in 20 minutes. But that takes a lot of electrical power. For a 50-kWh battery pack, it would take about 150 kW. Building fast-charge infrastructure will take a lot of time, effort, and expense. Yet, it is underway. In the United States, Tesla has an installed network of more than 2000 fast-charge stations with a charge capability of up to 250 kW. This makes long-distance EV travel feasible, if not as convenient as for ICE-powered cars. Major R&D efforts are now focused by the US DOE and auto companies on improving the capability of batteries to be charged at faster rates, and the development of infrastructure to provide higher charge power.23 Faster recharge will help reduce range anxiety for EV drivers and improve the commercial viability of 200-mile range vehicles.
Vehicle electrification, the transformation in the auto industry to electric and hybrid EVs, is now happening at a rapid pace. Liion batteries are enabling this dramatic transformation of the auto industry, and Li-ion batteries and their derivatives are likely to power the growing EV industry for decades to come.
© The Electrochemical Society. DOI: 10.1149/2.F09223IF
I thank Mark Verbrugge (GM), Ted Miller (Ford), Oliver Gross (Stellantis), Boryann Liaw (Idaho National Labs), and Mike Sanders (Avicenne) for helpful discussions.
Dennis Corrigan, Director of Vehicle Electrification Programs, Oakland University
Education: BS in Chemistry (Purdue University); PhD in Electrochemistry (University of Wisconsin).
Research Interests: The development of electrochemical power devices for hybrid and electric vehicles, including lead-acid, nickelzinc, nickel-metal hydride, and lithium-ion batteries as well as fuel cells and supercapacitors.
Work Experience: As Vice President of Ovonic Battery, he played a major role in the development of NiMH traction batteries for the EV1 electric vehicle. He was a founder of the electric drive vehicle education program at Wayne State University and developed core courses on electric and hybrid vehicles batteries. He has led research teams at GM R&D, ECD-Ovonics, Cobasys, US Army TARDEC, and XALT Energy.
Pubs + Patents: >170 presentations and publications and 19 US patents.
Work with ECS: Dennis has been a member of ECS since 1977 and has been very active in the Detroit local section of the ECS, serving three times as chair.
1. E. H. Wakefield, The History of the Electric Automobile, Society of Automotive Engineers, Warrendale PA (1994).
2. M. Sanders, “Market Report: Cell, Pack and Electrode Manufacturing in North America,” NAATBatt 2022 Annual Conference (February 2022).
3. S. R. Ovshinsky, M. A. Fetcenko, and J. Ross, Science, 260, 176–181 (1993).
4. Who Killed the Electric Car?, documentary film directed by Chris Paine, produced by Jessie Deeter, Sony Picture Classics (2006).
5. D. A. Corrigan and A. Masias, in Linden’s Handbook of Batteries, 5th Ed., K. W. Beard, Editor, Chap. 26A, McGraw Hill, New York (2019).
6. USABC Battery Test Manual for Electric Vehicles, Vehicle Technologies Program, US Department of Energy, Idaho National Laboratory Report INL/EXT-15-34184, Rev 3.1 (October 2020).
7. V. Ruiz, A. Pfrang, A. Kriston, N. Omar, P. Van den Bossche, and L. Boon-Brett, Renew Sust Energ Revs, 81, 1427–1452 (2018).
8. M. S. Whittingham, Chem Rev, 104, 4271–4301 (2004).
9. J. Dahn and G. M. Ehrlich, Linden’s Handbook of Batteries, 5th Ed., K. W. Beard, Editor, Chap. 17A, McGraw Hill, New York (2019).
10. G. E. Blomgren, J Electrochem Soc., 164, A5019 A5025 (2017)
11. M. Alamgir, IEEE Electrification Magazine, 5, (1), 43–52 (2017).
12. N. Nitta, F. Wu, J. T. Lee, and G. Yushin, Mater Today, 18, 252–264 (2015).
13. BloombergNEF 2022, https://about.bnef.com/blog/batterypack-prices-fall-to-an-average-of-132-kwh-but-risingcommodity-prices-start-to-bite/
14. D. Howell, Annual Merit Review, DOE Vehicle Technologies Office, Washington, DC (2017).
15. L. Grande, et al., Advanced Materials, 27, 784–800 (2015).
16. T. Li, et al., Adv Funct Mater, 29, 1901730 (2019).
17. A. Scott, Chemical & Engineering News, 99, (32), 20-22 (2021).
18. Y.-K. Sun, ACS Energy Lett, 5, 3221–3223 (2020).
19. K. Kerman, A. Luntz, V. Viswanathan, Y.-M. Chiang, and Z. Chen, J. Electrochem. Soc., 164, A1731–1744 (2017).
20. J. Liu, et al., Nature Energy, 4, 180 –186 (2019).
21. Argonne National Laboratory, Energy Systems Division, Light Duty Electric Drive Vehicles Monthly Sales Updates, https:// www.anl.gov/es/light-duty-electric-drive-vehicles-monthlysales-updates.
22. EV Volumes, https://www.ev-volumes.com/
23. E. J. Dufek, et al., J Power Sources, 526, 23112 (2022).
Electrochemistry and solid state science and technology are the keys to solving many global problems. ECS is committed to supporting its members to accelerate the speed of science. part of the solution; join ECS. Here’s what we offer
•
•
•
Traditionally, commercial, civil, and military aircraft have used rechargeable lead-acid and nickel-cadmium (Ni- Cd) batteries for main engine starting, auxiliary power units, communication, navigational, emergency, and other system power needs.1 Commercial non-rechargeable lithium battery technologies are also commonly used in commercial aircraft applications for main power sources or back-up and standby power devices requiring high specific energy, high volumetric energy density, and long shelf life.2 Throughout the 1990s significant increases in rechargeable Li-ion battery (LIB) technology readiness enabled early transitions from heritage Ni-Cd batteries to LIBs for advanced aircraft applications such as the B-2 bomber, Global Hawk surveillance unmanned aerial vehicle (UAV), and F-35 Joint Strike Fighter programs.3 Gains in aircraft fuel efficiency, lower emissions, and reduced noise have primarily evolved from improvements in engine and airframe technologies and design.4 These improvements also include more-electric passenger aircraft, such as The Boeing Co. 787 Dreamliner™, which overcame challenges while pioneering the safe use of LIB technologies for on-board aircraft systems.
More recently, an evolutionary technology shift toward decarbonizing the passenger aviation sector has emerged in the form of all-electric advanced air mobility (AAM) and electric hybridization of regional and single-aisle airliner applications.5,6 Various socioeconomic incentives, geopolitical demands, and global climatechange mitigation targets are driving widespread electrification of fossil fuel–consuming modes of passenger transportation. The early stages of decarbonizing the transportation sector are being led by the adoption of various electrified modes of road, waterborne, and rail transport. Enabled by lower cost, high specific energy LIB technologies, improvements to charging infrastructure, tax credits, and internal combustion engine–vehicle phase-out plans, global electric vehicle (EV) sales are expected to exceed 30% of all vehicles sold by 2030.7 Although the commercial aviation industry has experienced limited adoption of electrified passenger aircraft, the Federal Aviation Administration (FAA) has recently implemented policy changes aimed at the adoption of standards for reaching netzero greenhouse gas (GHG) emissions from the US aviation sector by 2050.8 In response, new aircraft designs utilizing advanced energy storage technologies are being developed to achieve FAA 2050 netzero GHG emissions goals and objectives.
Demand for new modes of sustainable air transportation will depend on safe and reliable high specific energy storage solutions. The emerging all-electric urban air mobility (UAM) market can have a significant impact on reducing the carbon footprint of vehicles in congested urban environments. All-electric vertical take-off and landing (eVTOL) aircraft supporting the UAM aviation market can only be achieved by improving LIBs so that they are capable of supporting the demanding flight requirements. Recent sizing studies
of 4-passenger eVTOL architectures indicate that LIBs with specific energies between 300 and 400 Wh/kg are required to meet energy demands for a reference 100-mile commercial flight.9 Battery pack specific energy needs for longer range hybrid-electric regional and narrow body class aircraft are estimated to range from 600 to over 800 Wh/kg, respectively.10,11 Factoring in battery packaging, electrical performance, environmental, and safety requirements, scaling analysis estimates suggest that Li-ion cell specific energies between greater than 500 and 600 Wh/kg would be required to meet the forecasted LIB-level specific energy requirements.12,13 Furthermore, UAM feasibility is dependent upon a compliant LIB power system capable of simultaneously meeting specific energy, power density, cycle life, fast charging, and stringent safety requirements across a wide range of operating conditions.
Recent years have seen significant increases in LIB performance and safety capabilities developed for EVs; and ongoing initiatives continue to extend the range of those vehicles. Despite the performance and safety maturity of state-of-the-art (SOA) Li-ion cell technologies, the UAM application market presents a significant challenge for the design of compliant LIB power systems.4,14 As a practical example, EV performance requirements present a basis for contrast with eVTOL capability requirements. As shown in Table I, a comparison of a commercial long-range EV to a representative eVTOL platform indicates that significant improvements in LIB energy and power are needed to support nominal eVTOL system requirements. In addition, new LIB technologies must comply with other emerging eVTOL system requirements such as cycle life, power demand, and safety characteristics.
Regardless of the specific flight profile and utilized propulsion configuration, all-electric aircraft batteries require significant increases in specific energy, continuous and peak discharge rate capability, recharge rate, and cycle life. Figure 1 depicts a representative eVTOL flight versus energy consumption profile for a reference single transit cruise cycle.
Both the takeoff and the descent toward landing phases of flight demand high power to balance the aircraft mass (craft with passengers and cargo), with the descent needing to extract that power when the battery is nearly depleted following cruise. High energy is necessary to achieve the desired flight range to support passenger-unique pickup and drop-off locations. Once landed, passengers disembark, and the flight craft will be briefly recharged. A higher-rate charge recovers a portion of the consumed battery energy to support fast turnaround for repeated subsequent scheduled flights throughout the
day. Battery sizing also includes reserve energy to support emergency flight contingencies. At the end of the flight day, a nominal recharge rate would then be utilized to return the flight battery to a high state-of-charge in preparation for the next day’s flight missions. Table II summarizes general Li-ion cell-level characteristics for a representative eVTOL LIB compared to a commercial EV-type application. Electric aircraft battery discharge rates may vary widely, from 1C to greater than 5C, depending on aircraft takeoff and landing characteristics.15,16
It is evident that, although SOA Li-ion cell technologies meet today’s commercial EV battery requirements, their performance capabilities do not align with the demands of eVTOL flight expectations. Much like the introduction and continuous-improvement demonstrated by EV batteries, there is an expectation that the viable business case justification for eVTOLs will rely upon a first-to-market strategy with the rapid pull-ahead of next-generation energy source options. The battery electric eVTOL aircraft type has been used in this discussion of battery performance requirements, but conventional takeoff and landing electric aircraft (eCTOL) and short takeoff and landing aircraft (eSTOL) with full electric and hybrid electric powertrains should also be considered. Relative to Fig. 1, these aircraft types would not hover but would have non-vertical takeoffs and landings and ground taxi segments at the beginning and end of the mission. These concepts may have lower specific power or recharge rate requirements but are still very sensitive to battery performance, especially specific energy and cycle life. These eSTOL and eCTOL and hybrid electric aircraft powertrains are also much more scalable to regional and large commercial aircraft than are pure electric designs.17
Li-ion cell technology is a generalized term for multiple electrochemical couples, active materials, and formulations. These typically include a
variety of anode and cathode materials, each developed and optimized to provide increased performance in certain key characteristics. Figure 2 compares a traditional aviation rechargeable battery, SOA Li-ion, and future Li-ion active materials in terms of projected cell and battery specific energy improvements.18,19 Although many of the high energy future Li-ion cell materials may meet the size and weight requirements of the electric aviation market, cycle life, chargedischarge rates, safety, and cost often remain as limitations. No current SOA Li-ion system is ideally suited to provide all the desired energy storage performance for electric aviation applications. Flight requirements for eVTOL, eCTOL, and eSTOL type aircraft greatly exceed these SOA materials capabilities, which is driving ongoing material and cell-level research and development efforts focused on advancing electrode materials, separator interfaces, electrolyte, and safety improvements. Additionally, electric aviation batteries can be an order of magnitude higher in cost than EV batteries due to extensive aviation safety certification testing requirements and lower production quantities. If electric aviation batteries continue to retain such a price premium, it could be challenging to establish competitive operating costs and market economics for all-electric or hybrid-type aircraft.
Fig 1. Battery energy and power demand for a simplified eVTOL single mission flight cycle. FigThe numerous UAM aircraft developers are thus taking different approaches to address the stated design challenges. Some are initially relying upon the limited capabilities of commercial-off-the-shelf (COTS) cells within well-understood safety-compliant designs. This approach allows them to focus on other aspects of aircraft certification with plans to adopt improved cell designs as they mature. Yet others are partnering with leading cell and battery companies that are working to accelerate and produce the next promising electrochemical couple. Although many have documented various comparable rechargeable Li-ion cell technology roadmaps, NASA’s Li-ion cell technology roadmaps anticipate that a viable 400Wh/kg pack level introduction could be as late as 2035.10 These emerging electrochemistry configurations represent the potential cell solutions aggressively pursued by the UAM market for adoption on electric and hybrid aircraft.
In human-rated space applications for NASA programs, LIB designs are required to be safe for ground processing, crew handling, operation in the enclosed environment of a crewed space vehicle, and safe to be mounted or used in unpressurized spaces adjacent to habitable areas.20 Lithium battery powered devices intended for use or transportation on US Navy submarines, ships, vessels, and aircraft are governed by requirements and guidelines found in various Naval Sea Systems Command standards.21 In response to various industry LIB safety incidents, these safety standards have been revised to include requirements for module and battery-level cell-to-cell thermal propagation tests to determine the severity of a catastrophic thermal runaway failure of one cell on neighboring cells in a real battery configuration.22-24
Passenger vehicle battery standards also recommend thermal propagation abuse testing for all-electric, hybrid, and plug-in hybrid EVs.25 Similarly, rechargeable and non-rechargeable lithium batteries used in commercial all-electric passenger aircraft must comply with safety requirements guided by FAA and RTCA standards.26, 27 These design standards share common requirements for hazard control, design evaluation, and verification testing and analysis.
Safety certification of rechargeable LIBs and battery systems for commercial passenger aircraft applications are regulated by RTCA DO-311A, which specifies safety through design, performance characterization, qualification, and installation requirements for the intended aircraft battery. DO-311A also specifies LIB safety design mitigations intended to avoid abusive operating conditions; addresses the potential for cell-to-cell failure propagation through thermal management and vented emissions control; and containment of any resulting multiple-cell failures. As next-generation Li-ion cell specific energy and volumetric energy density increases beyond SOA Li-cell technologies, the complexities of new battery packaging strategies, battery management systems, and thermal management approaches needed to meet DO-311A and other applicable standards becomes increasingly challenging.
To ensure safe electric aircraft operation, aircraft-level performance requirements and the limitations of the integrated LIB power system must be well characterized and understood. As such, industry lessons
learned from relevant LIB safety incidents indicate that the high specific energy and potential thermal instability of multi-cell LIBs contributes to the severity of catastrophic LIB safety hazards. Since the underlying mechanism of catastrophic thermal runaway is always present for individual LIB cells, thermal runaway containment is prescribed as the primary safety approach by aircraft regulations.28,29 However, LIB thermal runaway safety can be achieved using a variety of design approaches. For example, the likelihood of cell-tocell thermal runaway propagation has been proven to be effectively mitigated with appropriate packaging of battery cell modules. NASA has recently pioneered alternate safety methodologies which use passively propagation resistant (PPR) LIB electrical, mechanical, and thermal design features intended to mitigate the severity and consequences of cell-to-cell propagating thermal runaway.30,31
PPR LIBs are characterized by various design guidelines based on recent NASA experience with 18650 Li-ion cell-based LIBs supporting various human-rated space and passenger aviation programs.32 The first guideline is to control or mitigate the occurrence of 18650 cell can wall rupture during thermal runaway (Fig. 3). Commercial 18650 cylindrical cells are designed to vent their thermal runaway ejecta through their cap header and/or through a bottom vent if present. Breaches of the cell can wall or cap spin groove can occur as a high-pressure relief or melt-through event during the exothermic release of flaming gas, liquids, and solids. Breaches that eject highmomentum bursts of extremely hot ejecta material can lead to rapid thermal runaway propagation to adjacent cells.33
Secondly, LIB pack designs with cells in direct contact are almost certain to propagate thermal runaway. Battery designers can choose to thermally isolate cells from each other or to maximize heat conduction by heat sinking from cells. Although increasing cell-tocell spacing for thermal isolation creates a high-volume impact to the LIB design, this design approach has been proven to mitigate the severity of cell-to-cell thermal propagation.34
Thirdly, if a Li-ion cell in a parallel-series (p-s) configured LIB design proceeds into a failed thermal runaway condition, the resulting in-rush current will create an external short-circuit effect on electrically connected cells. As a result, cell-level overcurrent protection (such as individual cell fusing) can be used to protect cells electrically connected in parallel with the failed cell. Consistent (continued on next page)
Fig 3. Sidewall rupture of a Li-ion 18650 cell during thermal runaway. (Credit: NASA.)with DO-311A regulatory requirements and guidelines, LIBs used in passenger aircraft applications may also be required to have venting provisions designed to provide a pathway for relieving excessive pressure due to battery system emissions resulting from abnormal operation.35 Examples of vent mechanisms include vent ports (with flame-arresting properties), burst disks, or other devices.
An illustrative example of PPR implementation for airborne propulsion is NASA’s X-57 all-electric fixed-wing aircraft LIB power system.36 The NASA X-57 LIB incorporates numerous safety features to ensure suitability for passenger aircraft operation (Fig. 4). These features include high voltage safety accommodations for passengers and ground maintenance personnel, considerations for aircraft-specific protections and controls, fault and failure monitoring and reporting, and a unique mechanical packaging approach that is resilient to an aerospace-specific operating environment. In addition, fundamental to its thermal runaway protection strategy is the application of PPR design principles.
The X-57 LIB leverages multifunctional cell encasement methods to achieve the aims of PPR. The cell encasement is the primary structure and encapsulation of the battery module, providing both mechanical stability and environmental protection. Additionally, the metallic honeycomb design reinforces the cell sidewall and directs thermal runaway toward a central battery plenum and away from the adjacent cells. The central plenum contains flame and ejecta from cells in a thermal runaway and gas products are exhausted from the LIB module.
Adjacent cells are also protected by the honeycomb cell case (Fig. 5). The case acts to transfer the heat away from the affected cell and distributes it through the battery in a manner which avoids excessively overheating the surrounding cells. Thermally and electrically insulative materials included around the cells allows for reduced cell spacing and greatly increased volumetric packaging efficiency. To prevent cascading thermal runaway caused by cell damage from secondary short circuits, fusible linkages are used to disconnect the cells from the current collectors. This feature prevents additional cell heating from overcurrent discharge.
The worst-case cell position was identified, and multiple tests were performed in the module to demonstrate and qualify the module for manned flight. For each credited test, thermal runaway was induced in the trigger cells and cell failure was observed. However, adjacent cells in the module retained their ability to hold charge and provide power to the module after the conclusion of the cell thermal runaway event.37
For the near-term, the UAM market will have to tolerate the capabilities of leading-edge improvements to existing materials chemistry complemented by improved packaging methods that maintain safety while optimizing the realized module mass. Although most efforts to resolve the performance demands of eVTOL aircraft are intently focused upon the rapid evolution of next-generation electrochemistry options, there remain opportunities to optimize the necessary failure-mitigation strategies.
An alternative way to promote eVTOL commercialization in the near future is to design eVTOL cruise range based on SOA LIBs while improving the batteries’ fast-charging capability. Most intracity hops have trip distances within 50 miles, which can already be covered by SOA LIBs. However, eVTOLs generate revenue primarily in rush hours, so it is essential to ensure continuous operation during this period. The conventional approach is to design a long-range eVTOL with a large battery pack to warrant repetitive operation throughout the rush hours, which poses a formidable challenge to battery specific energy as well as vehicle and battery cost. The other path is to design an aircraft with a small battery pack for a short cruise range but quickly replenish the required energy for the next trip during passenger swapping between two trips. The latter has the potential to make eVTOLs technologically viable with SOA LIBs and also has the advantage of significantly reduced battery and vehicle costs. We should note that the anticipated high utilization rates of eVTOL vehicles for maximized revenue and the high frequency of fast charging pose a critical challenge to battery life. Hence, future research should focus on simultaneously improving battery specific energy, fast charging capability, and cycle life. These improvements will similarly benefit hybrid electric, eSTOL, and eCTOL aircraft, the success of which also depends on significant battery technology improvements.
© The Electrochemical Society. DOI: 10.1149/2.F010223IF
Fig 5. NASA X-57 LIB thermal runaway safety testing. (Credit: NASA.) Fig. 4. NASA X-57 PPR 20p16s LIB module. (Credit: NASA.)Thomas P. Barrera, President, LithiumIon Battery (LIB)-X Consulting Education: PhD in chemical engineering (UCLA, under the direction of Ken Nobe), postdoctoral research fellow in materials science and engineering (UCLA, under the supervision of Bruce Dunn). MS in industrial engineering (Northwestern University) and BS in chemical engineering and BA in mathematics-economics (UCSB).
Research Interests: LIB power systems engineering.
Work Experience: Previously, Tom was a Technical Fellow for The Boeing Co. where he led multidisciplinary teams in the endto-end development of advanced electrical power and battery energy storage systems for government and commercial spacecraft applications.
Pubs + Patents: Tom holds three US patents in the area of aviation battery safety and currently serves as editor-in-chief for a forthcoming reference book, Spacecraft Lithium-Ion Battery Power Systems (John Wiley & Sons).
Awards: AIAA Associate Fellow.
Work with ECS: Member-at-large, Battery Division; Institutional Engagement Committee.
Website: https://www.libxconsult.com/ https://orcid.org/0000-0001-8311-1889
James R. Bond, Director, Applications Engineering, EaglePicher® Technologies
Education: MS and BS in Electrical Engineering (Missouri University of Science & Technology).
Research Interests: Technology selection and integration for energy storage and power delivery system solutions; large-format Lithium-ion cells for space applications; commercial Lithium-ion aviation batteries.
Work Experience: Serving over 31-years across multiple roles in Engineering, and with an extensive Systems Engineering background, James worked to characterize large-format Lithium-ion cells for Space applications, led the development of EaglePicher’s first Lithium-ion commercial aviation battery, and presently leads the Next-Generation Aviation initiative that engages EaglePicher’s breadth of cell, battery, and systems integration technologies with the growing demands within electrified aviation.
Pubs + Patents: Five US patents ranging from mechanical systems innovations to a predictive algorithm for a state-of-health computational method. Website: https://www.eaglepicher.com/ https://orcid.org/0000-0002-6460-2233
Marty Bradley, Sustainable Aviation Consultant
Education: PhD, MS, and BS in Aerospace Engineering (University of Southern California).
Research Interests: The creation and progression of designs for electric and hybrid electric aircraft and sustainable aviation technologies
Work Experience: Marty has supported multiple start-up companies developing electric and hybrid electric aircraft, including Electra.aero and Ampaire. He is a lecturer at the University of Southern California where he teaches aircraft design and advises research teams on electric and sustainable aviation. As Technical Fellow for The Boeing Co., he led teams and developed advanced technology concepts for NASA and the US Air Force, including the trailblazing NASA SUGAR Volt concept that became the vision vehicle and research focus for future generations of commercial airliners.
Pubs + Patents: 2 patents and >30 publications and is a leader in electrified aircraft projects for the AIAA and IEEE Awards: AIAA Fellow
https://orcid.org/0000-0001-5208-577X
Rob Gitzendanner, Director of Applications Engineering, EaglePicher® Technologies
Education: PhD in Inorganic Chemistry (Cornell University), BS in Chemistry (University of Pennsylvania). Post-doc at the University of St. Andrews in lithium-ion cells and specifically synthesis and performance of new cathode materials.
Research Interests: Energy storage solutions; development of lithium-ion batteries and new technologies for aerospace and defense applications.
Work Experience: Lead project engineer on the MSP01 Mars Lander program, which resulted in the development and qualification of the first lithium-ion battery for a NASA space mission. Rob has led many military and aerospace production programs as program manager, including the NASA Orion Crew Module, B-2 Bomber, and Global Hawk unmanned aerial vehicle.
Website: https://www.eaglepicher.com/ https://orcid.org/0000-0002-7578-0190
Eric C. Darcy, Battery Technical Discipline Lead at NASA-Johnson Space Center
Education: PhD in chemical engineering (University of Houston).
Research Interests: Battery design, verification, and safety assessments for the rigors of manned spacecraft applications.
Work Experience: Over his 35-year career at NASA, Eric has developed safe, high performing battery systems with a focus on understanding, preventing, and mitigating cell latent defects that can lead to catastrophic hazards. He is co-inventor of a cell implantable internal short circuit device and fractional thermal runaway calorimeter providing significant design insights into cell responses during thermal runaway and enabled valid battery propagation assessments. https://orcid.org/0000-0002-8426-8607
Education: PhD and MS in aerospace engineering (Georgia Institute of Technology), BS in mechanical engineering (Brigham Young University).
Research Interests: Technical development of lightweight, certifiable battery technologies and innovative battery-based integrated propulsion systems.
Work Experience: At EP Systems, Dr. Armstrong has led multiple battery design, demonstration, test, and deployment programs, including the battery for NASA’s X-57 Maxwell. Prior to his role at EP Systems, he was the Hybrid Propulsion lead for Rolls-Royce North American Technologies, where he led propulsion systems design for various advanced electrified vehicle concepts, both fixed wing and VTOL.
Pubs + Patents: >25 patents filed; publications in advanced design methods, engine integrated electrical systems, and aircraft electric propulsion.
Website: https://epsenergy.com/ https://orcid.org/0000-0002-3252-1527
Chao-Yang Wang, William E. Diefenderfer Chair & Professor of Mechanical Engineering & Professor of Chemical & Materials Science & Engineering, Pennsylvania State University
Research Interests: The transport, materials, manufacturing, and modeling of batteries and fuel cells.
Work Experience: Dr. Wang is Director of the Electrochemical Engine Center at Penn State and is known for his innovative work in batteries and fuel cells; particularly for the crossdisciplinary understanding of transport and electrochemistry in fuel cells and for pioneering a new battery paradigm with switchable interfaces. The all-climate battery (ACB) he invented was adopted by 2022 Winter Olympics as well as commercialized by several carmakers. His latest inventions on fast charging batteries (FCB), safe, energy-dense batteries (SEB), and thermally modulated cell (TMC) technology have received widespread attention in industry. Pubs + Patents: 220+ journal publications, 37,000+ citations, and an h-index of 108. He holds over 140 patents and has published two books, Battery Systems Engineering (Wiley) and Modeling and Diagnostics of Polymer Electrolyte Fuel Cells (Springer). Honors and Awards: Fellow of the National Academy of Inventors (NAI) and the American Society of Mechanical Engineers (ASME). Work with ECS: ECS Member. Website: https://www.mri.psu.edu/wang-chao-yang
1. D. G. Vutetakis, Encyclopedia of Electrochemical Power Sources, J. Garche, C. Dyer, P. Moseley, Z. Ogumi, D. Rand, and B. Scrosati, Editors, p. 174–185, Elsevier (2009).
2. M. Waller, The Use of Electric Batteries for Civil Aircraft Applications, M. Waller, Editor, p. 1–10, SAE International (2018).
3. W. Yalen, M. Gulbinska, F. Puglia, and R. Gitzendanner, R., 6th International Energy Conversion Engineering Conference, AIAA 2008-5692, Cleveland, Ohio (2008).
4. National Academies Low Carbon Aviation Committee, Commercial Aircraft Propulsion and Energy Systems ResearchReducing Global Carbon Emissions, National Academies (2016).
5. C. Lents and Z. Baig, AIAA 2020-3555 (2020).
6. M. K. Bradley and C. K. Droney, NASA CR 2015-218704, Phase 2 (2015).
7. BloombergNEF, Zero-Emissions Vehicle Factbook (2021).
8. Federal Aviation Administration, United States 2021 Aviation Climate Action Plan (2021).
9. M. J. Duffy, S. R. Wakayama, R. Hupp, R. Lacy, and M. Stauffer, 2017 AIAA Aviation Technology, Integration, and Operations Conference (2017).
10. Argonne National Laboratory, The U.S. Department of Energy Vehicle Technologies Office and National Aeronautics and Space Administration Joint Assessment of the R&D Needs for Electric Aviation, White Paper, September (2021).
11. A. Bills, S. Sripad, W. L. Fredericks, M. Singh, and V. Viswanathan, ACS Energy Letters, 5 (2), 663–668 (2020).
12. J. Chin, K. Look, E. O. McNichols, D. Hall, J. Gray, and S.L. Schnulo, AIAA Propulsion and Energy 2021 Forum (2021).
13. C. Silva, E. Johnson, E. Solis, M. Patterson, and K. Antcliff, 2018 Aviation Technology, Integration, and Operations Conference, AIAA (2018).
14. V. Viswanathan, A. Epstein, Y. Chiang, E. Takeuchi, M.K. Bradley, M. Langford, and M. Winter, Nature, 601, 519–525 (2022)
15. X. G. Yang, T. Liu, S. Ge, E. Rountree, and C. Y. Wang, Joule, 5, 1–16 (2021).
16. W. L. Fredericks, S. Sripad, G. C. Bower, and V. Viswanathan, ACS Energy Lett. 3, 2989–2994 (2018)
17. K. Antcliff et al., Regional Air Mobility - Leveraging Our National Investments to Energize the American Travel Experience, NASA (2021).
18. M. Greenwood, M. Wentker, and J. Leker, Journal of Power Sources Advances 9, 100055 (2021).
19. S. Sripad and V. Viswanathan, Proceedings of the National Academy of Sciences, 118, e2111164118 (2021).
20. National Aeronautics and Space Administration, Crewed Space Vehicle Battery Safety Requirements, JSC 20793 (2017).
21. Naval Sea Systems, NAVY Lithium Battery Safety Program Responsibilities and Procedures, NAVSEA S9310-AQ-SAF-010 (2020).
22. National Transportation Safety Board, Aircraft Incident Report: Auxiliary Power Unit Battery Fire Japan Airlines Boeing 787-8, JA829J Boston, Massachusetts January 7, 2013, NTSB/AIR14/01, PB2014-108867, Notation 8604 (2014).
23. Japan Transport Safety Board, Aircraft Serious Incident Investigation Report, All Nippon Airways Co., LTD, JA804A, JTSB AI2014-4 (2014).
24. Air Accidents Investigation Branch, Report on the serious incident to Boeing B787-8, ET-AOP London Heathrow Airport 12 July 2013, Aircraft Accident Report 2/2015, Department for Transport Air Accidents Investigation Branch (2015).
25. Society of Automotive Engineers, Electric and Hybrid Electric Vehicle Rechargeable Energy Storage System (RESS) Safety and Abuse Testing, J2464 (2021).
26. Radio Technical Commission for Aeronautics, Minimum Operational Performance Standards for Rechargeable Lithium Batteries and Battery Systems, DO-311A (2017).
27. RTCA, Minimum Operational Performance Standards for NonRechargeable Lithium Batteries, DO-227A (2017)
28. G. H. Waller, J. K. Ko, T. H. Hays, and T. L. Jiang, Evaluation of Cell-to-Cell Propagation in Lithium-ion Batteries Containing 18650 Sized Cells, NSWCCD-63-TR-2020/01 (2020).
29. L. Torres-Castro, A. Kurzawski, J. Hewson, and J. Lamb, J. Electrochem. Soc., 167, 090515 (2020)
30. E. Darcy, Sustainable Aircraft Symposium, Redwood City, CA (2016).
31. E. Darcy and S. Scharf, The NASA Aerospace Battery Workshop, Huntsville, AL (2015).
32. E. Darcy, J. Darst, W. Walker, M. Keyser, D. Finegan, and G. Bayles, Driving Design Factors for Safe, High Power Batteries for Space Applications, Advanced Automotive Battery Conference, San Diego, CA (2018).
33. C. Fortune, E. Darcy, T. Adams, and J. Fontaine, Specification for Side Wall Rupture Characterization of Commercial Li-ion Cell Lots NASA EP-WI-038 (2021)
34. C. F. Lopez, J. A. Jeevarajan, and P. P. Mukherjee, J. of Electrochem. Soc., 162, A1905 (2015).
35. S. Sripad, A. Bills, and V. Viswanathan, MRS Bulletin, 46, 435–442 (2021).
36. NASA X-57. www.nasa.gov/specials/X57/ (last accessed 13 June 2022).
37. D. H. Lugo, S. Clarke, T. Miller, M. Redifer, and T. Foster, Beyond Lithium Ion XI, Cleveland, OH (2018).
The Canada Section held its annual Spring Symposium in person on June 12, 2022, on the University of Calgary campus, in honor of Professor Viola Birss and her contributions to the field of electrochemistry. The ECS Canada Section Symposium Organizing Committee (Heather Andreas, Kunal Karan, and Venkataraman Thangadurai) organized the symposium. Sponsors included the
Dr. David Wilkinson, Professor, University of British Columbia
Dr. Anne Co, Professor, Ohio State University
Dr. Scott Paulson, Research Associate, University of Calgary
Dr. Marwa Atwa, Postdoctoral Researcher, Stanford University
University of Calgary Department of Chemistry and Faculty of Science and the NSERC-U of Calgary CREATE ME2 Program, led by Professor Edward (Ted) Roberts, Department of Chemical Engineering, University of Calgary. Canadian and international researchers made presentations:
Dr. Paul Addo, President/CEO, SeeO2 Energy
Dr. Keryn Lian, Professor, University of Toronto
Dr. Dustin Banham, Chief Technology Officer, Guangdong TaiJi Power
Dr. Brad Easton, Professor, Ontario Tech University
For more information about this event, and to see the ECS Canada Section’s plans for the future, visit the section’s website or email ecs@ electrochem.ca
The ECS Detroit Section held its 2022 officer election from May 13 to June 3, 2022.
Chair Erik Anderson, American Battery Solutions
Vice Chair Tobias Glossmann, Mercedes-Benz R&D North America
Secretary Jiazhi (Emily) Hu, General Motors
Treasurer Vikram Patil, Freudenberg Battery Power Systems
Counselor Kris Inman, Freudenberg Battery Power Systems
Counselor Dennis Corrigan, Oakland University
Scribe Eleni Temeche, Mercedes-Benz R&D North America
Ambassador Ankun Yang, Oakland University
The new officers’ terms of office are two years or until their successors have been qualified. Their terms begin officially at 0800h ET on January 1 of the calendar year following the election. Learn more about the section’s new slate of officers on the ECS News feed.
Professors Vignesh Sundaresan, Joaquín Rodríguez-López, and Andrew J. Wilson, along with ECS members in the Midwest region, voted to merge the former Chicago and Cleveland sections and expand to include ECS members in the states of Indiana, Illinois, Kentucky, and Ohio, thus creating the ECS Mid-America Section. In acting Chair Andrew J. Wilson’s words, “This special region is home to a great number of world-class leaders in electrochemical and solid state science, and a broad diversity of students, faculty, scientists, and industries. We are excited about the section’s potential
to advance elctrochemical science and enhance the careers of young scientists in the field.”
The newly formed section held its welcome reception on June 6, 2022 at Haymarket Pub and Brewery in Chicago. The event was co-hosted by the ECS Mid-America Executive Committee and the University of Illinois at Chicago’s NGenE Workshop, and provided food, fellowship, and networking opportunities for attendees.
The Mid-America Section sought executive officer nominations through August 15, 2022. A formal Officer Election takes place this fall.
The National Capital Section held its 2022 officer election from April 22 to May 13, 2022.
Chair Chunsheng Wang, University of Maryland
1st Vice Chair A. Shoji Hall, Johns Hopkins University
2nd Vice Chair Nikki Ritzert, American Dental Association, NIST
Secretary Alexander Zestos, American University
Treasurer R. J. Santucci, United States Naval Academy/Naval Research Laboratory
Steve Policastro, Naval Research Laboratory Paul Albertus, University of Maryland
The new officers’ terms of office are two years or until their successors have been qualified. Their terms begin officially at 0800h ET on January 1 of the calendar year following the election.
The ECS Pacific Northwest (PNW) Section continues to host webinars and digital workshops on special topics in electrochemistry. The June 28 webinar “Pressurized Steam Electrolysis and a VYZion for Sustainable E-Fuels” was presented by Dr. Dustin McLarty of Washington State University (WSU). Dr. McLarty discussed pressurized operation of solid oxide cells, and trade-offs in
Ronen Aronov, Director, Fuel Cell Program, Cummins, Cummins New Power Business Unit
Max Cimenti, Principal Engineer/Scientist, Ballard Power Systems
Kathy Ayers, Vice President of Research and Development, Nel Hydrogen
The workshop—organized by Yuyan Shao, Chief Scientist, Pacific Northwest National Laboratory, and moderated by Olga A. Marina, Chief Scientist, Pacific Northwest National Laboratory, and Aaron Feaver, Director, Joint Center for Deployment and Research in Earth Abundant Materials (JCDREAM)—featured panelists’ perspectives and visions about hydrogen technologies and deployment, R&D needs, and hydrogen’s roles in decarbonization.
On August 17, Dr. Dianne Xiao, Department of Chemistry, University of Washington, presented a webinar titled “Conjugated Metal–Organic Nanostructures: Synthesis and Electrochemical Applications.” Dr. Xiao covered the status and applications of electrically conductive metal–organic frameworks; how to control
performance and economics; EIS testing of vanadium-doped zirconia electrodes; and testing facilities at WSU which are available for collaboration.
On July 21, the section hosted the “Pacific Northwest Hydrogen Workshop” with leading industry panelists:
Qingying Jia, Principal Project Scientist, Plug Power Inc.
Mark Monroe, Principal Engineer, Microsoft Datacenter Advanced Development
Hui Xu, CTO, Green Hydrogen, Head, H2 Center of Excellence, Envision Energy Group
the morphology of 2D conductive metal–organic frameworks; and new insights and properties gained from the synthesis of truncated 0D and 1D architectures.
The section enjoyed a robust awards season. Mitchell Kaiser, PhD candidate in Chemistry, University of Washington, received the 2022 Electrochemistry Student Award (established in 2021) in recognition of his promise in the field. The section also collected applications for the 2022 ECS PNW Section Electrochemistry Research Award, sponsored by Gamry Instruments. This award was established in 2021 to be given annually to an independent scientist or engineer working in Washington, Oregon, or Idaho, in recognition of excellence in electrochemistry and solid state science and technology research. Winner to be announced!
The ECS Taiwan Section has great plans for the fall! The 2022 Fundamentals of Electrochemistry Workshop took place at the National Taiwan University of Science and Technology (NTUST) September 1–2, 2022. At this workshop, professional researchers and experts in the field demonstrated basic electrochemical theories
and new insights into practical applications to participating students. The workshop offered lectures on fundamental electrochemistry, including an introduction to electrochemistry and electrochemical analysis methods (e.g., cyclic voltammetry (CV); electrochemical impedance spectroscopy (EIS); rotating disc electrode (RDE)
(continued
voltammetry; and spectroelectrochemical techniques). Lecturers also presented on applications of electrochemistry topics ranging from batteries to fuel cells, solar cells, supercapacitors, electrochemical sensors, and advanced electrochemical techniques.
The 2022 International Conference on Green Electrochemical Technologies and the 2022 Annual Meeting of the Electrochemical Society of Taiwan take place November 10–12, 2022 in the Department of Chemical Engineering at the National Tsing Hua University, the ECS Taiwan Section’s home institution. This conference covers five major topics: batteries, capacitors and fast charging devices, photoelectrochemistry, electrochemical
technologies, and electrochemical driven conversions. The section is pleased to announce three prestigious plenary lecturers for this event: Professors Tsutomu Miyasaka from Toin University, Stefano Passerini from Karlsruher Institut für Technologie, and Hsisheng Teng from National Cheng Kung University. Exciting discussions on energy conversion and storage technology are anticipated! The conference gathers experts spanning sectors from academia to industry to discuss cutting-edge technologies and pressing issues in the field of electrochemistry. Fifteen keynote speeches and many more invited talks are planned to stimulate the exchange of brilliant ideas and opinions. Don’t miss out! Check out our conference website
Arizona Section Candace Kay Chan, Chair
Brazil Section Luis F. P. Dick, Chair
Canada Section Heather Andreas, Chair
Chile Section José H. Zagal, Chair
China Section Yongyao Xia, Chair
Detroit Section Eric Anderson, Chair
Europe Section Philippe Marcus, Chair
Georgia Section Open
India Section Sinthai A. Ilangovan, Chair
Israel Section Daniel Mandler, Chair
Japan Section Seiichi Mayazaki, Chair
Korea Section Won-Sub Yoon, Chair
Mexico Section Carlos E. Frontana-Vázquez, Chair
Mid-America Section Andrew J. Wilson, Chair
National Capital Section Chunsheng Wang, Chair
New England Section Sanjeev Mukerjee, Chair
Pacific Northwest Section Jie Xiao, Chair
Pittsburgh Section Open
San Francisco Section Gao Liu, Chair
Singapore Section Zichuan J. Xu, Chair
Taiwan Section Chi-Chang Hu, Chair
Texas Section Jeremy P. Meyers, Chair
Twin Cities Section Victoria (Vicki) Gelling, Chair
Through ECS Society, Division, Section, and Student Awards, the Honors & Awards Program recognizes outstanding technical achievements in electrochemistry and solid state science and technology, and exceptional service to the Society.
The Carl Wagner Memorial Award was established in 1980 to recognize mid-career achievement; excellence in research areas of interest of the Society; and significant contributions to the teaching or guidance of students or colleagues in education, industry, or government. The award consists of a framed scroll, sterling medal, complimentary meeting registration for the award recipient and a companion, dinner held in the recipient’s honor during the designated meeting, and ECS Life Membership. Materials are due October 1, 2022.
The ECS Toyota Young Investigator Fellowship, established in 2015 in partnership with the Toyota Research Institute of North America, encourages young professionals and scholars to pursue research into batteries, fuel cells, and hydrogen, and future sustainable technologies. Each year, at least one candidate receives the fellowship restricted grant of no less than US $50,000 to conduct the proposed research within one year, and a one-year complimentary ECS membership. Recipients must present at a Society biannual meeting and publish their research in a relevant ECS journal within 24 months of receiving the award. Materials are due January 31 annually.
Fellow of the Electrochemical Society, established in 1989 as the Society’s highest honor, recognizes advanced individual technological contributions in the field of electrochemistry and solid state science and technology, and active ECS membership. The award consists of a framed certificate and lapel pin. Materials are due February 1, 2023.
The Henry B. Linford Award for Distinguished Teaching was established in 1981 for excellence in teaching in subject areas of interest to the Society. The award consists of a silver medal and plaque containing a bronze replica thereof; $2,500 prize; Society Life Membership; and complimentary meeting registration. Materials are due April 15, 2023.
Leadership Circle Awards, established in 2002 to honor and thank our electrochemistry and solid state science partners, are granted in the anniversary year that an institutional member reaches a milestone level. Awardees receive a commemorative plaque and recognition on the ECS website and in Interface. Nominations are not accepted.
The Olin Palladium Award was established in 1950 to recognize distinguished contributions to the fields of electrochemical or corrosion science. The award consists of a palladium medal; wall plaque; US $7,500; ECS Life Membership; and complimentary meeting registration. Materials are due October 1, 2022.
The Vittorio de Nora Award, established in 1971, recognizes distinguished contributions to the field of electrochemical engineering and technology. The award consists of a gold medal and plaque that contains a bronze replica thereof; $7,500; Society Life Membership; and complimentary meeting registration. Materials are due April 15, 2023
The Battery Division Early Career Award Sponsored by Neware Technology Limited, established in 2020, encourages excellence among early career professionals in battery and fuel cell research with the primary purpose of recognizing and supporting the development of talent and future leaders in battery and fuel cell science and technology. The award consists of a framed scroll; US $2,000; and complimentary meeting registration. Nominations are accepted beginning October 15, 2022; materials are due March 15, 2023.
The Battery Division Postdoctoral Associate Research Award Sponsored by MTI Corporation and the Jiang Family Foundation, established in 2016, encourages excellence among postdoctoral researchers in battery and fuel cell research. The award consists of a framed scroll; US $2,000; and complimentary meeting registration. Two awards are granted each year. Nominations are accepted beginning October 15, 2022; materials are due March 15, 2023.
The Battery Division Research Award, established in 1958, recognizes excellence in battery and fuel cell research and encourages publication in ECS journals. The award, which recognizes outstanding contributions to the science of primary and secondary cells, batteries, and fuel cells, consists of a framed certificate and US $2,000. Nominations are accepted beginning October 15, 2022; materials are due March 15, 2023.
Highlights follow. Visit www.electrochem.org/awards for more information.The Battery Division Technology Award, established in 1993, encourages the development of battery and fuel cell technology, and recognizes significant achievements in this area. The award’s field of interest is “that area of electrochemical technology which deals with the design, fabrication, scale-up, performance, lifetime, operation, control, and application of devices (i.e., primary and secondary cells and batteries, and fuel cells) in which chemical energy can be converted into usable electrical energy by an electrochemical process.” The award consists of a scroll; US $2,000; and Battery Division membership while the recipient is an ECS member. Nominations are accepted from October 15, 2022; materials are due March 15, 2023.
Biannual Meeting Travel Grants are awarded at each Society biannual meeting. Many ECS divisions and sections offer travel grants to undergraduates, graduate students, postdoctoral researchers, and young professionals and faculty presenting papers at ECS biannual meetings. The awards consist of financial support ranging from complimentary meeting registration to luncheon/ reception tickets, travel support, and more. Divisions and sections maintain their own application requirements. 243rd ECS Meeting Travel Grant applications are accepted from December 2, 2022 to February 27, 2023.
The Corrosion Division H. H. Uhlig Award was established in 1973 to recognize excellence in corrosion research and outstanding technical contributions to the field of corrosion science and technology. The award consists of a scroll and US $1,500. Materials are due December 15, 2022.
The Corrosion Division Rusty Award for Mid-Career Excellence, established in 2021, recognizes a mid-career scientist or engineer’s achievement and contributions to the field of corrosion science and technology. The award consists of a framed certificate; US $1,000; complimentary meeting registration; and up to US $1,000 for travel expenses. Materials are due December 15, 2022.
The Electrodeposition Division Early Career Investigator Award, established in 2015, recognizes an outstanding young researcher in the field of electrochemical deposition science and technology. The award consists of a framed certificate and US $1,000. Nominations are accepted from October 1, 2022 to April 1, 2023
The Electrodeposition Division Research Award recognizes outstanding research contributions to the field of electrodeposition and encourages the publication of high-quality papers in the Journal of The Electrochemical Society. The award is based on recent outstanding achievement in, or contributions to, the field of electrodeposition, and is given to an author/co-author of a paper published in JES or another ECS publication. The award consists of a framed certificate and US $2,000. Nominations are accepted from October 1, 2022 to April 1, 2023.
The High-Temperature Energy, Materials, and Processes Division J. Bruce Wagner, Jr. Young Investigator Award, established in 1998, recognizes a young Society member who demonstrates exceptional promise for a successful career in science and/or technology in the field of high-temperature materials. The award consists of a scroll and US $1,000. If needed, the recipient may receive complimentary meeting registration and up to US $1,000 in travel expenses. Nominations are accepted from October 2, 2022 to February 1, 2023.
The Canada Section W. Lash Miller Award, established in 1967, recognizes publications and/or excellence in the field of electrochemical science and technology and/or solid state science and technology. Recipients must be Canadian residents who completed their last advanced education degree no more than 15 years before the award year. The award is a framed certificate and CAD $1,500. Materials are due December 31, 2022.
The Europe Section Heinz Gerischer Award, established in 2001, recognizes an individual or group of no more than three individuals who have made an outstanding contribution to the science of semiconductor electrochemistry and photoelectrochemistry, including the underlying areas of physical and materials chemistry of significance to this field. The award consists of a framed certificate and €2,000, and if needed, up to €1,000 in travel expenses. Materials are due September 30, 2022.
The Battery Division Student Research Award Sponsored by Mercedes-Benz Research & Development recognizes promising young engineers and scientists in the field of electrochemical power sources and encourages recipients to initiate or continue careers in the field. Eligible candidates must be enrolled in college or university at the nomination deadline. The award consists of a framed certificate and US $1,000. Nominations are accepted beginning October 15, 2022; materials are due March 15, 2023.
The Canada Section Student Award, established in 1987, recognizes promising young engineers and scientists in the field of electrochemical power sources. The award of US $1,500 is intended to encourage recipients to initiate or continue careers in the field. Materials are due February 28, 2023.
The Corrosion Division Morris Cohen Graduate Student Award was established in 1991 to recognize and reward outstanding graduate research in the field of corrosion science and/or engineering. The award consists of a framed certificate; US $1,000; and up to US $1,000 in travel expenses. Materials are due December 15, 2022.
The Korea Section Student Award, established in 2005, recognizes academic accomplishments in any area of science or engineering in which electrochemical and/or solid state science and technology is the central consideration. The award of US $500 is presented at a Korea Section meeting at which the recipient may speak on a subject of major interest in the field of electrochemical and/or solid state science and technology. Materials are due December 31, 2022.
The Pacific Northwest Section Electrochemistry Student Award Sponsored by Thermo Fisher Scientific, established in 2021, recognizes promising young engineers and scientists in Washington, Oregon, and Idaho pursuing PhDs in electrochemical engineering and applied electrochemistry. The award is US $1,000. Materials are due February 28, 2023.
The San Francisco Section Daniel Cubicciotti Student Award, established in 1994, assists a deserving student in Northern California pursue a career in the physical sciences or engineering. The award consists of a metal plaque and US $2,000. Up to two honorable mentions are also awarded, each receiving a framed certificate and US $500. Materials are due February 15, 2023.
The Sensor Division Student Research Award, established in 2021, recognizes promising graduate students for conducting outstanding research in the field of sensors. The award consists of a framed certificate; US $200; complimentary meeting registration; and Sensor Division Business Lunch ticket. Materials are due March 1, 2023.
ECS Summer Fellowships, established in 1928, assist students pursuing research from June through August in a field of interest to ECS. Up to four summer fellowships are awarded each year: the Edward G. Weston Fellowship, Joseph W. Richards Fellowship, F. M. Becket Fellowship, and H. H. Uhlig Fellowship. Recipients receive US $5,000 to support their research and publication of a summary report in Interface Materials are due January 15 annually.
The Colin Garfield Fink Fellowship, first awarded in 1962, assists a postdoctoral scientist/researcher pursue research from June through August in a field of interest to the Society. The award consists of US $5,000 and publication of a summary report in Interface Materials are due January 15 annually.
ECS General Student Poster Session Awards, established in 1993, acknowledge the quality and thoroughness in candidates’ work, the originality and independence of their contributions, the significance and timeliness of their research results, and the depth of understanding of the research topics and their relationship to the Society’s fields of interest. Each Society biannual meeting awards a first place of US $1,500; second place of US $1,000; and third place of US $500. Awardees receive a certificate and announcement accompanying the respective meeting’s “Biannual Meeting Highlights” Interface article. Applicants must submit abstracts to the General Student Poster Session. The 243rd ECS Meeting abstract submission deadline is December 2, 2022.
The ECS Outstanding Student Chapter Award (formerly the Gwendolyn B. Wood Section Excellence Award) was established in 2012 to recognize distinguished student chapters that demonstrate active participation in the Society’s technical activities, establish community and outreach activities in the areas of electrochemical and solid state science and engineering education, and create and maintain a robust membership base. The winning Outstanding Student Chapter receives a recognition plaque; US $1,000; and award recognition and chapter group photo in Interface or other electronic communications. Up to two chapters are named Chapters of Excellence and receive recognition certificates and acknowledgement in Interface Materials are due April 15.
The following are part of ECS Honors & Awards Program, which has recognized professional and volunteer achievement within our multi-disciplinary sciences for decades. Join us in celebrating our peers as we extend congratulations to all!
Fikile Brushett is an Associate Professor in the Department of Chemical Engineering at the Massachusetts Institute of Technology (MIT). Prof. Brushett is especially interested in the fundamental processes that define the performance, cost, and lifetime of presentday and future electrochemical systems. His research approach combines synthesis and characterization of redox-active materials, design and engineering of electrochemical reactors, and techno-economic modeling of electrochemical systems. He places a strong emphasis on connecting system-level performance and cost goals to materials–level property requirements, and on leveraging this knowledge to guide the exploration of new chemistries and reactor formats. Ultimately, Prof. Brushett aims to develop robust and transferable guiding principles for the design of materials, processes, and devices that harness electrochemical phenomena. His group currently works on redox flow batteries for grid storage and on electrochemical processes for carbon management and chemical manufacturing. He also serves as the Research Integration Co-Lead for the Joint Center for Energy Storage Research, a DOE-funded Energy Innovation Hub.
He received his BS in Chemical and Biomolecular Engineering from the University of Pennsylvania in 2006 and his PhD in Chemical Engineering from the University of Illinois at UrbanaChampaign in 2010 under the supervision of Professor Paul J. A. Kenis. From 2010 to 2012, he was a Director’s Postdoctoral Fellow in the Electrochemical Energy Storage Group at Argonne National Laboratory under the supervision of Dr. John T. Vaughey. In 2012, he began his independent career at MIT where his research group seeks to advance the science and engineering of electrochemical technologies that enable a sustainable energy economy.
Yue Kuo is Nesbitt Professor and Professor of Chemical Engineering at Texas A&M University. Prof. Kuo has extensive semiconductor research experience, especially on thin film–related processes, materials, and devices of TFTs and ICs. He worked in industry (i.e., IBM T. J. Watson Research Center at Yorktown Heights, and Data General Corp. Semiconductor Division in Silicon Valley) before joining Texas A&M University in 1998.
Prof. Kuo received a PhD in Engineering Science from Columbia University. He has a distinguished record of accomplishment in advancing solid state science and technology. His research has resulted in breakthroughs that have solved some long-term problems in industry, and his inventions are used worldwide. He has received
many awards and honors, including ECS Fellow, ECS Gordon E. Moore Medal, ECS Electronics and Photonics Division (EPD) Award, 10 IBM awards, and many global university, industry, government, and professional awards. He is also a Fellow of IEEE, American Vacuum Society, and Materials Research Society. He has served on advisory and review boards, and panels for US National Academies, industry, universities, and governments.
Prof. Kuo has served as ECS President (2018–2019) and EPD Chair, and on ECS journal editorial boards and many committees. He founded the world’s longest continuous ECS Thin Film Transistors (TFT) Symposium series (held for 32 years) and the Semiconductor Technology for Ultra Large Integrated Circuits (ULSIC vs. TFT) Conference series (held for 14 years). He has consulted for semiconductor companies and advised students in many countries.
For the paper, Quantifying the Impact of Charge Transport Bottlenecks in Composite Cathodes of All-Solid-State Batteries [P. Minnmann et al., J. Electrochem. Soc., 168, 040537 (2021)]
Philip Minnmann received his BSc and MSc in Material Science with distinction from RWTH Aachen University and is currently a PhD candidate at the Institute for Physical Chemistry at Justus-LiebigUniversity Giessen. His main research interest is investigating charge transport to gain a better understanding of the correlation between microstructure and cathode kinetics. He used impedance spectroscopy to determine partial conductivities and to relate kinetic limitations of composite cathodes during full cell cycling to electronic and ionic charge transport bottlenecks. He also studied the influence of the solid electrolyte particle size distributions on the charge transport pathways. His current focus is on limitations of sheet type composite cathodes and their optimization.
During his undergraduate studies, Minnmann worked at the Forschungszentrum Jülich with Rüdiger Eichel on the mechanochemical synthesis of solid lithium-ion conductors for solid state batteries. He later joined JLU Giessen, where he worked with Jürgen Janek on solid state batteries with a focus on composite cathodes as part of the Cluster of Competence for Solid State Batteries (FestBatt), funded by the German Ministry of Education and Research.
Simon Burkhardt is a development engineer and project manager for Li-ion batteries at a German battery manufacturer. While completing a PhD in Physics at Justus Liebig University Giessen, he researched charge transport in solids, specifically for the study of charge transport in transition metal oxides that are typically used in electrochemical energy storage or in electrochromic devices. He focused on the development and application of experimental methods to investigate mixed ionic and electronic conduction in single µm-sized particles and to study hydrogen diffusion in electrochromic thin films.
Burkhardt then worked as a research associate in Prof. Jürgen Janek’s research group at the Institute of Physical Chemistry. There, he contributed to the research and development of oxide and sulfide solid electrolytes as well as lithium and sodium all solid state batteries. He then coordinated FestBatt, a German research consortium on solid state batteries.
For the paper, Low-Temperature Parylene-Based Adhesive Bonding Technology for 150 and 200 mm Wafers for Fully Biocompatible and Highly Reliable Microsystems. [F. Selbmann et al., ECS J. Solid State Sci. Technol., 10, 074010 (2021)]
Franz Selbmann has headed the Flexible Devices Group at the Institute for Electronic and Sensor Materials at TU Bergakademie Freiberg since 2019. Their research focuses on flexible electronics and sensors, including related materials science on advanced and smart materials, research on fabrication and integration technologies, transfer processes, and developing new concepts for flexible devices.
He received his BS in Electronic and Sensor Materials at the Technische Universität Bergakademie Freiberg in 2012, developing advanced technologies for fine line metallization of solar cells as part of his thesis. In 2014, Selbmann supported the Silicon Saxony Association representative during a multi-month stay at the College of Nanoscale Science and Engineering at the New York State University Polytechnic Institute. He completed an MS degree in 2015 at Fraunhofer Institute for Electronic Nano Systems ENAS, Chemnitz with a thesis in the field of parylene encapsulation. In 2016, he researched advanced materials for microsystems at Tohoku University. His PhD is a collaboration between the Institute for Electronic and Sensor Materials at TU Bergakademie Freiberg and the Fraunhofer Institute for Electronic Nano Systems. He focuses on the development of parylene as a structural and functional material in microsystems as well as a material for flexible devices.
Feng Lin is an Associate Professor of Chemistry at Virginia Tech. His research covers the design and synthesis, processing, characterization, and applications of energy materials in electrochemical systems, including batteries, catalysis, and smart windows. His research team has also established an integral analytical program to study materials dynamics under operating conditions using operando synchrotron X-ray and electron analytical techniques.
Prof. Lin holds a BS in Materials Science and Engineering from Tianjin University (2009) and PhD in Materials Science from the Colorado School of Mines (2012) with joint research at the National Renewable Energy Laboratory. Before joining Virginia Tech in 2016, Prof. Lin worked at QuantumScape Corporation as a senior member,
and at Lawrence Berkeley National Laboratory as a Postdoctoral Fellow. His awards and recognitions include SLAC Spicer Young Investigator; Ralph E. Powe Junior Faculty Enhancement Award; Journal of Materials Chemistry Emerging Investigator; RCSA Scialog Fellow; American Chemical Society Petroleum Research Foundation Doctoral New Investigator; Energy Storage Materials Young Scientist Award; National Science Foundation CAREER Award; John C. Schug Faculty Research Award; ECS Battery Division Early Career Award; and Scientific Highlights by the US Department of Energy National Laboratories.
Paul Coman is a Research Assistant Professor in the Department of Chemistry at the University of South Carolina. His research focuses on developing mathematical modeling for improving the performance and safety of Li-ion batteries. Prior to his current position, Prof. Coman was a Postdoctoral Fellow at the same university in Ralph E. White’s group, working with various Li-ion battery projects—from modeling of thermal runaway to performance studies, aging and thermal modeling, and design of battery packs, in collaboration with Eric C. Darcy from NASA JSC. Prof. Coman graduated with his PhD from the University of Southern Denmark (SDU) after completing a BS at the Technical University of Iasi and MS at SDU. He has worked on modeling Li-ion batteries since 2013.
Photo: Christopher Woodley
Alexis Maurel is a Fulbright Postdoctoral Researcher in the Aerospace and Mechanical Engineering Department at the University of Texas at El Paso (UTEP). Dr. Maurel received the 2021 Fulbright France Research Fellowship to pursue his research at UTEP with Eric MacDonald (Mechanical Engineering) and Sreeprasad Sreenivasan (Chemistry Department). His research takes place at the ESTRELLA lab (Energy SToRage and ELectronics 3D Printing Laboratory) where he works on additive manufacturing of lithiumand sodium-ion batteries via material extrusion and vat photopolymerization, in close collaboration with his former PhD advisors, Sandia and Oak Ridge National Laboratories, Youngstown State University, and NASA. His current work is dedicated to 3D printing of batteries from lunar and Martian regolith.
Dr. Maurel received a 2-year diploma in Chemical Engineering in 2014, followed by his BS in Materials Chemistry in 2015 from Université Paul Sabatier. His research then focused on wastewater recycling in Mexico. In 2017, Dr. Maurel received his MS in Materials for Energy Storage and Conversion from a joint Erasmus Mundus program with Politechnika Warszawska, Universidad de Córdoba, Université Paul Sabatier Toulouse, and Université de Picardie Jules Verne. He completed his PhD in Materials Chemistry in 2020 at the Université de Picardie Jules Verne, researching battery 3D printing under the supervision of Loic Dupont, Sylvie Grugeon, and Stéphane Panier, in collaboration with Michel Armand. For this work, he received the Best PhD Thesis Prize.
Boryann Liaw is a Directorate Fellow at Idaho National Laboratory (INL). Since the early 1990s, Dr. Liaw has conducted laboratory and real-life battery and vehicle testing, data collection and analysis, battery modeling and simulation, battery performance and life prediction, battery fast charging technology development, and battery failure mode and effect analyses.
Dr. Liaw received his BS in Chemistry from National Tsinghua University, his MS in Chemistry from University of Georgia, and PhD in Materials Science and Engineering from Stanford University. He was a faculty member at University of Hawaiʻi at Mānoa for more than 27 years, co-founder of Ambient Micro, and founder of High Power Research Laboratory. He joined INL in May 2016 as Department Manager of Energy Storage and Advanced Vehicles. A Fellow of the Electrochemical Society, Dr. Liaw has actively served in Society leadership roles on several journal editorial boards, executive boards, and associate editorships. He is a Past President of the International Battery Materials Association and serves as scientific advisor to several international and national programs, including the Department of Energy’s Energy Frontier Research Center at Stony Brook University. His recent accolades include the IBA Technology Award (2020) and Asian American Engineer of the Year (2019).
Atsuo Yamada is Professor of Chemical System Engineering at the Tokyo Institute of Technology. His research, focused on battery materials, is particularly recognized for sophisticated approaches to structureproperty relationships, including very early stage exploration/optimization of LFP and, more recently, identification and understanding of several functional electrolytes.
Prof. Yamada’s career spans academic and industrial research. After serving as a Laboratory Head at the Sony Research Center, in 2002 he was appointed as Associate Professor at the Tokyo Institute of Technology, and then, in 2009, Full Professor at the University of Tokyo. Prof. Yamada spent a year as Visiting Scholar in John B. Goodenough’s lab at the University of Texas at Austin. On a sabbatical as Invited Professor at the Université de Bordeaux, he worked on enhancing research communication with Dr. Claude Delmas at the Institut de Chimie de la Matière Condensée de Bordeaux, a Joint Research Unit of the university and the Centre National de la Recherche Scientifique and the Institut Polytechnique de Bordeaux. Over the last decade, Prof. Yamada has led important Japanese national research programs: Elements Strategy Initiative for Catalysts and Batteries, Specially Promoted Research, Core Research for Evolutional Science and Technology, and Data Creation and Utilization Type Materials Research and Development Project. He now serves on the Advanced Energy Materials Scientific Advisory Board.
Among his many honors, Prof. Yamada received the American Ceramic Society Spriggs Award and Purdy Award, ECS Japan Scientific Achievement Award, and International Battery Association Research Award. He holds a PhD and 90 patents, has published 25 chapters and well over 260 refereed journal papers with over 28,000 citations, delivered 125 plenary, keynote, and invited presentations, and ranked as a Clarivate Analytics Highly Cited Researcher.
Singyuk Hou’s academic career has revolved around the research of electrolytes and interfaces for metal anodes. After completing her BS and MS in Chemistry, she started her PhD under the supervision of Prof. Chunsheng Wang at the University of Maryland, College Park. Her PhD research focuses on the molecule-level control of the solvation structure using chelants that promote the charge transfer kinetics of magnesium and calcium metal batteries. It also extends to SEI engineering for high-energy lithium-ion and lithium metal batteries by developing novel electrolyte systems. She has published more than 50 peer-reviewed papers and co-authored four patents.
John Holoubek is a PhD candidate and NASA Graduate Fellow at the University of California, San Diego under the supervision of Professors Zheng Chen and Ping Liu. He works on understanding the impact of electrolyte chemistry and structure on battery performance, primarily at reduced temperatures. This research integrates experimental investigation and computational modeling to connect variance in electrochemical behavior to molecular phenomena at the electrode/electrolyte interphase. The primary technological motivation is to improve the kinetic behavior of highenergy battery chemistries with an emphasis on the Li metal anode. Holoubek has also contributed to research in lithium-ion, aqueous, and dual-ion batteries.
Jun Lu is a scientist at Argonne National Laboratory. His research focuses on solving some of the world’s most challenging rechargeable Li-ion battery (LIB) and next generation Li battery problems. Over the past 10 years, he has pioneered and led research on novel cationic and anionic redox (CAR) materials for high energy density LIBs, LiO2, and Li-S batteries.
Dr. Lu has published more than 430 papers in journals that include Journal of The Electrochemical Society, Nature, Science, Nature Energy, Nature Nanotechnology, Nature Review Materials, Nature Communications, Chemical Reviews, Journal of the American Chemical Society, and Proceedings of the National Academy of Sciences. He has received more than 45,000 citations with an h-index of 117. He ranked as a Clarivate Analytics Global Highly Cited Researchers from 2018 to 2021. Dr. Lu has filed more than 20 patents and patent applications. He received the 2022 IBA Research Award; 2020 IBA Early Career Award; 2019 R&D 100 Award; 2019 American Chemical Society Division of Energy & Fuels Emerging Researcher Award; 2018 Rising Star Award for Argonne Commercialization Excellence; 2016 International Automotive Lithium Battery Young Investigator Award; and 2016 International Academy of Electrochemical Energy Science Award for Research Excellence in Electrochemistry Energy.
Mark Orazem is a Distinguished Professor and the Dr. and Mrs. Frederick C. Edie Professor in the Department of Chemical Engineering at the University of Florida (UF). He obtained his BS and MS degrees from Kansas State University and PhD in 1983 from the University of California, Berkeley. In 1983, he began his career as an Assistant Professor at the University of Virginia, and joined the UF faculty in 1988. He has received numerous UF term professorships and teaching awards and was recognized as a University of Florida Foundation Preeminence Professor. Prof. Orazem is a Fellow of The Electrochemical Society and the International Society of Electrochemistry (ISE), and served as ISE President from 2011 to 2013. He has more than 220 refereed publications. Prof. Orazem co-authored, with Bernard Tribollet of the Centre Nationale de la Recherche Scientifique (CNRS), Electrochemical Impedance Spectroscopy, which was published by Wiley in 2008. Chemical Industry Press published the textbook’s Chinese translation in 2014. The second edition appeared in 2017 with the Chinese translation in preparation. Woodhead Publishing published his edited book, Underground Pipeline Corrosion, in 2014. With co-author Bernard Tribollet, Prof. Orazem received the 2019 Claude Gabrielli Award for contributions to electrochemical impedance spectroscopy. Prof. Orazem received the 2012 Henry B. Linford Award for Distinguished Teaching.
Mariko Kadowaki is a Researcher at the National Institute for Materials Science in Japan. She finished her BS in 2016, MS in 2018, and PhD in 2021 in the Department of Materials Science at Tohoku University. During her PhD research, she applied a micro-electrochemical technique to clarify the relationship between the microstructure of carbon steels and pitting corrosion resistance. She also applied first-principles calculations to elucidate the electronic interaction between Fe and interstitial atoms in carbon steels. An active member of The Electrochemical Society, in 2017 she received a 232nd ECS Meeting Student Poster Session Award Honorable Mention. She won the 2021 Japan Society for the Promotion of Science Ikushi Prize as well as a L’Oréal-UNESCO For Women in Science Japan Fellowship for Materials Science.
David M. Bastidas is Associate Professor at The University of Akron in the Department of Chemical, Biomolecular, and Corrosion Engineering, and Corrosion Expert at the National Center for Education and Research on Corrosion & Materials Performance. His research focuses on electrochemical thermodynamics, kinetics of corrosion, and inhibition mechanisms. He leads the research lab in Corrosion Protection and Materials Performance.
Prof. Bastidas completed his BSc in Chemical Science and PhD in Material Science and Engineering at the Universitat de Barcelona. He served as Chair of the 2022 Corrosion and Materials Degradation Conference and Trustee of The University of Akron AMPP Section. Prof. Bastidas is the Faculty Advisor of the Corrosion Squad Student Association and received the Outstanding Campus Advisor Award and Outstanding Faculty Engagement Award in 2022. His research has garnered accolades that include the inaugural winner of the 2022 ECS Corrosion Division Rusty Award for Mid-Career Excellence; inclusion on the 2020 Top Researchers list; 2020 National Corrosion Award of Excellence; and Outstanding Research Career Award I3 Program. Prof. Bastidas has authored over 150 publications and presented at a number of invited conferences.
Nikolay Dimitrov is a Professor in the Department of Chemistry at The State University of New York at Binghamton. His research interests are in electrodeposition, catalysis, and design and synthesis of functional nanomaterials. Prof. Dimitrov’s basic-science research focuses on studying, understanding, and controlling the surface morphology evolution during electro- or electroless deposition of metals and alloys. His applied research projects emphasize a study of the performance and reliability of structures and devices associated with electronic packaging. Illustrating the outstanding practical value of electrodeposition, these projects prove instrumental for understanding, controlling, and improving the reliability of leadfree solder joints. He also contributed to the design and optimization of approaches for through-hole copper filling for the development of glass interposers applicable in 2.5/3D packaging.
Prof. Dimitrov completed his education (BS/MS from Sofia University and PhD from the Bulgarian Academy of Sciences) in 1993. He then held postdoctoral and research faculty appointments at Arizona State University until 2003 when he joined SUNY Binghamton. His multifaceted scholarly activity was recognized by the 2008 NSF CAREER Award and 2020 Distinguished Research Award of the American Chemical Society Binghamton Chapter.
Fudong Han is the Priti and Mukesh Chatter ‘82 Career Development Chair and Assistant Professor in the Department of Mechanical, Aerospace, and Nuclear Engineering at Rensselaer Polytechnic Institute. His research focuses on developing advanced materials for electrochemical energy storage, particularly solid state batteries, with the aid of advanced neutron-based characterization techniques.
Prof. Han received his BS in 2009 and his MS in 2012 in Materials Science and Engineering from Shandong University. He completed a PhD in Chemical Engineering in 2018 and a year of postdoctoral research at the University of Maryland, College Park. Prof. Han was a Guest Scientist in the Materials for Energy and Sustainable Development Group at the National Institute of Standards and Technology. He received the 2018 ECS Battery Division Student Research Award and 2017 Materials Research Society Graduate Student Gold Award. His work on battery research has led to two patents and patent applications. Prof. Han is the author
of more than 70 papers in scientific journals with 12,000 citations and an h-index of 50. He has also authored two book chapters on solid state batteries.
Katherine Ayers is Vice President of R&D for Nel Hydrogen US, with responsibility for developing and executing Nel’s technology strategy in proton exchange membrane electrolysis. Dr. Ayers manages a broad portfolio of internally and externally funded research projects, across a range of collaborators in academia, industry, and national labs. She is also the lead principal investigator on the US Department of Energy benchmarking program, spanning the primary water-splitting technologies funded by the Hydrogen and Fuel Cell Technologies Office (HFTO), with the goal of providing protocols and standards for consistency in water-splitting research.
Dr. Ayers completed her PhD in Chemistry at the California Institute of Technology working with Nate Lewis, and spent several years in the battery industry before joining Nel in 2007. She has served on multiple scientific advisory boards for Energy Frontier Research Centers and similar consortia such as the JCAP Solar Fuels Hub, as well as two federal-level advisory committees for the DOE: HTAC (Hydrogen and Fuel Cells Technical Advisory Committee) and BESAC (Basic Energy Sciences Advisory Committee). She received R&D Awards at the 2012 and 2021 DOE Merit Reviews from the HFTO Production Team, and an American Chemical Society Rising Stars Award in 2014 from the Women Chemists Committee. She also received the Fuel Cell Seminar Award in 2015. She became a Fellow of The Electrochemical Society in 2020.
Scott A. Barnett is a Professor in the Materials Science and Engineering Department at Northwestern University (since 1986). His past research topics include semiconductor growth by molecular beam epitaxy, ion-assisted thin film deposition, nitride hard coatings and superlattices, solid oxide fuel cells and electrolyzers, Li-ion battery cathodes, and electrode materials characterization using three-dimensional tomography and impedance spectroscopy. Current research interests include the development and characterization of novel fuel cell electrode materials, energy storage using solid oxide cells and Li-ion batteries, and accelerated testing to characterize cell degradation processes.
After receiving his PhD in Metallurgy from the University of Illinois at Urbana-Champaign in 1982, Prof. Barnett held postdoctoral appointments at the University of Illinois and Linkøping University (1983–1986). He has been a Fulbright Guest Professor at Centro Atómico Bariloche (2016), Otto Mønsted Guest Professor at the Danish Technical University (2013), Cheng Tsang Man Endowed Professor at Nanyang Technological University (2009), and a visiting scientist at Los Alamos National Laboratory (1998). Prof. Barnett was founder and Chief Technical Consultant at Functional Coating Technology, LLC (2001–2011) and Applied Thin Films, Inc.
(1998–2001). The author or co-author of more than 300 publications (h-index of 90), he has 15 patents, and has advised nearly 50 PhD students. Prof. Barnett is a Fellow of The Electrochemical Society, AVS, and American Ceramic Society. He was recognized as an Office of Naval Research Young Investigator.
Liangbing Hu is the Herbert Rabin Distinguished Professor at the University of Maryland, College Park. His research group focuses on materials innovation, device integration, and manufacturing in general, with ongoing research activities on wood nanotechnologies, 3000K extreme materials, and beyond Li-ion batteries.
Prof. Hu received his BS in Physics from the University of Science and Technology of China in 2002, where he worked on colossal magnetoresistance (CMR) materials for three years. He completed a PhD at the University of California, Los Angeles (2002–2007), focusing on carbon-nanotube–based nanoelectronics. In 2006, Prof. Hu joined Unidym, Inc. as a co-founding scientist, leading the development of roll-to-roll printed carbon nanotube films and device integration in touchscreens, LCDs, flexible OLEDs, and solar cells. During a postdoc at Stanford University from 2009 to 2011, he researched various energy storage technologies using nanomaterials/ nanostructures.
Prof. Hu has published some 400 research papers (including about 10 in Science and Nature). Among the many awards he has received are Clarivate Analytics Highly Cited Researcher (2016–2021); Wiley-Small Journal 2019 Young Innovator Award; Blavatnik National Awards Honoree; 2019 TAPPI Nano Middle Career Award; 2019 Exemplary Research Recognition; 2018/2020/2021 R&D 100 Winners; 2018 HIVE 50 Innovator; 2017 Nano Letters Young Investigator Lectureship; 2016 Office of Naval Research Young Investigator Award; 2016 American Chemical Society Division of Energy and Fuel Emerging Investigator Award; 2016 SME Outstanding Young Manufacturing Engineer Award; 2019 and 2014 University of Maryland Invention of the Year; and 2013 Air Force Young Investigator Award.
Kailash Mishra received his PhD in Physics from Sambalpur University, and completed post-doctoral research at the State University of New York at Albany. In 1985, he joined OSRAM Sylvania Lighting Research Center (then GTE Sylvania) as an advanced R&D engineer. Dr. Mishra continued working at OSRAM Sylvania first as a staff scientist and then as R&D Manager until his retirement in 2017 as the global Head of Technology Scouting. At the Lighting Research Center, Dr. Mishra was engaged in phosphor research for lighting applications.
Dr. Mishra’s main research interest is in the theory of electronic structures and associated properties of luminescent materials, and the fundamentals of luminescence. He is one of the early researchers who applied methods of quantum chemistry to study the structure and luminescence properties of materials. Dr. Mishra demonstrated
how to obtain a cogent picture of luminescence by applying band structure and cluster molecular orbital methods in a synergetic manner. In recent years, he has engaged in the application of quantum electrodynamics to study various luminescence processes in materials. Dr. Mishra is currently engaged in studying application of adiabatic approximation to investigate the temperature dependence of radiative and nonradiative processes in solids. He has coauthored more than 130 papers in peer-reviewed journals and has 10 patents.
Since joining ECS in 1998, Mishra has served as Chair of the ECS Luminescence and Display Materials Division, co-organized several ECS symposia, and co-edited multiple volumes of ECS Transactions He is a Fellow of The Electrochemical Society and has served as a Technical Editor of the ECS Journal of Solid State Science and Technology since 2011.
Anja Boisen is Head of Section and Professor in the Department of Health Technology at the Danmarks Tekniske Universitet (DTU). She heads IDUN, a Danish National Research Foundation and the Villum Foundation Center of Excellence that conducts research in micro and nano technology. Her research group focuses on the development and application of micro and nano mechanical sensors and microfabricated systems for oral drug delivery. Prof. Boisen co-founded the companies Cantion, Silmeco BluSense Diagnostics, and LightNovo, and is currently heading a research project on therapeutic drug monitoring that should result in a start-up company in a few years’ time.
Prof. Boisen completed an MS in Physics at Roskilde University and PhD at DTU in 1997. After a research period at IBM Almaden and postdoc at DTU, she joined DTU as Associate Professor in 1999 and became a full professor in 2005. Prof. Boisen is a board member of the Leo Foundation, Villum Foundation, Danish Academy of Technical Sciences, and Royal Danish Academy of Sciences. She has an h-index of 62 with more than 14,000 citations. Her work has garnered significant recognition. In 2021, Prof. Boisen was elected Fellow of The Electrochemical Society and MNE 2021 Fellow at the annual international conference on Micro and Nano Engineering (MNE). In 2020, she was awarded the Order of Dannebrog by Her Majesty Margrethe II, Queen of Denmark. Prof. Boisen received the 2013 Danish Council for Independent Research Sapere Aude Top Researcher Award; 2012 Danish Ministry of Research, Innovation and Higher Education EliteForsk Award; and 2008 Villum Kann Rasmussen Award (the largest Danish research prize).
David P. Wilkinson is Professor of Chemical Engineering and a Tier 1 Canada Research Chair in Clean Energy and Electrochemical Technologies at the University of British Columbia (UBC). His university research covers a wide range of electrochemical areas, including fuel cells, electrolyzers, battery research, carbon dioxide conversion, electrochemical approaches to clean energy and fuels, and
electrochemical treatment of wastewater and drinking water. Much of this research is used by companies, which resulted in the formation of Mangrove Lithium, a new company using a modified electrodialysis process for improved lithium refining.
He received his chemical engineering degree from UBC and then received his PhD in Chemistry in 1987 at the University of Ottawa under the guidance of Prof. Brian Conway. He was an ECS Summer Fellow during his PhD research on proton donor and medium effects in electrochemical proton discharge. Before joining UBC in 2004, Prof. Wilkinson spent over 18 years in electrochemical industries, as a group leader in electrochemistry at Moli Energy developing rechargeable Li metal batteries, and at Ballard Power Systems as a Director and Vice President of R&D in polymer electrolyte membrane fuel cell and hydrogen technology. He spent a short period as a Group Leader and Principal Research Officer with the Canada National Research Council. In 2002, he received the ECS Battery Division Technology Award for his work related to rechargeable Li batteries and polymer electrolyte fuel cells.
Prof. Wilkinson has published more than 230 refereed publications, a co-authored book, a number of edited books and book chapters, and holds over 82 issued patents. He has received a number of honors for his work, including the Grove Medal, Lifetime Award of the Canadian Hydrogen and Fuel Cells Association, and Order of Canada, as well as fellowships in the Engineering Institute of Canada, Canadian Academy of Engineering, Chemical Institute of Canada, and the Royal Society of Canada.
Sho Fujita is a PhD candidate in the Department of Chemistry at Queen’s University. His research interests lie in electrochemistry, electrocatalysis, and surface science. Fujita’s work at Queen’s under the supervision of Dr. Gregory Jerkiewicz focuses on the synthesis, design, and characterization of nanomaterials for microscopic rechargeable batteries. Fujita completed his BSc in Engineering and MSc in Chemical Engineering at Yokohama National University under the supervision of Dr. Shigenori Mitsushima. For his MSc, he collaborated with industrial companies on the degradation mechanism and electrocatalytic activity of a lithium-doped nickel oxide anode for use in alkaline water electrolyzers.
Jerry Barker is co-founder and Chief Scientist at Faradion Limited, a UK-based start-up specializing in Na-ion battery technology. In 2019, Dr. Barker was appointed Honorary Professor in the School of Chemistry at the University of St. Andrews. To target the low-cost and sustainable Li-CAM supply chain, he founded the start-up company, Redoxion Limited, in April 2022.
After completing a PhD in Solid State Electrochemistry from the University of Exeter, Dr. Barker worked at British Petroleum (BP) and spent time at the University of California, Santa Barbara. There he studied alkali ion doped polyacetylene with Nobel Prize laureates Alan Heeger and Hideki Shirakawa. As Chief Electrochemist at BP Solar, Dr. Barker developed a process for the large-scale electrodeposition of II-VI semiconductors for PV
applications. He also served as Chief Scientist and Research Director at Valence Technology, Inc.
Dr. Barker is the named inventor on more than 115 issued US patents covering numerous alkali ion active materials as well as the carbothermal reduction (CTR) volume manufacturing method. CTR is widely regarded as the benchmark process for the largescale synthesis of LiFePO4. His inventions have culminated in five commercially successful battery enterprises. Dr. Barker has published extensively (h-index 59 and over 11,000 citations). In 2012, he received the IBA Technology Award for his contributions to Li-ion battery materials. He has appeared as a patent litigation expert witness in Europe and North America. Currently, Dr. Barker acts as an Expert Panel member for the Faraday Institution and Advisory Board member for Australia’s storEnergy initiative.
Stefano Passerini is Professor at the Karlsruhe Institute of Technology and Director of the Helmholtz Institute Ulm. His research focuses on the basic understanding and development of materials for highenergy batteries and supercapacitors, with the goal to create sustainable energy storage systems from environmentally friendly and available materials and processes. He is an internationally recognized pioneer in the field of ionic liquids and the development of sodium-ion batteries.
Prof. Passerini received his PhD in Electrochemistry from Sapienza – Università di Roma in 1992. He then worked as a senior scientist at the University of Minnesota and later at ENEA (Italian National Agency for New Technologies, Energy and Environment). He has been a visiting scientist at Waseda University and the Universidade de São Paulo. Appointed Professor at the Karlsruhe Institute of Technology in 2014, he became Director of the Helmholtz Institute Ulm in 2018.
The co-author of more than 600 scientific papers (h-index of 103), a few book chapters, and several international patents, Prof. Passerini has been the Editor-in-Chief of the Journal of Power Sources since 2015. His work has been recognized with numerous accolades, including Fellow of The Electrochemical Society (2020) and International Society of Electrochemistry (2016). He has been a member of the Leopoldina Academy of Science since 2019. The ECS Battery Division gave him their Research Award in 2012.
Youngjin Ham recently received her PhD from the Department of Materials Science and Engineering at the Korea Advanced Institute of Science and Technology. Her research focused mainly on the development of strategic design principles for multidimensional electrodes toward practical organic batteries and biocatalytic CO2 reduction, as published in Energy & Environmental Science and Advanced Energy Materials. Throughout her PhD work, Dr. Ham pursued approaches spanning the engineering of electrode architectures via 3D nanopatterning techniques and molecular-level control of redox functionality using graphene-based materials. Her work has been highlighted in more than 30 media outlets and recognized by several awards, including the Korea Institute of Materials Science’s 2022 Best Academic Paper Award.
Mitchell Kaiser is a PhD student at the University of Washington (UW). He received his BS in Chemistry and ACS certification at UW in 2018. As an undergraduate in Prof. Daniel Gamelin’s UW lab, he studied the post-synthetic modification of cesiumbismuth-halide nanoparticles by anion exchange to achieve structures not accessible via direct hot-injection synthesis. Kaiser is now a member of Prof. Jun Liu’s lab, fabricating ultramicroelectrode devices from monolayer molybdenum disulfide. He uses a technique called quantum capacitance spectroscopy that he developed with Dr. Mengyu Yan to study the relationship of the electronic structure of pristine and modified electrode materials and the catalytic activity of the material for various reactions.
Julie Fornaciari recently completed her PhD in chemical engineering at the University of California, Berkeley under Prof. Alex Bell and Dr. Adam Weber. Her research centered on electrochemical energy conversion and storage of hydrogen gas. She spent the past five years working on low temperature water electrolysis, focused on multiscale transport in electrolytic devices, and on diagnostic experiments and characterization of these devices.
A first-generation college graduate, Dr. Fornaciari joined the US Department of Energy as a Hydrogen Shot Fellow in the Hydrogen and Fuel Cell Technology Office. In addition to her research, she is passionate about science communication and on bridging the gap between scientists and non-scientists. She has volunteered for various programs through the nonprofit Community Resource for Science, including the Bay Area Scientists Inspiring Students (BASIS) program; and was a writer and editor for the Berkeley Science Review; a Berkeley SciComm Fellow; and served on the ComSciCon Flagship Conference program committee.
Rachel Zhuojun Huang is a fifth-year PhD student at Stanford University. Her research at the intersection of polymer and electrochemistry focuses mainly on polymeric interface for lithium metal anodes and next generation safe polymeric electrolytes. She completed a BS in Materials Science and Engineering at the University of California, Berkeley.
Matthew Liu is a PhD student in the Chemical Engineering Department at Stanford University and a NASA Space Technology Graduate Research Fellow. Liu studies electrochemical reactive-separation processes to recover ammonia from nitrogenrich wastewaters as a member of William Tarpeh’s lab. His particular focus is on electrocatalytic nitrate reduction, which has led him to investigate systems driven by heterogeneous catalysis, molecular catalysis, and single atom catalysis. Liu is also interested in how the structure and composition of the microenvironment at the electrode-electrolyte interface directs nitrate reduction performance. As an undergraduate studying at the University of California, Berkeley and researching at Lawrence Berkeley National Laboratory, he also focused on energy and the environment, studying free-radical chemistry in aqueous aerosol under Frances Houle and electrochemical carbon dioxide reduction under Prof. Bryan McCloskey
The designation “Fellow of The Electrochemical Society” was established in 1989 for advanced individual technological contributions to the fields of electrochemistry and solid state science and technology, and for service to the Society. These members are recognized for scientific achievements, leadership, and active participation in the affairs of ECS. Each year, their peers choose up to 15 renowned scientists and engineers for this honor
Please join us in celebrating the 2022 Class of Fellows of The Electrochemical Society.
Michel Armand is Emeritus Researcher at the Centre National de la Recherche Scientifique (CNRS), and works at CICEnergiGUNE in Spain. After introducing the intercalation concept, Dr. Armand led the community with outstanding contributions to the field of battery electrochemistry with major industrial applications. His most important findings are solid state polymer electrolytes for Li metal polymer batteries which are now commercialized; new highly conductive salt families like LiTFSI (commercialized) and LiFSI for advanced electrolytes; and carbon-nanopainting of LiFePO4 leading to wide-scale commercialization of this olivine in electric vehicles and grid storage batteries.
Dr. Armand received his PhD in Physics from Université Joseph Fourier in 1978. He was a Senior Scientist at Lawrence Berkeley National Laboratory (1982–1983); Director of Research at CNRS starting in 1989; Professor at the Université de Montréal (1995–2004); and Director of the Joint CNRS-UdM International Laboratory on Electroactive Materials (2000–2004). Dr. Armand joined CIC-EnergiGUNE in 2011 as part of the Scientific Committee of the Electrochemical Storage, Batteries, and Supercaps area, while leading the creation of the Polymer Electrolyte Research Group, currently a leader in European research on solid state batteries.
Dr. Armand is the author or co-author of more than 500 publications (h-factor 95) with more than 69,500 citations, as well as contributing to 16 book chapters. Among the many honors he has received are the 2010 Galileo Award; 2000 ECS Europe Section Volta Award; 1995
International Society for Electrochemistry Pergamon Medal; 1988 ECS Battery Division Award; 1985 Royal Society Faraday Division Award; and CNRS 1978 Bronze and 1989 Silver Medals. Stanford University included him on their 2019 List of 100,000 Top Scientists. The Clarivate Highly Cited Researchers List included him for multiple years. In 2020, the Society published the JES Focus Issue on Challenges in Novel Electrolytes, Organic Materials, and Innovative Chemistries for Batteries in Honor of Michel Armand
Perla Balbuena is Professor of Chemical Engineering and holds the Mike O’Connor Chair I at Texas A&M University, where she also holds joint appointments in Chemistry and Materials Science and Engineering. Her expertise is in first principles analysis of materials and interfacial reactions for catalysis, electrocatalysis, and battery materials. Her work has contributed to elucidating crucial interfacial reaction mechanisms such as the formation and growth of solid-electrolyte interphases in advanced battery technologies.
Prof. Balbuena completed a PhD in Chemical Engineering at the University of Texas at Austin in 1996 after finishing a BS in 1973 at Universidad Tecnológica Nacional, Argentina, and an MSE in 1983 at the University of Pennsylvania. Included in the numerous awards she has received throughout her career are the 2020 Texas A&M Engineering Experiment Station (TEES) Research Impact Award; 2017 Engineering Genesis Award; 2002 University of South Carolina Young Investigator Award; NSF CAREER awards (1997–1999); and fellowships from TEES, the American Association for the Advancement of Science, and the American Institute of Chemical Engineers. Prof. Balbuena serves as an Associate Editor for the Journal of The Electrochemical Society and ECS Advances
Gerbrand Ceder holds the Samsung Distinguished Chair in Nanoscience and Nanotechnology at the University of California, Berkeley, with a joint appointment as Senior Faculty Scientist at Lawrence Berkeley National Laboratory. There he combines theory, computation, and experiments to develop novel materials for energy storage. For over 25 years, Prof. Ceder has worked in Li-ion technology, and more recently also on alternative energy storage approaches such as solid state batteries, Na-ion, and Mg-ion devices. He is a co-inventor of the disordered rocksalt cathode materials (DRX) for Li-ion technology and was active in formulating the US Materials Genome Initiative.
Prof. Ceder began his academic career studying at the Katholieke Universiteit Leuven, and then received his PhD in Materials Science from UC Berkeley. After 24 years as a professor at the Massachusetts Institute of Technology (MIT), he returned to UC Berkeley. The author of more than 500 papers (h-index 154), he holds more than 50 US and foreign patents. Prof. Ceder is a member of the US National Academy of Engineering, Royal Flemish Academy of Belgium for Science and Art, and American Academy for the Arts and Sciences. He is a Fellow of the Materials Research Society (MRS); Metals, Minerals, and Materials Society; and American Physical Society; and received the 2017 National Academy of Engineering Award; 2016 International Battery Research Award; 2016 MRS Materials Theory Award; 2009 MRS Gold Medal; 2007 MIT School of Engineering Graduate Teaching Award; and 2004 ECS Battery Research Award.
(continued on next page)
Wilson K. S. Chiu is Professor of Mechanical Engineering at the University of Connecticut. In collaboration with an international team of scientists and engineers, Prof. Chiu pioneered new synchrotron-based X-ray imaging and spectroscopy methods to elucidate complex 3D electrochemical and transport processes in energy materials. Applications include solid oxide and polymer electrolyte membrane fuel cells and electrolyzers, mixed ionic-electronic conducting separation membranes, battery and solar materials, ceramic waste forms, and molten salts.
Prof. Chiu earned his PhD in Mechanical Engineering from Rutgers University. He has held visiting appointments at Columbia University and the Università degli Studi di Napoli Federico II. Prof. Chiu has published seven book chapters/special volumes, 126 journal articles, and 203 conference articles/abstracts. He has served on beam line advisory, science review, and proposal review committees for synchrotron light sources. Among his honors, Prof. Chiu is an elected Fellow of the American Society of Mechanical Engineers (ASME) and American Society of Thermal and Fluids Engineers. He was awarded an Otto Mønsted Guest Professorship; United Technologies Corporation Professorship in Engineering Innovation; and elected to the Connecticut Academy of Science and Engineering. He received the Office of Naval Research Young Investigator Award, Army Research Office Young Investigator Award, and the NSF CAREER Award. Prof. Chiu is the Editor-in-Chief of the ASME Journal of Electrochemical Energy Conversion and Storage, guest editor for the Journal of The Electrochemical Society, and has served as an associate editor and on the editorial board of several other journals. He is the lead organizer of the triannual ECS Symposium on Heterogeneous Functional Materials (HeteroFoaMs) for Energy Conversion and Storage
Andrew Hoff is Professor and Graduate Director of the Electrical Engineering Program at the University of South Florida (USF). His research focuses on diverse applications of plasma processing in the material and biomedical realms. These include afterglow chemical processes, corona-Kelvin metrology, and drug and DNA molecular delivery for cancer treatment.
Prof. Hoff received his PhD in Electrical Engineering from Pennsylvania State University in 1988 and joined the faculty at USF that year as a founding member of the Center for Microelectronics Research. Prof. Hoff is Past Director of the CMR Metrology Laboratory and Co-Director of the state workforce training Agile Initiative (1998–2004). He directed or collaborated on NSF-ATE workforce development programs in Florida from 2002 to 2018. Prof. Hoff received a 2013 Outstanding Engineering Educator award from the Florida West Coast IEEE and the 2000 Pioneering Award for Non-Contact Metrology.
Prof. Hoff joined ECS as a student member in the late 1970s and began attending conferences in the late 1980s. He has served the Society through the Electronics and Photonics division (EPD) since he joined it in 2003; as member of the Executive Committee (2004–present); symposium organizer; ECS Publication Committee division representative (2005–2009); Interface Advisory Board member (2005–2011); and EPD Division Secretary (2007–2009), Vice Chairman (2009–2011), Chairman (2011–2013), and Past Chairman (2013–2015).
Gao Liu is Senior Scientist and Group Leader of the Applied Energy Materials Group at Lawrence Berkeley National Laboratory (LBNL) where he specializes in energy storage R&D. With over 20 years of experience in developing materials and system engineering for electrical energy storage, Dr. Liu has led research projects for industry and the US Department of Energy (DOE). His research combines synthetic chemistry, composite engineering, and electrochemistry to solve interdisciplinary problems in energy generation, storage, and usage. Dr. Liu’s lab uses advanced diagnostics to understand fundamental and critical issues in energy systems, and synthetic techniques to develop new materials that improve overall system performance. His ongoing research in battery and energy storage covers electrode binder, silicon, sulfur, and lithium metal materials, electrode engineering, electrolytes and additives, and solid state conductors. Besides energy storage research, Dr. Liu also performs materials and engineering research in building resiliency, circular economy, and advanced manufacturing.
Dr. Liu received a BS from Beijing University in 1993, and a PhD in Chemistry from Michigan State University in 2001. He has more than 180 peer-reviewed publications and more than 20 granted patents. His work has garnered numerous awards, including the 2013 and 2015 R&D 100 Award, 2014 FMC Corporation Scientific Achievement Award, and University of California 2011 Discovery Grant.
Brett Lucht is Professor of Chemistry at the University of Rhode Island. His research focuses on novel electrolytes and electrolyte electrode interfaces for lithium-ion battery applications, which include extending the calendar life and improving low temperature properties, the performance of novel high capacity anodes such as lithium metal and silicon, and the performance of high voltage cathode materials. He conducts basic science and applied research, and collaborates extensively with industrial partners. Prof. Lucht has mentored more than 100 students, including more than 40 graduate students, 30 undergraduate students, and 20 postdoctoral fellows, along with several visiting students.
Prof. Lucht received a BS in Chemistry from the University of Puget Sound in 1991 and PhD in Chemistry in 1996 from Cornell University. He completed postdoctoral research at the University of California, Berkeley before beginning work at the University of Rhode Island in 1998. Prof. Lucht was promoted to Associate Professor with tenure in 2002 and Professor in 2006. He is Associate Editor for the Journal of The Electrochemical Society and ECS Advances as well as Vice Chair of the ECS Battery Division. He has published more than 170 manuscripts (h-index 64) and two book chapters, and holds nine patents. Prof. Lucht has been an invited or keynote speaker for more than 100 companies, universities, national laboratories, and international conferences.
Janine Mauzeroll is Professor of Chemistry at McGill University. There she heads a research group of 20 graduate students, undergraduates, postdoctoral fellows, and research associates focused on topics ranging from electrochemistry in organic and biological media to electronically conducting polymers. Her group systematically combines experimental and numerical simulation approaches to study
electrochemical systems in the fields of biology, corrosion, batteries, electrolysis, electrocatalysts, and electrosynthesis.
Prof. Mauzeroll completed her BS at McGill in 1999, followed by a PhD in 2004 from the University of Texas at Austin (2004) with Allen J. Bard. She completed a postdoctoral fellowship in 2005 in Jean-Michel Savéant’s laboratory. After serving as Assistant and then Associate Professor at the Université du Québec à Montréal from 2005 to 2011, Prof. Mauzeroll returned to her alma mater, McGill, as Associate Professor in 2012. She received the 2015 Chemical Society of Canada Fred Beamish Award and 2003 ECS Summer Fellowship.
A Technical Editor for the Journal of The Electrochemical Society Organic and Bioelectrochemistry topical interest area since 2016, Prof. Mauzeroll was recently appointed Technical Editor for the Organic and Bioelectrochemistry topical interest area of ECS Advances. Prof. Mauzeroll has also served on the editorial boards of Analytical Chemistry and ChemElectroChem. She chaired the 2020 Gordon Conference on Electrochemistry and has given more than 100 presentations with 60 invitations to give talks at major universities, plenary lectures, and numerous invited lectures. She is active in her scientific community with 13 conferences (organized or chaired) and service on 26 scientific committees (including the Chair of the NSERC Chemistry Liaison Committee and a Member of NSERC-Discovery Grant Committee on Chemistry).
Nguyen Minh is presently with the Center for Energy Research at the University of California, San Diego (UCSD). An internationally known expert on solid oxide fuel cells (SOFCs), solid oxide electrolysis cells (SOECs), and related technologies, his current SOFC research at UCSD focuses on basic and applied science and engineering studies on properties, phenomena, and designs key to SOFC/SOEC stack technology and development of advanced concepts. Over the last 30 years, he has dedicated his research to the development of SOFCs/SOECs and new energy systems. His experience covers a full spectrum of industrial/product R&D areas, ranging from technology assessment, strategy and roadmap formulation, and fundamental and engineering study to materials processes, and manufacturing development, system design and operation, prototype demonstration, and cost/market analysis.
Before UCSD, Dr. Minh was Chief Scientist/Manager, Fuel Cells at General Electric and Honeywell/AlliedSignal, and a Group Leader/Staff in Electrochemical Technology at Argonne National Laboratory. He is the author/co-author of the book, Science and Technology of Ceramic Fuel Cells, and nine book chapters, some 150 published technical articles on SOFCs and related technologies, and 21 patents. His review paper, “Ceramic Fuel Cells,” published in the Journal of the American Ceramic Society in 1993, is a classic widely cited in the literature and translated into several languages. Dr. Minh has received awards that include the 2007 American Society of Mechanical Engineers Francis T. Bacon Medal; 2017 Fuel Cell Seminar & Energy Exposition Award; and 2021 Electrochemical Society H-Temp Division Subhash Singhal Award.
Deborah J. Myers is a Senior Chemist and Leader of the Hydrogen and Fuel Cell Materials Group in Argonne National Laboratory’s Chemical Sciences and Engineering Division. She is Co-Lead of the US Department of Energy (DOE) Hydrogen and Fuel Cell Technologies’ Electrocatalysis Consortium (ElectroCat); Deputy Director for DOE’s “Hydrogen from the Next Generation of Electrolyzers of Water”
Consortium (H2NEW); and Deputy Director for DOE’s “Million Mile Fuel Cell Truck” consortium (M2FCT). Dr. Myers’ expertise is in the development, characterization, and understanding of fuel cell and electrolyzer electrocatalysts and electrodes. Her present research areas include: in situ and operando X-ray scattering, spectroscopy, and ex situ X-ray tomography techniques to understand the active sites, degradation mechanisms, and factors controlling the performance and durability of fuel cell and electrolyzer catalysts and electrodes; and high-throughput approaches to develop and implement platinum group metal-free oxygen reduction and oxygen evolution electrocatalysts for fuel cells and electrolyzers.
Dr. Myers received her PhD in 1989 from the University of Illinois, Urbana-Champaign with Professor Andrzej Wieckowski, specializing in physical electrochemistry. Her postdoctoral research, with Dr. Zoltan Nagy in the Argonne National Laboratory’s Chemical Technology Division, focused on synchrotron X-ray reflectivity studies of electrode-electrolyte interfaces. Dr. Myers’ subsequent staff position in Argonne’s Fuel Cell Section focused on developing catalysts and electrolytes for solid oxide and direct methanol fuel cells and water-gas shift catalysts for hydrogen production. In 2001, she became Group Leader for Shift Catalysis and, subsequently, for Solid Oxide Fuel Cells, and in 2003, assumed her current position. Among the awards she has received are the 2004 and 2020 DOE Hydrogen Program R&D Award; 2017 Argonne National Laboratory Director’s Award; 2004 and 2016 Argonne National Laboratory Pacesetter Award; 2015 US Car Award for the Fuel Cell Technology Team; and 2000 DOE National Laboratory Fuel Cell R&D Award. Dr. Myers is the author of more than 80 publications and two book chapters with over 8,000 citations (15 publications with more than 100 citations, including one with more than 3,000 citations), and more than 200 presentations (41 invited). She holds eight US patents.
James (Jamie) Noël is Associate Professor of Chemistry and Faculty Scholar at Western University. His research group works on corrosion and related electrochemical topics in several areas, with a focus on determining the corrosion lifetimes of materials potentially involved in the safe, permanent disposal of nuclear fuel waste, including both candidate container materials (copper, steel, titanium, and nickel-based superalloys) and nuclear fuels (uranium dioxide and thoria).
Prof. Noël and his team helped to resolve the key corrosion questions required for the Government of Sweden to license a nuclear fuel waste repository in 2022 in Sweden. Prof. Noël’s other interests include development of new experimental methods (e.g., combined electrochemical/neutron scattering approaches) and custom apparatus, such as vessels for electrochemical experiments in extreme environments (e.g., supercritical water, intense radiation fields, deep undersea, and under ultra-high vacuum).
Prof. Noël earned BS and MS degrees in Chemistry from the University of Guelph and a PhD in Chemistry from the University of Manitoba. He worked at Ontario Hydro and then Atomic Energy of Canada Ltd. before joining Western University as Research Scientist with Dave Shoesmith. When Shoesmith prepared for retirement, Prof. Noël was appointed to lead the research group. He is Chair of the ECS Corrosion Division and a former Chair of the ECS Education Committee and ECS Canada Section. He teaches two popular ECS Short Courses on the fundamentals of electrochemistry. Prof. Noël was named a 2022 Faculty Scholar at Western University (see page 34) and received the Canada Section’s 2019 R.C. Jacobsen and 2003 Lash Miller Awards.
Elizabeth (Lisa) J. Podlaha-Murphy is Chair of the Chemical and Biomolecular Engineering Department at Clarkson University. Her research experience and expertise lie in the area of electrodeposition of novel alloy and composite materials for a variety of applications, including water splitting for hydrogen generation, corrosionresistant surface coatings, nanostructured materials for micro and nanodevice components, and modeling battery and electrochemical systems.
In 1986, Prof. Podlaha received her BS and MS in Chemical Engineering with an Honor’s Program distinction from the University of Connecticut, followed by employment at IBM. Inspired by her IBM colleagues doing cutting-edge research, she returned to school, earning a PhD in Chemical Engineering from Columbia University. She completed a postdoctoral position in the Department of Materials Science at the École Polytechnique Fédérale de Lausanne. Her first academic appointment was at Louisiana State University as an Assistant/tenured Associate Professor in Chemical Engineering (1998–2007), and at Northeastern University as a tenured Associate/ full Professor (2007–2017). As part of NSF, DOE, NIH, and DARPA funded projects, including the NSF CAREER Award, Prof. Podlaha has directed 26 graduate (20 PhD and 6 MS) and more than 40 undergraduate students in electrochemical research in the lab. She has authored or co-authored 84 peer reviewed journal publications (half of those in the Journal of The Electrochemical Society and Electrochemical and Solid State Letters), 24 proceedings papers, one book chapter, and four patents. Prof. Podlaha is the Treasurer of The Electrochemical Society (2022–2026). She was past Chair of the ECS Electrodeposition Division (ELDP) (2015–2017), and held the preceding officer positions of Member at Large, Treasurer, Secretary, and Vice Chair. Prof. Podlaha has served on many Society committees, including the Membership Committee, and organized, chaired, and co-chaired symposia. In 2019, she helped co-advise the newly formed ECS Student Chapter at Clarkson University.
Vijay Ramani is the Vice Provost for Graduate Education and International Affairs at Washington University in St. Louis (WUSTL). He holds the Roma B. and Raymond H. Wittcoff Distinguished University Professorship and serves as a Professor in the Department of Energy, Environmental, and Chemical Engineering. His research interests lie at the confluence of electrochemical engineering, materials science, and renewable energy technologies, with a focus on electrochemical energy conversion and storage (low temperature fuel cells, electrolyzers, and redox-flow batteries). Research directions in his group include multi-functional electrolyte and electrocatalyst materials for electrochemical systems, analyzing the source and distribution of overpotential in electrochemical systems, mitigating component degradation in electrochemical devices, and in situ diagnostics to probe electrochemical systems.
Prof. Ramani has a PhD from the University of Connecticut and BE from Annamalai University, both in Chemical Engineering. The National Science Foundation, Department of Energy, Office of Naval Research, and Advanced Research Projects Agency–Energy have funded his research with mechanisms including a 2009 NSF CAREER Award and 2010 ONR Young Investigator Award. He received the
2010 3M Non-tenured Faculty Award, and 2012 ECS ETD Division Supramaniam Srinivasan Young Investigator Award. Prof. Ramani has been affiliated with ECS since 2001 and is a Life Member. Prof. Ramani has served as co-editor of ECS Interface (2013–2017) and is a Past Chair of the ECS IE&EE Division and AIChE Area 1E.
Yasuhiro Shimizu is Professor and Dean of the Graduate School of Engineering at Nagasaki University. His research focuses on chemical sensors, including various kinds of gas sensors capable of detecting humidity, oxygen, VOCs, and odors, by employing several detection principles. Most recently, his work has been directed at developing gas sensors for use in safety and health care.
Prof. Shimizu received his PhD in Engineering in 1987 from Kyushu University after completing his BS in Applied Chemistry there in 1980. He joined the faculty of Nagasaki University in 2005. The Electrochemical Society of Japan (ECSJ) recognized his scientific contributions and service by appointing him as a Fellow in 2020. Other awards include the 2021 ECS Sensor Division Outstanding Achievement Award; 2008 ECSJ Scientific Achievement Award; 2001 Seiyama Award of the Japan Association of Chemical Sensors (JACS); 2001 and 2005 ECSJ Distinguished Paper Awards; and 1992 ECSJ Sano Award for a young distinguished researcher. He has served as Chair of the Asia/Pacific Region in the Executive Steering Committee of the International Meeting on Chemical Sensors (since 2016); Chair of the International Steering Committee of the Asian Conference on Chemical Sensors (since 2017); President of the Japan Association of Chemical Sensors (an ECSJ expert division) (2015–2016); Editor of Sensors and Actuators B: Chemical (2008–2018), and Co-Editorin-Chief of Sensors and Actuators B: Chemical (since 2018).
Shunpei Yamazaki is the Founder and President of Semiconductor Energy Laboratory Co., Ltd. He is also General Managing Director of the Foundation for Promotion of Material Science (MST), and President and Founder of the Kato & Yamazaki Educational Foundation (KYEF). His current research focuses on the research and development of an oxide semiconductor. Dr. Yamazaki received his BE (1965) and ME (1967) from Doshisha University. While a PhD student there, he invented a non-volatile memory known as “flash memory.” After completing his PhD in 1971, Dr. Yamazaki joined TDK Corporation (formerly known as Tokyo Denki Kagaku Kogyo K.K). In 1980, he founded Semiconductor Energy Laboratory Co., Ltd. (SEL) and assumed the position of president. Doshisha University awarded Dr. Yamazaki honorary degrees in 2011 and the title of “Friends of Doshisha” in 2015. He has received the 2018 American Ceramic Society W. David Kingery Award; 2015 SID Special Recognition Award; 1997 Japanese Prime Minister’s Medal with Purple Ribbon; and 1984 Richard M. Fulrath Award. Synectics ranked him 58th in the “Top 100 Living Geniuses Survey” in 2007. Dr. Yamazaki has published or co-published more than 500 papers and conference presentations. He holds the 2004, 2011, and 2016 Guinness World Records for “holding the most patents credited as inventor”—11,353 as of June 2016. In 2005, USA Today named him the most prolific inventor in history. He is a member of the Royal Swedish Academy of Engineering Sciences and Academician of World Academy of Ceramics.
H. E. M. Huissein, Coventry, Warwickshire, UK
AJinkwang Hwang, Kyoto, Kyoto, Japan
Duha Ahmed, Baghdad, Baghdad, Iraq
Michael Armstrong, Danville, CA, USA
BAldona Balčiūnaitė, Vilnius, Vilnius County, Lithuania
Victor Beck, Livermore, CA, USA
Rana Biswas, Ames, IA, USA
Timothy Bradow, Lakewood, CO, USA
Valentín Briega Martos, Erlangen, BY, Germany
Sofie Cambré, Antwerp, East Flanders, Belgium
Qing Cao, Urbana, IL, USA
Sharon Chen, Mississauga, ON, Canada
Ju-hsiang Cheng, Maple Ridge, BC, Canada
Jun Young Cheong, Bayreuth, BY, Germany
Wendy Chung, Montréal, QC, Canada
Stefano Cinti, Naples, Campania, Italy
Brian Collins, Holly Springs, NC, USA
Peter Csernica, Mountain View, CA, USA
Celia Cunningham, Detroit, MI, USA
Christian Désilets, Shawinigan, QC, Canada
Sergei Devyatkin, Kyiv, Kyiv, Ukraine
Oleg Dimitriev, Kyiv, Kyiv, Ukraine
Fang Dong, Pasadena, TX, USA
Jan Dubowski, Sherbrooke, QC, Canada
Michael Forbes, North Vancouver, BC, Canada
Liliya Frolova, Dnipro, Dnipropetrovsk, Ukraine
Kuan-Zong Fung, Tainan, Tainan, Taiwan
Prashanth Ganeshram, Auburn Hills, MI, USA
Iñigo Garbayo, Sanguesa, Navarra, Spain
Irina Goncharova, Kyiv, Kyiv, Ukraine
Mikaela Gorlin, Cambridge, MA, USA
Yvonne Grunder, Liverpool, England, UK
Rohini Gupta, Hillsboro, OR, USA
Lucas Hof, Montréal, QC, Canada
Jong Wook Hong, Ulsan, Yeongnam, Republic of Korea
Bo Hu, Xi’an, Shaanxi Province, China
Jiazhi Hu, Troy, MI, USA
Zachary Hudson, Vancouver, BC, Canada
Michael Hughes, North Vancouver, BC, Canada
Yutaka Ie, Ibaraki, Osaka, Japan
Timo Jacob, Ulm, BW, Germany
Haipeng Ji, Zhengzhou City, Henan, China
Hannes Jónsson, Reykjavík, Capital Region, Iceland
Jung Min Joo, Busan, South Gyeongsang, Republic of Korea
CSoon Hyung Kang, Gwangju, Gyeonggi-do, Republic of Korea
Pinar Karayaylali, Augsburg, BY, Germany
Amit Kathpalia, Delhi, DL, India
Santosh KC, San Jose, CA, USA
Michael Kennedy, West Palm Beach, FL, USA
Chang Soo Kim, Seoul, Gyeonggi-do, Republic of Korea
Daekeun Kim, Seoul, Gyeonggi-do, Republic of Korea
Moonil Kim, Daejeon, Hoseo, Republic of Korea
DTae-Gon Kim, Ansan, Gyeonggi-do, Republic of Korea
Tsunenobu Kimoto, Kyoto, Kyoto-fu, Japan Brian Kirkpatrick, Allen, TX, USA
Andrei Kolmakov, Gaithersburg, MD, USA
Valerii Kotok, Dnipro, Dnipropetrovsk, Ukraine
Stanislav Kovalyov, Dnipro, Dnipropetrovsk, Ukraine Ivar Kruusenberg, Tallinn, Estonia, Estonia Serhii Kuleshov, Kyiv, Kyiv, Ukraine
Seong Jung Kwon, Seoul, Gyeonggi-do, Republic of Korea
Robert Lapierre, Bloomfield Township, MI, USA
Linh Le, Long Island City, NY, USA
Phung Le, Richland, WA, USA
Carlos Leon y Leon, State College, PA, USA
Victor Leung, Vancouver, BC, Canada
Phoebe Li, Port Coquitlam, BC, Canada
Andrea Listorti, Bari, Puglia, Italy
HQuanlin Liu, Beijing, Beijing, China
Yijin Liu, Menlo Park, CA, USA
Qingye Lu, Calgary, AB, Canada
Xiaoyan Luo, Burnaby, BC, Canada
MXiaowei Ma, Langley, BC, Canada
Philipp Maass, Osnabrück, NI, Germany
Gabriele Magna, Rome, Lazio, Italy
Elisha Martin, Bathgate, Scotland, UK
Hisayoshi Matsushima, Sapporo, Hokkaido, Japan
Prantik Mazumder, Ithaca, NY, USA
Dmitry Medvedev, Ekaterinburg, Sverdlovsk, Russia
Olha Medvezhynska, Kyiv, Kyiv, Ukraine
Sajjad Husain Mir, Dublin, Leinster, Ireland
Yuhei Miyauchi, Kyoto, Kyoto-fu, Japan
Chris Moffitt, Corpus Christi, TX, USA
Chochanon Moonla, La Jolla, CA, USA
Max Mullen, Longmeadow, MA, USA
Krystal Munoz, Cumberland, RI, USA
Azusa Muraoka, Bunkyo-ku, Tokyo, Japan
NAtsushi Nakajima, Yokohama, Kanagawa, Japan
Teppei Nakano, Leuven, Flemish Brabant, Belgium
Inessa Novoselova, Kyiv, Kyiv, Ukraine
OYohei Okada, Fuchu, Tokyo, Japan
Francesco Orfino, Port Moody, BC, Canada
Sai Kiran Oruganti, Ulsan, Fukuoka, Japan
Masayuki Otsuji, Hikone City, Shiga, Japan
Taiichi Otsuji, Sendai, Miyagi, Japan
Keigo Otsuka, Bunkyo-ku, Tokyo, Japan
Muthukumaran Packirisamy, Montréal, QC, Canada
Mohammad Javad Parnian, Calgary, AB, Canada
Philippe Perret, Boucherville, QC, Canada
Andreas Podias, Petten, North Holland, Netherlands
Yuliya Polishchuk, Dnipro, Dnipropetrovsk, Ukraine
Pragati Poudel, Rochester, NY, USA
Guannan Qian, Concord, CA, USA
Alasdair Robertson, Bathgate, Scotland, UK
John Rogers, Evanston, IL, USA
Ghuzanfar Saeed, Busan, South Gyeongsang, Republic of Korea
Guido Schmitz, Stuttgart, BW, Germany
Franz Selbmann, Chemnitz, SN, Germany
Rinky Sha, Kalyani, WB, India
Olesia Shmychkova, Dnipro, Dnipropetrovsk, Ukraine
Irina Sknar, Dnipro, Dnipropetrovsk, Ukraine
Yuri Sknar, Dnipro, Dnipropetrovsk, Ukraine
Zhen Song, Beijing, Beijing, China
Steven Sparkowich, Vancouver, WA, USA
Bryan Steinhoff, Ann Arbor, MI, USA
Mohammad Hossein Tahmasebi, Halifax, NS, Canada
Chixia Tian, Christiansburg, VA, USA
Toan Tran, Da Nang, Central Vietnam, Vietnam
Alexander Urban, New York, NY, USA
Hui Wang, Louisville, KY, USA
Lihui Wang, Berkeley, CA, USA
Ying Wang, Baton Rouge, LA, USA
Karl-Michael Weitzel, Marburg, HE, Germany
Bryan Wygant, Albuquerque, NM, USA
Younan Xia, Atlanta, GA, USA
Hisato Yamaguchi, Los Alamos, NM, USA
Yasutaka Yamaguchi, Suita, Osaka, Japan
Kazuhiro Yanagi, Tokyo, Tokyo, Japan
Takashi Yanagishita, Hachioji, Tokyo, Japan
Huaxiang Yang, Baton Rouge, LA, USA
Chae-Ho Yim, Ottawa, ON, Canada Wonseok Yoon, Southborough, MA, USA
Shengli Zhai, Edmonton, AB, Canada
Dong Zhao, San Jose, CA, USA
Leyi (Roy) Zhao, Langley, BC, Canada
Shuai Zhao, Berkeley, CA, USA
Songrui Zhao, Montréal, QC, Canada
Weijia Zhou, Jinan, Shandong, China
Gaohua Zhu, Ann Arbor, MI, USA
Aref Aasi, Worcester, MA, USA
Pedram Abbasi, La Jolla, CA, USA
Mohamed Abdelrahman, Pittsburgh, PA, USA
Ibrahim Abdullahi, Rolla, MO, USA
Michael Addo, Johnson City, TN, USA
Albert Adserias, Burnaby, BC, Canada
Khantesh Agrawal, Bangalore, KA, India
Hoda Ahmed, Montréal, QC, Canada
Masoud Akbari, Waterloo, ON, Canada
Md Younus Ali, Hamilton, ON, Canada
Mehrzad Alizadeh, Minoh, Osaka, Japan
Elizabeth Allan-Cole, Boulder, CO, USA
Papa Amoah, Newport News, VA, USA
Meichun An, Ithaca, NY, USA
Yuki Ando, Hamilton, ON, Canada
Eleni Andreou, Glasgow, Scotland, UK
Fabusuyi Aroge, Surrey, BC, Canada
Tamil Arumugam, Sivagangai, TN, India
Kyung Taek Bae, Daejeon, Hoseo, Republic of Korea
Mohammad Bahzad, Knoxville, TN, USA
Mohammed Bazaid, Atlanta, GA, USA
Diana Beltran, Pittsburgh, PA, USA
David Bruce, Norman, OK, USA
Sebastian Büchele, Berg, BW, Germany
Sven Burke, Pittsburgh, PA, USA
Quinn Carvalho, Corvallis, OR, USA
Marco Cetina-Dorantes, Mérida, Yucatán, México
Eric Chadwick, Heidelberg, BW, Germany
Patatri Chakraborty, Buffalo, NY, USA
Michael Chan, Brooklyn, NY, USA
Tsewei Chen, London, England, UK
Zijie Chen, Ann Arbor, MI, USA
Chao-Ching Chiang, Gainesville, FL, USA
Basudev Choudhury, Mumbai, MH, India
Anna Claire, Andover, MN, USA
Morgan Clark, Ames, IA, USA
Saida Cora, Boston, MA, USA Giovanni Crivellaro, Padova, Veneto, Italy
Nicholas D’Antona, Eugene, OR, USA
Casey Davis, Boulder, CO, USA Zhiping Deng, Edmonton, AB, Canada
Astou Diop, Sherbrooke, QC, Canada
Sevde Doğruer Erkök, Miami, FL, USA
Elizabeth Donkor, Stillwater, OK, USA
Kelsey Duncan, Burnaby, BC, Canada Josephine Dykstra, Detroit, MI, USA
Heigo Ers, Tartu, Tartumaa, Estonia Amir Esfahani, Montréal, QC, Canada
Rida Farhat, Vancouver, BC, Canada
Stanley Feeney, Dover, NH, USA
Kara Ferner, Pittsburgh, PA, USA
Kathryn Ford, Christchurch City, Canterbury, New Zealand
Karli Gaffrey, Trenton, MI, USA
Zhi Gao, Auburn Hills, MI, USA
Mayank Garg, Munich, BY, Germany Kaitlin Garman, Boulder, CO, USA Sakshi Gautam, Pullman, WA, USA
Adjmal Ghaur, Düsseldorf, NRW, Germany
Ali Ghazanfar, Novara, Piedmont, Italy
Zahed Ghelichkhah, Bethlehem, PA, USA Hooman Gholamzadeh, Kingston, ON, Canada
Bon Seung Goo, Daejeon, Hoseo, Republic of Korea
Adrian Grzedowski, Vancouver, BC, Canada
Raymond Guan, Toronto, ON, Canada
Jia Guo, Aalborg, North Jutland, Denmark
Priyanka Gupta, New Delhi, DL, India
HChaeyoung Ham, Toronto, ON, Canada
Johan Hamonnet, Christchurch City, Canterbury, New Zealand
Umu Hanifah, Ikoma, Nara, Japan
Wei Hao, Austin, TX, USA
Md. Mehadi Hassan, Calgary, AB, Canada
Xinzi He, College Park, MD, USA
Alexander Heenan, Christchurch, Canterbury, New Zealand
Dominic Hey, Cambridge, England, Great Britain
Sarah Higgins, Monifieth, Angus, Scotland, UK
Lucas Hille, Garching, BY, Germany
Jesse Hinricher, Pipestone, MN, USA
Wei-Chi Huang, Zhongli, Taoyuan, Taiwan
Khang Huynh, Rapid City, SD, USA
John Hymel, Atlanta, GA, USA
Mohamed Nazmi Idros, St Lucia, Queensland, Australia
Bahar Iranpour, Vancouver, BC, Canada
JGeorgina Jahan, Doral, FL, USA
Sukanya Jankhunthod, Muang, Nakhon Ratchasima, Thailand
Syeda Ramin Jannat, St Albans, Hertfordshire, UK
Dasol Jin, Seoul, Gyeonggi-do, Republic of Korea
Bjorto Joensen, Stanford, CA, USA
Cyril Jonathan W, Salem, TN, India
M. Jothibas, Mayiladuthurai, TN, India
Kätlin Kaare, Tallinn, Estonia, Estonia
Chifaa Kaddouri, Montréal, QC, Canada
Keerakit Kaewket, Muang, Nakhon Ratchasima, Thailand
Pascal Kaiser, Erlangen, BY, Germany
Uddipana Kakati, Philadelphia, PA, USA
Hyeong Cheol Kang, Seoul, Gyeonggi-do, Republic of Korea
Mavis Kang, Singapore
Angela Kapitanović, Zagreb, Zagreb, Croatia
Aaron Kaufman, Eugene, OR, USA
Annabelle Kilham, North Vancouver, BC, Canada
Beom-Seok Kim, Cheonan-si, Chungcheongbuk-do, Republic of Korea
Dongyeon Kim, Daejeon, Hoseo, Republic of Korea
Mijin Kim, Busan, Gyeongsangnam-do, Republic of Korea
Seojin Kim, St Andrews, Fife, Scotland, UK
Yuzu Kobayashi, Wako-shi, Saitama, Japan
Leya Kober, Toronto, ON, Canada
Nattakan Kongkho, Muang, Nakhon Ratchasima, Thailand
Crystal Kotan, Las Vegas, NV, USA
YuPing Ku, Erlangen, BY, Germany
Deepak Kumar, Agra, UP, India
Ravindra Kumar, Pune, MH, India
Mayuri Kushare, Golden, CO, USA
Tobias Kutsch, Garching, BY, Germany
Taehui Kwon, Seoul, Gyeonggi-do, Republic of Korea
Diego Landi, Miami, FL, USA
Eunjin Lee, Daejeon, Hoseo, Republic of Korea
Hojae Lee, Seoul, Gyeonggi-do, Republic of Korea
Kyungbin Lee, Atlanta, GA, USA
Youngju Lee, Saint Louis, MO, USA
Valerie Lefebvre, Montréal, QC, Canada
Danielle Lehto, Eau Claire, WI, Country
Anita Li, Ann Arbor, MI, USA
Jian-Sian Li, Gainesville, FL, USA
Guntae Lim, Daejon City, Hoseo, Republic of Korea
Jiawei Liu, Pittsburgh, PA, USA
Matthew Liu, Stanford, CA, USA
Siyi Liu, Huntsville, AL, USA
Alice Llewellyn, London, England, UK
Björn Lönn, Gothenburg, Västergötland, Sweden
Jeremy Lowen, Birmingham, West Midlands, UK
Jiayao Lu, Vancouver, BC, Canada
Kyle Ludwig, Berwyn Heights, MD, USA Norman Luu, Chicago, IL, USA
Vaishali Maheshkar, Buffalo, NY, USA
Tanushree Mandal, Galway, Connacht, Ireland
Sophie McArdle, Christchurch, Canterbury, New Zealand
Alexander McLeod, Vancouver, BC, Canada
Dylan McNally, Boulder, CO, USA
Meyssoune Meriem, Tazoult, Batna, Algeria
Juan Mesa, Pittsburgh, PA, USA
Alexandra Meyer, EggensteinLeopoldshafen, BW, Germany
Harry Michael, London, England, UK
Dean Miller, Stanford, CA, USA
Anna Mills, Oak Ridge, TN, USA
Maja Milosevic, Erlangen, BY, Germany
Maria Minichova, Erlangen, BY, Germany
Janhavi Mishra, Katpadi, TN, India
Viswanathan Mohandoss, Waterloo, ON, Canada
Soumyadip Mondal, Klosterneuburg, Niederösterreich, Austria
Jun-yeob Moon, Seoul, Gyeonggi-do, Republic of Korea
Polina Morozova, Moscow, Russia
Adrian Mularczyk, Eindhoven, North Brabant, Netherlands
James Murawski, London, Surrey, UK
Mohhamed Arshad Muzibur Raghuman, Madurai, TN, India
Deeksha N. V. N., Bengaluru, KA, India
Quang-Tung Ngo, Trois-Rivières, QC, Canada
Martin Nguyen, Boulder, CO, USA
Tan Nguyen, Vancouver, BC, Canada
LAmeer Nizami, Brossard, QC, Canada
Baydaa Obeid, Grenoble, Auvergne-RhôneAlpes, France
Lorenz Olbrich, Oxford, England, UK
Amaia Ortega-Santos, Linköping, Östergötland, Sweden
Sommer Osman, Ames, IA, USA
Natalie Page, Maple Shade, NJ, USA Jong-Hyeok Park, Cheonan, Chungcheongnam-do, Republic of Korea
Junghum Park, Seoul, Gyeonggi-do, Republic of Korea
Seong Jun Park, Daejeon, Hoseo, Republic of Korea Harsharaj Parmar, Toronto, ON, Canada
Fausto Pasmay, Pittsburgh, PA, USA Yashkumar Patel, Ahmedabad, Gujarat, India
Brittany Pelletier-Villeneuve, Philipsburg, QC, Canada
Yu-Ren Peng, Hsinchu, Hsinchu County, Taiwan
MEmmanuel Peprah-Yamoah, Johnson City, TN, USA
Rhyz Pereira, Philadelphia, PA, USA Kornkanok Phakhunthod, Muang, Nakhon Ratchasima, Thailand
Jackson Pope, Boulder, CO, USA Eli Powell, West Henrietta, NY, USA Sashikanta Prusty, Bhubaneswar, OR, India
Fei Qin, Lafayette, IN, USA Candell Grace Quino, Ikoma, Nara, Japan
RRupesh Rajendran, Atlanta, GA, USA Katrina Ramirez-Meyers, Pittsburgh, PA, USA
Supriya Rana, Hoshiarpur, PB, India Tidapa Rattanaumpa, Mueang, Nakhon Ratchasima, Thailand
Svanik Ravi, Meridian, MS, USA
Hassan Raza, Hung Hom, Kowloon, Hong Kong
Nallayagari Reddy, Rome, Lazio, Italy Saketh Reddy, Chennai, TN, India
Md Masudur Rhaman, Detroit, MI, USA
Bill Riehl, Newark, OH, USA
Ulrich Rist, Eggenstein-Leopoldshafen, BW, Germany
Chushan Ru, Amherst, NY, USA
SRajkumar S., Trichy, TN, India
Mageswari S. S., Coimbatore, TN, India
Mohammad Sabeti, London, ON, Canada
Nicole Saguì, Eugene, OR, USA
Sadman Sakib, Hamilton, ON, Canada
Samaneh Salek, Saint-Philippe, QC, Canada
Varsha Sasikumar S. P., Limerick, Munster, Ireland
Pantipa Sawatmuenwai, Muang, Nakhon Ratchasima, Thailand
Vanessa Scheck, Ulm, BW, Germany
Tess Seip, Toronto, ON, Canada
Brett Setera, Bowie, MD, USA
Shailendra Sharma, Christchurch, Canterbury, New Zealand
Pragati Shaw, Edmonton, AB, Canada
Sachin Shendokar, Greensboro, NC, USA
Angela Shipman, Raleigh, NC, USA
Siddhant Singh, Ann Arbor, MI, USA
Zujhar Singh, Montréal, QC, Canada
Ines Šoljić, Zagreb, Zagreb, Croatia
Matthew Spry, London, England, UK
Soorya Srinivasan, Mayiladuthurai, TN, India
Rhys Standing, Cwmbran, Wales, UK
Nicholas Stavinski, Amherst, NY, USA
James Sturman, Ottawa, ON, Canada
Pragadeesh Subramaniam Venkatesh, Auburn Hills, MI, USA
Faria Sultana, Detroit, MI, USA
Phurin Surachotimongkol, Muang, Nakhon Ratchasima, Thailand
Yoshimasa Suzuki, Kobe, Hyogo, Japan
TMeaghan Tabobondung, Peterborough, ON, Canada
Sadiya Tahsin, Newport News, VA, USA
Akihisa Tanaka, Bunkyo-ku, Tokyo, Japan
Mert Taş, Talas, Kayseri, Turkey
Kaeden Teindl, Chilliwack, BC, Canada
Tushar Telmasre, Austin, TX, USA
Raghav Sai Thiagarajan, Austin, TX, USA
Charlotte Thomas, Boulder, CO, USA
Kira Thurman, Eugene, OR, USA
Sung-Lin Tsai, Yokohama, Kanagawa, Japan
Zarina Turtayeva, Vandoeuvre-lès-Nancy, Lorraine, France
VRinish Reddy Vaidyula, Austin, TX, USA
Francesc Valls Mascaró, Pollenca, Balearic Islands, Spain
Maxime van der Heijden, Eindhoven, North Brabant, Netherlands
Surishi Vashishth, Bengaluru, KA, India
Rebecca Vincent, Santa Barbara, CA, USA
Prerna Vinchhi, Ahmedabad, Gujarat, India
WZakar White, Pittsburgh, PA, USA
Jinnapat Wijitsak, Mueang, Nakhon Ratchasima, Thailand
(continued from previous page)
Rhys Williams, Durham, County Durham, UK
Lea Winter, New Haven, CT, USA
Erin Witherspoon, Rochester, MI, USA
Sara Worku, Detroit, MI, USA
Zhou Yang, Montréal, QC, Canada
Ryan Yao, San Jose, CA, USA
Taber Yim, Philadelphia, PA, USA
Bixian Ying, Münster, NW, Germany
Qihang Yu, Montréal, QC, Canada
Chentian Yuan, Victoria, BC, Canada
YPeter Zacher, Eau Claire, WI, USA
Hao (Nick) Zhang, Baltimore, MD, USA
Yirui Zhang, Cambridge, MA, USA
Jason Zhao, Auburn, AL, USA
Yaoli Zhao, Buffalo, NY, USA
Geyang Zhou, Vancouver, BC, Canada
Larissa Zhou, Mt Pleasant, UT, USA
Penghui Zhu, Eggenstein-Leopoldshafen, BW, Germany
Matej Zlatar, Erlangen, BY, Germany
New Members by Country Look who joined ECS in the Second Quarter of 2022.
Algeria Australia Austria Belgium Canada China Croa�a Denmark Estonia France Germany Hong Kong Iceland India Iraq Ireland Italy
Japan Lithuania Mexico Netherlands New Zealand Russia Singapore South Korea Spain Sweden Taiwan Thailand Turkey UK Ukraine USA Vietnam
Algeria 1 Australia 1 Austria 1 Belgium 2 Canada 66 China 5 Croatia 2 Denmark 1 Estonia 3 France 2 Germany 24 Hong Kong 1 Iceland 1 India 22 Iraq 1 Ireland 3 Italy 6
Japan 22 Lithuania 1 Mexico 1 Netherlands 3 New Zealand 5 Russia 2 Singapore 1 South Korea 24 Spain 2 Sweden 2 Taiwan 3 Thailand 8 Turkey 1 UK 18 Ukraine 13 USA 147 Vietnam 1
ECS congratulates the 2022 Outstanding Student Chapter winner, the ECS Purdue University Student Chapter, for its dedication and commitment to the advancement of electrochemical and solid state science and engineering education. The Society established the Outstanding Student Chapter Award in 2012 to recognize distinguished ECS Student Chapters that demonstrate active participation in the Society’s technical activities; establish community and outreach activities in the areas of electrochemical and solid state science and engineering education; and create and maintain a robust membership base.
In the past year, Purdue University’s student chapter webinar series promoted discussion and collaboration between electrochemistry and solid state science students at Purdue and in the Greater Midwest. A recent topic was “Modeling, Characterization & Analytics of Electrochemical Energy Systems.” The chapter maintains a website and active Twitter and LinkedIn profiles where it lists activities and members to engage with the larger electrochemistry community.
Think your student chapter’s achievements should be considered for next year’s award? Applications for the 2023 ECS Outstanding Student Chapter Award are due on April 15, 2023. STUDENT
ECS also congratulates the 2022 Chapter of Excellence winner, the ECS University of Waterloo Student Chapter, for its hard work and dedication. This year the chapter maintained an active membership, hosted a virtual workshop series on electrochemistry techniques, and engaged with the larger electrochemistry community via its website and social media profiles on Twitter, Instagram, and LinkedIn.
A special thank you to the outstanding Student Chapter Award Subcommittee for reviewing applications and choosing award recipients from among the many excellent ECS Student Chapters:
Neal Golovin, Individual Membership Committee Chair, Booz Allen Hamilton
Alice Suroviec, Education Committee Chair, Berry College John Staser, Ohio University
Uroš Cvelbar, Jožef Stefan Institute Mohammedreza Nazemi, Yale University
On June 2, 2022, the ECS Board of Directors approved the chartering of four new student chapters, bringing the total number of chapters around the world to 117. We welcome these new student chapters into our supportive, global community.
Suranaree University of Technology, Thailand University of Illinois at Urbana-Champaign, US
For more information about student chapters and the benefits they bring to their members, visit the ECS Student Center. To gauge the scope of the Society’s global student chapter network, check the Student Chapter Directory To establish an ECS student chapter at your academic institution, review the student chapter guidelines and submit an application.
Lambton College, Canada College of Engineering, India ECS Purdue Student Chapter Officers (left to right): Anu Alujjage (Communications Director), Julia Meyer (Vice President), Susmita Sarkar (Executive Advisor), Debanjali Chatterjee (President), Sourim Banerjee (Secretary), and Aditya Singla (Treasurer). ECS Waterloo Student Chapter Officers (left to right): Elizabeth Esther Miller (Treasurer), Mariam Gad (Internal VP), Gillian Hawes (President), Mohammadali Emadi (Outreach Coordinator), Vipin Kumar Singh (Media Chair), and Moin Ahmed (External VP).The ECS Detroit Student Chapter continued to grow during the past semester, welcoming members from Oakland State University and the University of Detroit Mercy, including Prof. Ankun Yang (Oakland) and Prof. Marwa Abdel Latif (Det. Mercy). Prof. Long Luo from Wayne State University continued as faculty advisor. On March 25, the chapter reported 17 members and elected Chair Sachini Rodrigo, Vice Chair Pragadeesh Subramaniam Venkatesh, Secretary Josephine Dykstra, and Treasurer Daohua Liu to lead the group into the future.
The chapter hosted several online talks in the spring as part of its monthly Coffee Fueled Electrochemistry seminar series. Noteworthy speakers included Prof. Paul Bohn, a top researcher in molecular nanotechnology from the University of Notre Dame, who participated
in a networking lunch and seminar in April; and Dr. Cameron Tanner of Corning Inc., who spoke at the May seminar on the development of thin ceramics at Corning and their application to high energy density batteries. On July 28, the chapter hosted Prof. Lei Chen from the University of Michigan, who presented a talk on accelerating computational design of dendrite-free lithium-ion batteries.
The Lambton College Student Chapter joined ECS on June 2. Members conduct research in the Centre for Electrochemical, Energy & Nanotechnology Research (CE2NTRE) at Lambton College, where research interests cover many industry-driven areas such as energy conversion and storage technologies; materials selection, characterization, and optimization for industrially important applications; water and wastewater desalination; corrosion protection and monitoring; and electrosynthesis and nano-catalysis for energy applications.
Chapter members will present several times throughout 2022 and 2023 on current research in the CE2NTRE Lab. The bi-monthly presentations will be hosted in person at Lambton College and virtually as part of the Lambton College Research & Innovation Seminar Series. The purpose of the Seminar Series is to share knowledge, enhance collaboration, and create connections by
On June 18, the chapter hosted its first in-person gathering of the year—a barbecue on Detroit’s Belle Isle—and said goodbye to wonderful former chair Elif Tezel, who is pursuing other opportunities near family, and Dr. Ruchiranga Ranaweera, the chapter’s founding chair, who recently graduated and is starting work at Intel, Inc. The chapter thanks ECS for its continued support. showcasing research projects to industry partners, local businesses, and members of the college’s research and innovation department or any other interested party.
In mid-August, Mohsen Sheikhzadeh, a PhD student working in the CE2NTRE Lab, presented on the performance and optimization of a desalination process using a direct contact membrane distillation technique. Upcoming presentation topics include: the development and optimization of pulsed electrodeposition for energy storage applications; experimental and computational studies on electrical conductivity of inorganic solutions aiming at optimization of industrial processes; the design, fabrication, and optimization of electrochemical flow-through cells for electrodeposition; and electrochemical studies of electrodes and membranes employed in metal-ion and metal-air batteries as well as supercapacitors and lowtemperature fuel cells.
The ECS Technische Universität (TU) Ilmenau Student Chapter elected new officers via online voting at the end of 2021. Chair Martin Leimbach, Vice Chair Ivan Genov, Secretary Gisella Lucero, and Treasurer Nurul Amanina Binti Omar began their terms in 2022.
The chapter held its first in-person meeting of the year May 19–20, a group visit to Atotech, a worldwide supplier of chemicals and processes for electroplating in various fields (e.g., PCB, plating on plastic, corrosion protection, and decorative and functional coatings). After a short introduction to the company, the chapter enjoyed a plenary talk by Prof. Andreas Bund, head of the Electrochemistry and Electroplating Group at TU Ilmenau. Prof. Bund presented on the fundamentals of state-of-the-art redox flow battery systems and future possibilities to deploy the technology in power grids and in stationary applications. The chapter participated in a guided tour of the Atotech facilities and R&D laboratories, and attended two presentations. Chapter member Martin Leimbach described the challenges and opportunities he experienced while working toward his PhD in electrochemistry and electroplating at TU Ilmenau, where he worked on the characterization of chromium deposition from trivalent electrolytes. Mirko Ante discussed his experience of starting a career in industry with a background in energy technology, thereby improving large-scale hydrogen storage techniques.
On the second day, chapter members presented their current research topics. Lukas Grohmann gave an update on his work on the interdiffusion of gold and nickel, which occurs during the fabrication
of glass feedthroughs for wire contacts in electronic devices and batteries. Ivan Genov briefly introduced his dissertation on “anodefree” lithium metal rechargeable batteries and on how to improve their performance. Gisella Lucero discussed electrochemical detection of nitrite ions with PEDOT films and PdAu/PEDOT composites. Lars Lösing gave a talk on the deposition of tin from electrolytes at neutral pH for applications in electronics.
The ECS TU Ilmenau Student Chapter hopes to continue such activities in the future, and plans to meet in person again this fall.
ECS TU Ilmenau Student Chapter members meet at Atotech in Berlin.In 2022, the chapter hosted several events that focused on special topics in electrochemistry. On March 30, Prof. Brett L. Lucht (University of Rhode Island) visited the university’s Chair of Technical Electrochemistry and gave a colloquium on “Solid Electrolyte Interphase (SEI) of Lithium-Ion batteries: Role of additives and crossover reactions on stability and performance.” Lucht provided interesting insights into the anode SEI formation in the presence of electrolyte additives such as vinylene carbonate (VC) and fluoroethylene carbonate (FEC). The colloquium was held as a hybrid event, making it possible to reach a broad audience.
On July 7, the chapter hosted an in-person panel discussion titled “How Do We Impact the Transition into a Renewable Energy Economy?” with invited speakers Dr. Andreas Peschel (Linde Engineering), Dip-Ing. Peter Rostek (Airbus SE), Prof. Thomas Hamacher (TUM), Eugenio Scionti (E.ON) and Dr. Kai Zosseder (Scientists 4 Future & TUM). The main goal was to gain insights into the current state of the transition into a renewable energy economy. How is this transition happening? What are the limiting factors and where can we contribute most efficiently to this challenge? Panelists from industry and academia were invited to combine different points of view and build a broader picture of the issues. More than 60 guests, including students, PhD students, and industry representatives attended. With this event, the chapter intended to inspire young students in their early careers and widen their perspectives on the challenges of the future. An informal gathering with food and drinks followed the panel, providing guests with time for further questions and exchanges.
In the coming months, the chapter plans to host seminars on frequently used laboratory methods to gain a deeper understanding of them from experts in the field, including thermogravimetric analysis with Dr. Mohammad Reza Saraji-Bozorgzad (Photonion GmbH) and scanning electron microscopy as part of the Materials & Methods Club.
The ECS Oklahoma State Student Chapter engaged with and supported many local community events. Chapter members volunteered as presentation judges for Oklahoma State University (OSU)’s First Annual Undergraduate Research Symposium and worked tables at events for recruitment purposes. Members demonstrated science experiments at OSU’s Grandparents University, a three-day event for grandparents and grandchildren to inspire K-12 students to engage with many different fields to find their passion.
In fall 2022, student chapter members attended the Women in Science event at the Science Museum of Oklahoma. This event, funded by Oklahoma EPSCoR (Established Program to Stimulate Competitive Research), is designed to introduce young girls to STEM fields and inspire them to pursue STEM careers. The chapter is excited to participate in similar upcoming community outreach projects, as one of the group’s main priorities is to inspire the next generation in the field of electrochemistry.
ECS Oklahoma State Student Chapter members (front row from left to right) Dr. Barry K. Lavine (Faculty Advisor), Zia Syed, James T. Moulton, Akansha Sharma, Haoran Zhong, (standing from left to right) David Bruce, George Affadu Danful , Theophilus Neequaye , Elizabeth Donkor, and Viswanath Nukala Attendees at the ECS Technische Universität München (TUM) Student Chapter’s panel discussion, “How Do We Impact the Transition into a Renewable Energy Economy?” (bottom: from left to right): Dr. Kai Zosseder (Scientists 4 Future & TUM), Dip-Ing. Peter Rostek (Airbus SE), Eugenio Scionti (E.ON), Prof. Thomas Hamacher (TUM), and Dr. Andreas Peschel (Linde Engineering).In collaboration with the Department of Chemical Engineering at Texas Tech University (TTU), the ECS Texas Tech University Student Chapter hosted its first post-lockdown in-person seminar on April 1. In his presentation, “Beyond ‘Dream’ Materials,” Dr. William E. Mustain encouraged the audience to think about materials being engineered to better the performance of fuel cells and energy storage devices. Dr. Mustain discussed scenarios in which better performance and operational stabilities were achieved without new materials, and suggested that thinking differently about bettering cell performance is necessary in order to move forward. Moreover, Dr. Mustain pointed out the disconnect between reality and the theoretical capacities of Al batteries, which cannot reach those theoretical capacities and cannot compete with existing technology unless drastic operational changes are implemented.
Before the seminar, the chapter hosted a reception for Dr. Mustain where students and faculty discussed Dr. Mustain’s experiences in graduate school, as a professional, and as an ECS member serving on multiple Society committees. The chapter was honored to host Dr. Mustain and learn from his valuable experiences.
From July 14 to 15, in an effort to speed electrochemistry into the future, the chapter hosted a two-day outreach event called “Racing into the Future.” The chapter partnered with the Texas Tech Alumni Association (as part of their Legacy University initiative) to provide
kids aged 7 to 13 with a taste of electrochemistry and its impact. Chapter advisor and TTU Department of Chemical Engineering Chair Dr. Gerardine G. Botte conducted the program. Participants learned about fuel cells, their applications, and challenges with their implementation. The course consisted of five different modules, each of which had a dedicated mentor (an ECS student chapter member) training each of the six teams that participated. The kids enjoyed hands-on training with fuel cells and learned about their pivotal role in the functioning of fuel cell cars. Participants (and their grandparents) showed off what they had learned in three Jeopardy-style quizzes throughout the event. On Day 2, the kids operated fuel cell cars and performed calibration to understand the amount of fuel necessary for their cars to run a specified distance. The six teams then participated in a fuel cell race, with participants, grandparents, and mentors enthusiastically cheering on their cars. Three prizes were awarded: race winner, quiz winner, and mentor’s choice award. Overall, this was a productive and satisfying outreach event for everyone involved. The grandparents spent quality time with the kids; the kids learned electrochemical technologies from their mentors; and mentors taught electrochemistry to the next generation. Through “Racing into the Future,” electrochemistry brought together people of all ages for a lively, fun, and educational session.
The ECS University of Waterloo Student Chapter planned and held its first event with its new executive team members on June 9, a virtual Lithium-Ion Battery Industry Panel Discussion. The chapter hosted panelists from three Li-ion battery space industry leaders (Echion Technologies, Li-Cycle, and Zen eBikes) to get their perspectives on working as electrochemists and engineers in industry, their personal career paths into the roles they currently hold, and their experiences as leaders in the Li-ion battery industry. The panelists were Dr. Loubna El Ouatani, Chief Product Officer of Echion Technologies, which specializes in fast-charging Li-ion anodes; Chris Biederman, PEng, CTO of Li-Cycle, which specializes in Liion battery recycling; and Dr. Ravi Kempaiah, co-founder of Zen Electric Bikes, which manufactures premium electric bicycles. The well-attended event with 96 registrants featured a lively interactive discussion. A full recording is available on the chapter’s website
The 243rd ECS Meeting and 18th International Symposium on Solid Oxide Fuel Cells (SOFC-XVIII) will take place in Boston, MA from May 28 to June 2, 2023, at the Hynes Convention Center and Sheraton Boston. This international conference brings together scientists, engineers, and researchers from academia, industry, and government laboratories to share results and discuss issues on related topics through a variety of formats such as oral presentations, poster sessions, panel discussions, tutorial sessions, short courses, professional development workshops, a career fair, and exhibits. The unique blend of electrochemical and solid state science and technology at an ECS meeting provides an opportunity and forum to learn and exchange information on the latest scientific and technical developments in a variety of interdisciplinary areas.
To give an oral or poster presentation at the 243rd ECS Meeting and/or SOFCXVIII, you must submit an original meeting abstract for consideration via the ECS/SOFC website, no later than December 2, 2022. Faxed, e-mailed, and/or late abstracts are not accepted. Meeting abstracts should explicitly state objectives, new results, and conclusions or significance of the work. After the submission deadline, symposium organizers evaluate all abstracts for content and relevance to the symposium topic, and schedule accepted submissions as either oral or poster presentations.
Letters of Acceptance/Invitation are sent via email in February 2023, notifying corresponding authors of accepted abstracts, and the date, time, and location of their presentations.
How and when a poster or oral presentation is scheduled is at the symposium organizers’ discretion, regardless of presenters’ requests.
Oral presentations must be in English. LCD projectors and laptops are provided for all oral presentations. Presenting authors MUST bring their presentations on USB flash drives to use with dedicated laptops located in each technical session room. Speakers requiring additional equipment must make written request to meetings@electrochem.org at least one month prior to the meeting so appropriate arrangements can be made, subject to availability, and at the author’s expense.
Poster presentations must be displayed in English, on a board approximately 3 feet 10 inches high by 3 feet 10 inches wide (1.17 meters high by 1.17 meters wide), corresponding to their abstract number and day of presentation in the final program.
ECS Meeting Abstracts—All meeting abstracts are published in the ECS Digital Library, copyrighted by ECS, and become ECS’s property upon presentation.
ECS Journals—Authors presenting papers at ECS meetings are encouraged to submit to the Society’s technical journals: Journal of The Electrochemical Society, ECS Journal of Solid State Science and Technology, ECS Advances, or ECS Sensors Plus. Although there is no hard deadline for submitting these papers, six months from the symposium date is considered sufficient time to revise a paper to meet stricter journal criteria. Author instructions are on the ECS website.
ECS Transactions—Select symposia publish their proceedings in ECS Transactions (ECST). Please check the individual symposia Calls for Papers in this document. Authors presenting in these symposia are welcome to submit a full-text manuscript based on their presentation to ECST. Issues of ECST are available for sale on a pre-order basis, as well as through the ECS Digital Library and the ECS Online Store. Review each individual symposium’s listing in this Call for Papers to determine if your symposium is publishing an ECST issue. Visit the ECST website for additional information, including overall guidelines, author and editor instructions, a downloadable manuscript template, and more.
ECSarXiv All authors are encouraged to submit their full-text manuscripts, posters, slides, or datasets to ECS’s preprint service, ECSarXiv. For more information, visit the ECSarXiv website. Note that submission to ECSarXiv does not preclude submission to ECST.
ECS Short Courses provide students and seasoned professionals with in-depth education on a wide range of topics in a short, intensive time period. Novices and experts advance their technical expertise and knowledge through personalized instruction by academic and industry
experts. Short Courses require advance registration and may be canceled if course enrollment is less than 10 registrants. Learn more at https:// www.electrochem.org/short-courses
The 243rd ECS Meeting is the right place to exhibit. The Society provides a powerful platform for meeting major new customers while enhancing relationships with current customers from around the world. Traffic in the exhibit hall is generated by supplying coffee and networking breaks along with evening poster sessions. Your presence at ECS’s leading industry event positions your brand as serious and reliable—and it’s a great way to build buzz for new products! Exhibit opportunities can be combined with sponsorship to suit your marketing needs. Contact sponsorship@ electrochem.org for further details.
All participants—including authors and invited speakers—are required to pay the appropriate registration fees. Meeting registration information is posted on the ECS website as it becomes available. The deadline for discounted early registration is April 24, 2023.
The 243rd ECS Meeting and SOFC XVIII take place at the Hynes Convention Center and Sheraton Boston. Please refer to the meeting website for the most up-to-date information on hotel availability and blocks of rooms where meeting participants receive special rates. The hotel block is open until April 24, 2023 or it sells out.
Letters of Invitation are sent in February 2023 via email to the corresponding authors of all accepted abstracts, notifying them of the date, time, and location of their presentations. Anyone requiring an official Letter of Invitation should email abstracts@electrochem.org These letters do not imply any financial responsibility on the part of ECS.
ECS divisions and sections offer travel grants to assist students, postdoctoral researchers, and young professionals in attending ECS biannual meetings. Applications are available beginning December 2, 2022, at www.electrochem.org/travel-grants. The submission deadline is February 27, 2023. For general travel grant questions, contact travelgrant@electrochem.org.
Additional financial assistance is limited and generally governed by symposium organizers. To inquire if additional funding is available, contact the organizers of the symposium in which you are presenting.
ECS biannual meetings and SOFC-XVIII provide a wonderful opportunity to solidify and strengthen your brand through sponsorship. Give your brand more visibility and reinforce your position as an industry leader by sponsoring ECS and SOFC meeting events. Companies can choose from a wide array of activities, from symposia to special events, which deliver worldwide recognition as a supporter of electrochemical and solid state research—and enhance ECS meetings.
ECS and SOFC also offer specific symposium sponsorship. By sponsoring a symposium, your company helps offset travel expenses, registration fees, complimentary proceedings, and/or hosts receptions for invited speakers, researchers, and students. Please contact sponsorship@ electrochem.org for further details.
BioLogic USA/BioLogic SAS (14*)
Duracell (65)
Gamry Instruments (15)
Gelest, Inc. (13)
Hydro-Québec (15)
Pine Research Instrumentation (16)
Energizer (77)
Faraday Technology, Inc. (16)
GE Global Research Center (70)
Lawrence Berkeley National Laboratory (18)
Scribner Associates, Inc. (26)
Toyota Research Institute of North America (14)
BASi (7)
Central Electrochemical Research Institute (29)
Center for Synthetic Organic Electrochemistry, University of Utah (1)
DLR-Institut für Vernetzte Energiesysteme e.V. (14)
EL-CELL GmbH (8)
Electrosynthesis Company, Inc. (26)
Ford Motor Corporation (8)
GS Yuasa International Ltd. (42)
Honda R&D Co., Ltd. (15)
Medtronic Inc. (42)
Nissan Motor Co., Ltd. (15)
Pacific Northwest National Laboratory (PNNL) (3)
Panasonic Corporation (27)
Permascand AB (19)
Plug Power, Inc. (1)
Teledyne Energy Systems, Inc. (23)
Underwriters Laboratories (1)
Center for Solar Energy and Hydrogen Research
Baden-Württemberg (ZSW) (18)
Advanced Cell Engineering (1)
Cummins, Inc (4)
General Motors Holdings LLC (70)
Giner, Inc./GES (36)
Ion Power Inc. (8)
Kanto Chemical Co., Inc. (10)
Los Alamos National Laboratory (14)
Metrohm USA, Inc. (9)
Microsoft Corporation (5)
Occidental Chemical Corporation (80)
Sandia National Laboratories (46)
Sherwin-Williams (1)
Technic, Inc. (26)
United Mineral & Chemical Corporation (1)
Western Digital GK (8)
Westlake (27)
Yeager Center for Electrochemical Sciences (24)
ECS, a prestigious nonprofit professional society, has led the world in electrochemistry, solid state science and technology and allied subjects since 1902, providing a rigorous and high-quality home for the whole community.
ECS is dedicated to moving science forward by empowering researchers globally to leave their mark on science. The Society connects a diverse and representative constituency of members and nonmembers to accelerate scientific discovery, facilitate the engagement of an inclusive network, and champion the dissemination of research to support a sustainable future.
For more information on becoming a member, or publishing in ECS publications, visit electrochem.org