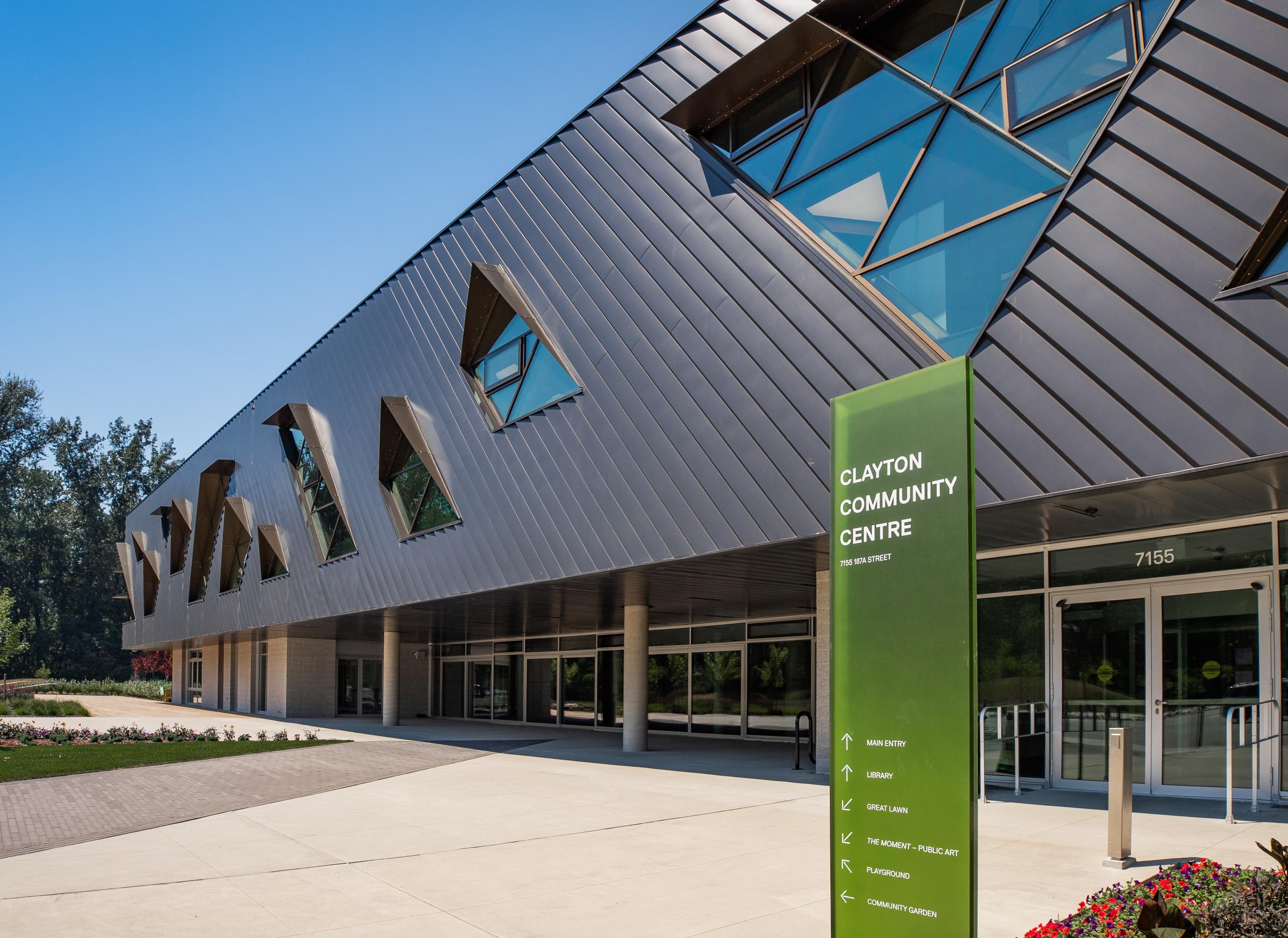
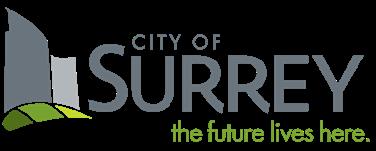
This study has been made possible through the Impact Fund. The Impact Fund is an internal program aimed at supporting thought leadership initiatives around sustainability and digital innovations for the built environment. It runs on an annual basis and funds projects, studies, essays and advisory pieces that provide new insights on how we perceive, design and build our human environment. It is an opportunity to reflect, question, think big and offer solutions to make our world more resilient, just, and regenerative.
Thomas Bamber would like to thank the following people for their contributions to this paper:
Stuart Hood
Sonja Lotimer
Louise Hamot
Aiman Arar
The City of Surrey Facilities team
Melissa Higgs
The HCMA team
Tracy Wong
Eben Simmons
Kevin Hydes
As engineers, we continually talk about how to maximize results for each new project. One of the best methods is to revisit completed buildings and measure their lived performance against the fine detail of our original designs — in other words, to close the performance gap. Visiting completed projects to carry out a post-completion energy evaluation is a vital and yet frequently omitted opportunity.
Following its first full year of operation since COVID-19, Introba revisited Clayton Community Centre, Canada’s onetime largest Passive House building, to check whether its performance lived up to its design credentials. We were delighted that the study’s results were overwhelmingly positive, showing Passive House really does deliver on its promises of minimal energy for large, commercial buildings – a valuable insight for future projects.
One of the most significant failings of the construction industry is the lack of transparency in sharing knowledge about what has and hasn’t worked in practice.
Imagine how much faster building design could evolve and improve if everyone published the lessons learned.
It could provide invaluable hard data about what’s missing in the gap between design and reality. So why isn’t such collaboration common practice? Sadly, even if our clients permit us to share data, the major stumbling block is competitiveness between different companies, which inhibits us from sharing performance outcomes that would benefit everyone. On top of this, most companies lack the time and funding to revisit past projects. I know this is because I, too,
am guilty of failing to share useful knowledge from unexpected building outcomes. While I’ve spent the past few years extolling the virtues and innovations of Clayton Community Centre at conferences and webinars, for example, I’ve only recently found time to revisit the occupied building for a post-completion energy evaluation. I’d have liked to return sooner to examine and interrogate the practical working of the systems we designed – and, ideally, to share any findings with colleagues and competitors to benefit our future collective clients.
This time, I’m sharing what we learned from the project with two clear questions: is the actual energy consumption in line with our design calculations? and was Passive House a good fit for a large, non-domestic building? Let’s find out.
Location: Clayton, Greater Vancouver, British Columbia
Budget : $46m CAD
Floor Area: 7,000 m2
(Used for Energy Use Intensity (EUI) data)
Treated Floor Surface Area (TFSA): 5,764 m2
(Used in Passive House calculations)
Client : City of Surrey
Architect : HCMA
Structural: Read Jones Christofferson
Mechanical: Integral Group (now Introba)
Electrical: AES
• Includes a 1,200 m2 Library, Gymnasium, Fitness Centre, Multipurpose Rooms
• Substantial completion in 2020. Official opening in October 2021.
• Passive House Certified, PHPP output as below:
• Heating Energy Demand Intensity (TEDI) = 8.4 kWh/m2.yr
• Cooling Energy Demand Intensity (CEDI) = 0.4 kWh/m2.yr
• Primary Energy Renewable (PER) = 87.3 kWh/m2.yr
• Fully electrified mechanical systems:
• 2 x 140 kW Air Source Heat Pumps provide building heating and cooling
• Radiant panel and hydronic fan coil unit heating and cooling
• Natural ventilation for shoulder season cooling and ventilation
• Electric immersion heaters for domestic hot water heating
thermal energy demand
building,
1. T he building is comparatively well-metered thanks to a combination of building controls system trend data, electrical submeters and utility electricity bills. The mechanical systems do not include thermal meters, so the heating and cooling thermal demand profile in Figure 1 was calculated using a combination of Air Source Heat Pump (ASHP) trend data, ASHP literature and weather data. A more detailed breakdown of how the ‘2022 Actual numbers’ were completed is provided in Appendix A.
2. PHPP is the energy calculation tool used for Passive House buildings, and is also a key part of the compliance process. For an applesto-apples comparison, the PHPP design spreadsheet from 2017 was adjusted to correspond with how the building is currently being used. A more detailed breakdown of the PHPP spreadsheet adjustment is provided in Appendix B. Adjustments include:
a. Use of 2022 recorded weather for the site (nearest weather station is Abbotsford)
b. Removal of Café that isn’t in operation
c. Heating and cooling setpoints reflect occupant usage
d. Lighting hours match hours of operation
Figure 1 presents the annual of the which had to be calculated from building metering and controls data. This was one of several steps required to inform the energy breakdown for the building presented in Figure 2.Probably the most celebrated aspect of Passive House as a philosophy is the central tenet of minimizing heat demand by going the extra mile regarding insulation, air-tightness, thermal bridging, form factor, and the harvesting of internal heat gains to offset mechanical heating. The compliance heat demand target of 15 kWh/m2.year is astonishingly low compared to typical new buildings, and the Clayton Community Centre PHPP calculations clock in at 8.4 kWh/m2.year.
Per Figure 2, the Actual heating consumption of the building in 2022 was marginally higher than the adjusted PHPP, indicating that envelope and heat retention principles have paid the intended dividends. The average monthly cost of heating the 7000 m² building in 2022 was $433.
On a $/m² basis, this is about seven times lower than the British Columbian average across all building types, based on a literature review.
A closer review of the systems on-site showed that minimal injection of heat is needed from the two Air Source Heat Pumps (ASHP) during winter. On a typical winter’s day, which means 4°C and cloudy in greater Vancouver, only one of the eight available heat pump compressors is required to keep the building warm. If the sun is shining, the building is effectively self-heating in winter. There were several days during peak winter in February of 2022 when both the ASHP experienced a fault and were locked out. The alarm signal wasn’t even spotted because it took three days for any noticeable drop in room temperature.
What is also promising is that the post-completion energy evaluation review highlighted inefficiencies in the system that can be ironed out, offering the opportunity for lower heating bills in the future, see Lessons Learned sections.
Figure 2 compares the energy consumption breakdown for the Passive House Planning Package (PHPP) design calculations versus the recorded consumption for 2022. Figure 2: 2022 Actual Energy Usage vs PHPP CalculationsThe data shows that, during its first year of operation, Clayton Community Centre used 22% more energy than was predicted from the Passive House design and compliance tool PHPP.
Consuming 22% more energy than the design calculations is much better than it sounds— 22% of a very low Passive House target is still low.
A 2020 study of Housing projects in British Columbia demonstrated that most projects exceed their energy calculations by 25% to 280% and that ambitious sustainability targets typically increase the gap between design and actual energy performance. As discussed below, Clayton’s postcompletion energy evaluation also identified several operational inefficiencies and hence room for improvement in 2023 and beyond.
The figures are also distorted by the surprise worst offender: the LED lighting.
If we remove lighting from the equation, the building only uses 6% more energy than its calculated target. In one sense, the poor performance of the lighting flatters the building. If the worst offender in terms of energy is lighting, then the traditionally big-ticket items such as heating, cooling, and equipment must be performing exceptionally well. As to why the lighting is so much higher, per Figure 2, the design team confirmed that it isn’t the installed power density, which leaves either an unknown circuit, such as Performing Arts lighting use, or perhaps lighting left on overnight (a straightforward fix).
It’s also fair to question whether the building’s deep plan is partially responsible for the high lighting load. In chasing the ideal form factor, i.e.,
minimizing the exterior surface area to reduce heat loss, the project became more challenging in harvesting daylight. Despite prominent skylights, the electric lighting is typically on, even on clear days. Further analysis is scheduled to explore whether dimming can achieve energy savings.
Six of the seven remaining energy end uses in Figure 2 highly correlate with the PHPP design calculations. Given the impressive ambition of Passive House’s energy targets, it is undoubtedly a great success for the project to be so close to its targets, with room for further improvement.
Figure 3 shows how the development compares with other community centers in BC. Based on greenhouse gas (GHG) emissions, the project is an exemplar thanks in part to being fully electrified. The lower graph shows how Clayton’s overall energy use intensity (EUI) is comparable to other highperformance facilities based on the first year of operation.
The real success story for the project, however, is its popularity.
Visit any morning or evening, and it’s clear that a whole host of community groups and demographics occupy the various spaces inside and out. Since the building is operating close to capacity, it makes the comparison between PHPP and Actual consumption more compelling; and it is impressive and reassuring to see that PHPP bore such a close correlation for metrics such as equipment and hot water.
The Gathering Place CoV
Champlain Heights Community Centre CoV
Coal Harbour Community Centre CoV
Hastings Community Centre CoV
Dunbar Community Centre CoV
Douglas Park Community Centre CoV
Roundhouse Community Centre CoV
Kitsilano Community Centre & Arena CoV
Creekside Com Incl Daycare (New) CoV
Sunset Community Centre (New) CoV
Barclay Manor CoV
Mt Pleasant Com Centre (New) CoV
Fraser Heights Rec Centre CoS
Guildford Rec Centre & Library CoS
Bridgeview community centre CoS
Newton Athletic CoS
Don Christian Rec Centre CoS
Clayton Community Heights CoS
City
The Gathering Place CoV
Coal Harbour Community Centre CoV
Creekside Com Incl Daycare (New) CoV
Champlain Heights Community Centre CoV
Kitsilano Community Centre & Arena CoV
Hastings Community Centre CoV
Dunbar Community Centre CoV
Douglas Park Community Centre CoV
Sunset Community Centre (New) CoV
Barclay Manor CoV
Roundhouse Community Centre CoV
Mt Pleasant Com Centre (New) CoV
Fraser Heights Rec Centre CoS
Newton Athletic CoS
Guildford Rec Centre & Library CoS
Bridgeview community centre CoS
Don Christian Rec Centre CoS
Clayton Community Heights CoS
City of Vancouver & Surrey - Community Centres Energy Intensity
ekWh/m2.year
One of the key takeaways from the post-completion energy evaluation was that the mechanical controls were not operating to their full potential.
One example was hot and cold air being supplied to adjacent parts of the same space. These unnecessary calls for simultaneous heating and cooling show up in the pumping energy demand of Figure 2, as hydronic energy is wastefully circulated around the building. There were instances where free cooling or natural ventilation could have resolved thermal demand without any input from the hydronic systems.
The root cause of these inefficiencies is not uncommon; the controls system’s sequences of operation (code-based instructions dictating how mechanical plant and valve systems respond to room sensors and other inputs) were both bespoke and relatively sophisticated due to the nature of a large, innovative, commercial Passive House development.
These systems can become tripwires for the operators who inherit them and are required to resolve urgent issues on the fly during the first few seasons of the building’s operation. Having neither designed them nor written the script, it’s all too
easy to make changes that impact performance elsewhere, often during an entirely different season. The result is that many buildings have systems that are not operating in the optimal, most efficient way they were designed. The solution is to ensure that post-completion energy evaluation frameworks are implemented.
An excellent example is CIBSE Soft Landings which uses a client-focussed approach to smooth the transition into occupancy. It is extraordinary to think this isn’t the norm; for a tiny fraction of the cost of the building, you have the designers spend time in the building working with the operator to ensure the various systems operate as intended.
There is real payback here, both in terms of utility costs and ensuring that occupants enjoy the healthiest, most comfortable version of the building. A recent Soft Landings project in Canada by Introba achieved such significant annual energy savings that the study carried a two-year payback.
Another key takeaway from the post-completion energy evaluation was that the natural ventilation (NV) system was not operating to its full potential. This is an area where the building could still achieve a huge improvement in energy, comfort, and health, and which carries lessons for other future buildings.
Clayton Community Centre features a mixed-mode system whereby NV operates interchangeably with mechanical systems. Mixedmode is gaining traction across many markets as it offers a powerful means to make buildings and occupants more resilient. Mixed-mode buildings are nimble and inherently more able to mitigate the uncertainties of climate change, pandemics, wildfires, and blackouts. While natural ventilation is active, it improves health by boosting ventilation rates and minimizing carbon dioxide and airborne pathogens.
Based on the PHPP calculations (verified with independent energy modeling), NV achieves a 45% saving in annual cooling energy and is key in meeting the PHPP Cooling Energy Demand Intensity (CEDI). The post-completion energy evaluationfound that the system operates nowhere near its potential for several reasons.
A. The first issue concerns spatial planning and late design changes that go unnoticed. The staff who occupy the desk at the feature Library space every day are standing in direct sunlight beneath the windows. The staff desk was originally internal but was switched to the façade during construction. This fundamentally impacts HVAC operation, as permanent occupants beneath the window are significantly more likely to overheat than others just a few meters away. The net result was that the staff were understandably turning down the thermostat to 21°C to stay comfortable when the sun was shining. In a mixed-mode building, this bodes badly for natural ventilation as the mechanical systems will automatically come on during relatively mild days.
The irony is that natural ventilation can achieve fantastic comfort levels at much higher temperatures than air-conditioning, often into the high 20s and early 30s degrees Celsius. Generous airflow and shading from direct sun are key to achieving this goal. The lesson is to ensure that occupants, especially permanent ones, are not in direct sunlight. Internal blinds could help in this instance.
B. The second issue, and one of the most common flaws that blight natural ventilation, are the openings themselves need to achieve the target
accessible areas. At Clayton, the Multipurpose Room fell into this trap due to unforeseen details. The thickness of the framing plus the thickness of the Passive House triple glazing meant we achieved a sliver of the target. What’s more, the security requirements of the space meant that when this issue came up on-site, we could not revert to a side-hung operable. The result is a compromised NV system: it can give you a psychological connection to the outdoors but can’t provide an alternate means of cooling and ventilating the space.
This incongruence between the design-free area and that realized by the installation is especially likely to come up in markets such as North America, where natural ventilation is still relatively uncommon. It could be the sill, actuator, or security limitation if it isn’t the frame. The lesson learned here is to mandate holistic framing package shop drawings, including the operables at full extents, the mounted actuators at design extents, electrical and controls wiring, structural framing, and adjacent elements such as sills.
C. Finally, it seems there are some ongoing discussions related to controls. The permanent occupants weren’t fully clear about how to operate and override the natural ventilation via the thermostats, which aren’t intuitive despite having ‘traffic-light’ back-lighting intended to inform when conditions are appropriate for natural ventilation. One solution could be adding permanent signage next to the relevant stats to clarify the operation and intent.
Alternatively, more effort could be spent making the stats more intuitive and easier to use. This is an area where further post-completion energy evaluation and client-focused discussion could significantly improve performance.
The good news is that the post-completion energy evaluation of Clayton Community Centre confirmed that the highly ambitious Passive House energy targets are a reality, not just a number in a spreadsheet.
Based on this project, Passive House principles seem to be a fantastic fit for large, commercial, and public buildings in this climate. The benefits are most evident when we consider the incredibly low thermal energy demand of the building. While there are costs associated with the details and specifications required for Passive House, the benefits are unequivocal.
The project also provides a powerful exemplar of resilient architecture. Resilience is something of a buzzword in 2023 in politics and building design alike. As a community cent that threads together the surrounding urban populations, Clayton Community Centre enhances social connectedness. The building itself is highly nimble and adaptable, able to serve as a critical refuge during any catastrophe or unforeseen event. It has demonstrated that it can provide comfort during BC’s extremes of heat and cold with a minimal energy input. If a pandemic or blackout occurs, the building has daylight and natural ventilation to help it stay occupiable and contribute to human health with elevated ventilation levels. Similarly, the systems can revert to mechanical mode with
air highly filtered if there are wildfires.
Such benefits have brought trade-offs in design, however. While the compact form and deep plan made it financially feasible to maximize airtightness and insulation, this also meant daylight didn’t penetrate as far as we’d have liked, and it increased the number of hours electric lighting is required in some of the larger spaces. The postoccupancy analysis addressed this by showing the clear steps occupants can take to further reduce consumption, including bringing down the lighting load, resolving teething issues with the building’s system controls, and taking full advantage of the available natural ventilation system.
In conclusion, as a building designer, there’s no greater satisfaction than seeing your projects occupied and used as you intended. It’s a continual learning process, and impossible to get every detail right… but we keep trying. I hope that by sharing both the successes and the lessons learned from this project, we’ve also contributed in some small way to the wider pool of knowledge
This section provides a deeper dive into the process of extracting and compiling the 2022 Actual consumption from building controls’ historic data, submeters and Hydro bills.
Equipment
11%
Lighting 44%
Heat Pumps, DHW, Pumps, HRV 33%
Fitness Centre HRV's 4% Elevators 1% Gym HRV's 7%
Loads which are outside of the building were removed from the consumption calculations and not included in Figure 4. These loads include exterior lighting and electric vehicle charging.
The ‘Equipment’ submeter includes socket power from all spaces inside the building, the equipment in the Fitness and Spin rooms, plus the power consumption of the two comms data rooms.
Figure 4 provides a breakdown of the % electrical consumption of the building on a submeter-bysubmeter basis.Final efficiency is also a function of the ASHP average recover outlet temperature extracted from the building controls historic data, per Figure 8. The outlet temperature varies significantly based on operating mode i.e. whether the unit is in heating, cooling or simultaneous demand.
The lift is calculated per operating mode determined as a function of the heating and cooling degree day (HDD and CDD) fraction. Figure 9 shows the HDD and CDD for the site recorded weather data in 2022.
The equations are resolved with assumed heating and cooling setpoints calibrated to the actual system operation and by reviewing error metrics between the actual and modelled electrical demands, the setpoints are adjusted to provide the strongest possible correlation, see Figure 10.
The Normalized Mean Bias Error (NMBE) discrepancy between the actual and modeling plots is 0%, and the Coefficient of Variance of the Root Mean Square Error (CVRMSE) is 19%. This is in line with ASHRAE Guideline 14-2014 for monthly and hourly data calibration.
Derived Thermal Demand Profile for 2022
Derived Heating Thermal Demand, kWh Derived Cooling Thermal Demand, kWh
Based on the COP of heating, cooling and simultaneous demand at each condition the thermal energy demand intensity is calculated per Figure 11.
For a normalized comparison with the 2022 recorded data, adjustments were made to the PHPP design spreadsheet from 2017 to reflect how the building is being used, and what the recorded climate was for that period. Figure 13 compares the two PHPP calculations. Adjustments include:
A. Use of 2022 recorded weather from the nearest weather station.
• The design PHPP uses external dry bulb temperature data from a historical Vancouver weather file. Given PHPP uses monthly average mean temperatures, the peak temperature from the weather file was 18.4°C, see Figure 12..
• The adjusted PHPP uses recorded 2022 weather data from a local weather station, Abbotsford, see Figure 12. The peak temperature of this weather file is 21.1°C.
B. Heating and cooling setpoints reflect occupant usage. The Design PHPP was calculated with benchmark heating and cooling setpoints of 20°C and 25°C, respectively, in winter and summer. The reality is that occupants and facilities often choose somewhere in-between for the automated heating and cooling systems, which increases energy demand. In the case of the fitness room, it was observed that the setpoint was 6°C colder in cooling. This reflects one of the limitations of PHPP; that it has a global setpoint for the whole building.
• Removal of café that isn’t in operation
• Lighting hours match hours of operation of the facility
The total energy use of the normalized PHPP file increased from 27% from the original design calculations, per Figure 13, from 438.1 MWh/year total to 558.1 MWh/year total. Six of the eight energy end use categories showed a greater correlation with the actual 2022 data after the PHPP was adjusted. Lighting and pump energy were both identified as elements that require further study and optimization.