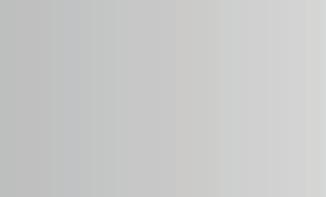


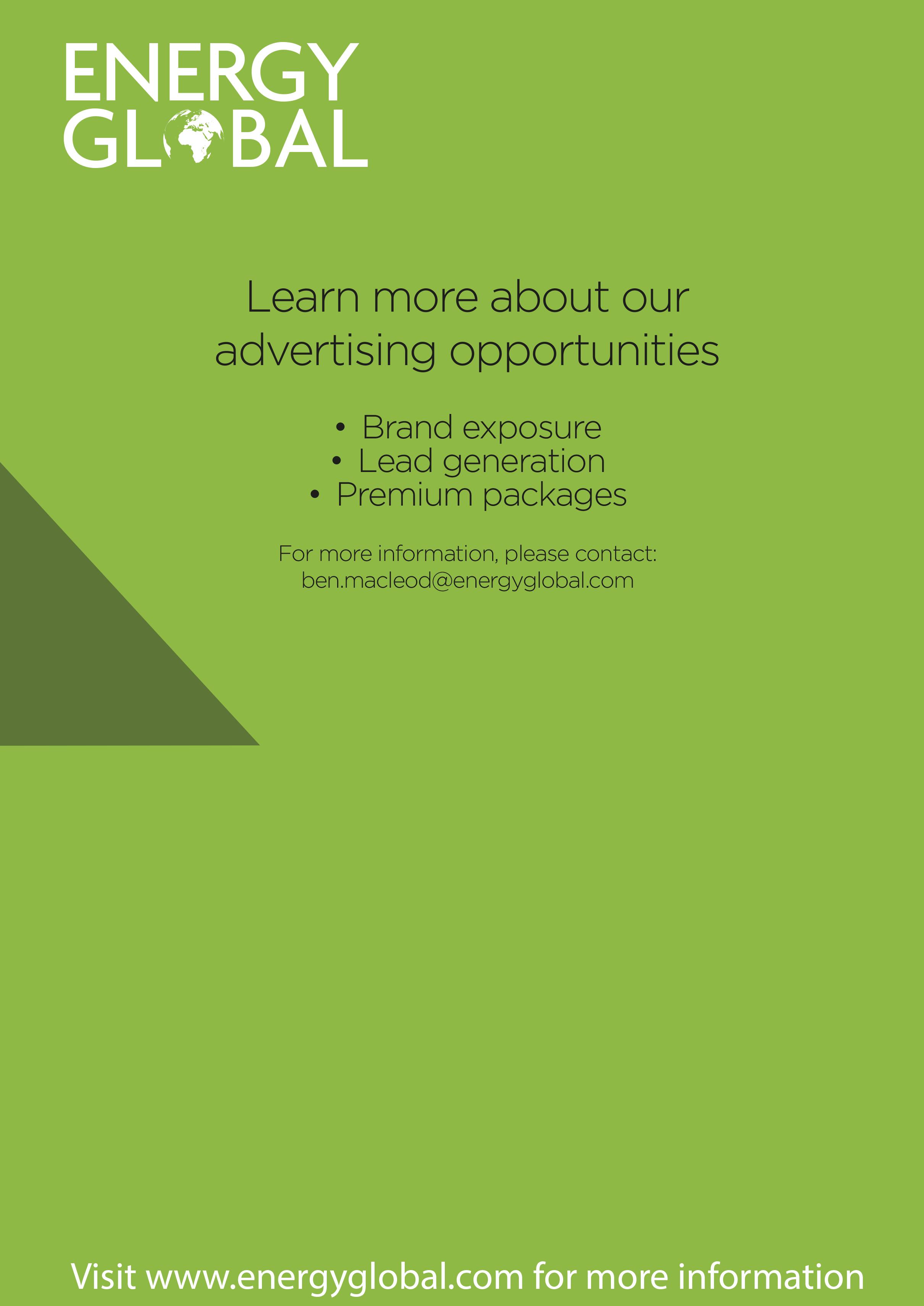
Guest
Naomi
means
10. Making wind wireless
Daniel Sangines, Energy Portfolio &
The changing solar landscape
Adam Bernadi, Burns & McDonnell, USA.
20. Small state, big potential
Martin Vogt, CEO, MPC Energy Solutions, the Netherlands.
24. Renewables and storage are better together
Jeff Damron, Wärtsilä’s Energy Storage & Optimisation Business, USA.
28. Power to the max
Upcycling industrial heat to cut the carbon.
With the urgency to fight climate change and end dependence on
Matt Roberts, Director of Renewable Projects, Emerson, USA.
32. Looking to the future
Michael Ducker, Senior Vice President and Head of Hydrogen Infrastructure, and Tom Cornell, Senior Vice President for Energy Storage Solutions, Mitsubishi Power Americas, USA.
40. Generate to accelerate Alastair Morris, Chief Commercial Officer, Powerstar, UK.
44. Time to transition Steve Wirtel, Kore Infrastructure, USA.
48. Waste not, want not David Richardson, Business Development Manager Global Renewables, Yokogawa, UK.
52. From wasteful to digital Simon Geiger, STEAG Energy Services, Germany.
58. Ride the wave Sam Leighton, Managing Director, Bombora Wave Energy, UK.
62. Pumped to be green Brian Minhinick, Mott MacDonald, Australia.
67. Seeing the bigger picture Chris Poynter, President, ABB System Drives, Switzerland.
70. Global news
The global floating solar market continues to remain a niche segment, but it is showing signs of strong growth prospects as the technology evolves and more stakeholders enter the market segment. In 2022, the total market is expected to be just shy of 4 GW direct current (GWdc), a 150% y/y growth.
Though it only accounts for 2% of the overall global solar demand, the compounded annual growth for the floating solar market is expected at 15% in the next 10 years, and the cumulative global installations are expected to surpass 58 GWdc.
The confluence of land scarcity, increased land costs, new market entrants, and growing market maturity will all contribute to the growth of the floating solar market. Offshore and near-shore floating photovoltaic (PV) developments are also gaining traction where in-land water bodies or their use for solar is limited.
Many new project announcements and capacity auctions are happening around the hybridisation of floating PV with hydropower. The two technologies can complement each other; during the dry season, floating solar can maximise production, and, during the wet season, hydro can be the majority power producer. Together, they can also help manage peak demand and smooth out the total power production. Co-location of floating solar with existing offshore wind projects can also help with cost amortisation as floating solar can take advantage of the existing transmission infrastructure.
The segment continues to evolve with disruptive solutions on all fronts of the component stack to make floating solar more adaptable and robust for water-based applications, where we’re seeing new market entrants and stakeholders develop innovative technologies to design such floating systems. Examples include dual glass modules, wave-dampening floats, and flexible mooring solutions.
The Asia-Pacific region continues as the largest market for floating solar. With 3 GWdc, the region captures over
93% of the total floating solar installations in 2022.
High population density leads to land scarcity, which in turn makes land acquisition costs high – one of the major demand drivers for floating solar in the region. CAPEX for floating solar is also one of the lowest in the region compared to others due to the availability of components that are made locally, as well as labour rates that are cheaper than most other regions.
China bolstered its lead with the commissioning of the 320 MW floating project by Huaneng Power International in the Dezhou region of the Shandong province at the start of 2022, which, at the time of writing, is currently the largest floating solar project in the world.
India, Indonesia, South Korea, Vietnam, and Thailand have all either executed large scale floating solar projects or have announced pipelines for the development of floating solar projects in the next 2 – 3 years.
With solar competing with agriculture for land use in Europe, developers have looked at floating solar as an alternate method to develop solar capacity in the region. France and the Netherlands continue to be leaders in the floating solar segment, sharing over 85% of the total floating solar capacity for Europe in 2022. This is expected to change as new markets, such as Spain and Portugal, also adopt and develop new floating solar projects.
Despite abundant land availability, the US is also exploring floating solar projects and is expected to have approximately 10% y/y growth in floating solar installations in 2022. Even though the cost of floating PV development is approximately 20 – 40% higher compared to ground-mounted solar, the elimination of land lease costs in urban areas that have a demand for solar can be a big driver for the adoption of floating PV.
With demand for solar increasing across the globe and developers looking for innovative ways to increase solar capacity, floating solar has the potential to emerge as the leader in the alternative segment due to all the benefits it can offer.
Low-carbon hydrogen plays a key role in ambitions for a renewable transition across Europe, in particular for hard-to-abate sectors such as heavy goods vehicles (HGV) transportation, heavy industry, and heating. The term ‘low-carbon hydrogen’ is widely understood to incorporate a number of ‘shades’ of the technology, which vary according to production method. This includes green hydrogen produced through electrolysis via renewables – such as solar photovoltaics (PV) and wind energy.1 Throughout Europe, and especially within the EU, green hydrogen has received significant attention. The EU announced its target of 40 GW of electrolyser capacity for green hydrogen by 2030 in its bloc-wide hydrogen strategy published in July 2020. 2 Drawing on findings from Cornwall Insight’s Low-carbon Hydrogen Index, 3 this article will explore the policy and commercial landscape for green hydrogen in countries across Europe. It will chart the emergence of supply chains as well as forward-looking government plans and present regulation.
At present, Germany is one of the global leaders in terms of hydrogen capacity targets, which increased from 5 – 10 GW by 2030 after the inauguration of the new Federal Government in late 2021. 4 The country also intends to be at the heart of development across the globe, having launched organisations and funding schemes aimed at encouraging investment in hydrogen projects abroad. 5
In its national hydrogen strategy, Germany has a clear focus on scaling-up green hydrogen domestically. 6 No subsidies are made available for the production of blue hydrogen, and all targets for
Naomi Potter, Cornwall Insight, UK, outlines how green hydrogen is playing a key role in the renewable transition across Europe, especially for hard-to-abate sectors.
capacity pertain only to the green shade of the technology. Domestically, the government maintains its commitment to fund and develop the green shade of the fuel.
This has come into conflict with key industry and government figures. In spite of any domestic debate, the sector is still developing at pace in Germany. As detailed in the Hydrogen Strategy for North Germany, a key advantage for the scale-up of low-carbon hydrogen across the country is that “the generation, temporary storage, distribution, and use of hydrogen are geographically concentrated.” 7 This clarity regarding demand centres and sources of supply offers confidence for developers and investors, allowing the German hydrogen supply chain to begin building out. For instance, transport networks – an area of concern for hydrogen industries across Europe –are already in construction in Germany as RWE and OGE’s H2ercules get underway to link the North Sea coast to industrial clusters in southern Germany. 8
The UK stands alongside Germany in having the highest capacity target for low-carbon hydrogen in Europe at 10 GW.9 A recent update on these ambitions included a commitment that ‘at least half is to come from electrolytic hydrogen’, meaning that the 10 GW is roughly split into a 5 GW target for blue hydrogen and 5 GW for green hydrogen.9 This technology agnostic approach stands in contrast to Germany, inviting criticism and debate amongst key players across the industry.
The UK’s funding schemes for low-carbon hydrogen are already in motion, with applications for the Net-Zero Hydrogen Fund Strand 1 and 2 closed and competition winners due to be announced later this year.10
Funding in both strands is focused on hydrogen production projects.
There are also plans for the next five years relating to the trialling and funding of demand-side projects; however, these are yet to get underway.11 Research across the sector, such as Cornwall Insight’s paper on the role for industrial decarbonisation, has helped to shed light on what sectors are likely to offer the strongest use cases in the UK and the routes-to-market for the technology.12 Moreover, some cross-supply chain partnerships are forming across the country, such as the recently announced Octopus Hydrogen and GeoPura joint project to use hydrogen as a replacement for diesel generators.13
One sector on the demand-side requiring more focus in the coming years is transport. At present, the country has 19 active low-carbon hydrogen refuelling stations, substantially behind Germany and France, which stand at 101 and 41 stations respectively.14 The UK Government Hydrogen Sector Action Plan lists transport as a key demand centre for low-carbon hydrogen fuel.15 However, more action is required in this sector to ensure a lack of refuelling stations does not hold back this potential key use-case for the technology, especially given the rapid scale-up and decreasing costs of electric vehicles.
France is third behind Germany and the UK in terms of electrolyser capacity targets, with 6.5 GW committed to by 2030.16 As in the UK, transport and industry are expected to be key demand centres with both being listed in France’s Hydrogen Strategy as priority areas for investment.16 As mentioned previously, France is
FigureTechnika przewiertów która w przyszłości napędzi rozwój HDD
Wind farms have become a mainstay of the energy transition in recent years, their potential is considered the most economical among renewable energies.
Trenchless installation of the power lines for connecting wind turbines and distributing
the green current makes it possible to tap this potential in an economically sensible and ecologically friendly way.
Besides, the reduced land requirements can make a valuable contribution to the acceptance of wind farms in the public.
further ahead than the UK in building out its transport infrastructure. Indeed, significant activity has been seen in the past five years with regional governments taking a key role. For instance, Auvergne-Rhône-Alpes installed a total of 14 refuelling stations on major roads, and there have also been key developments in rail transportation.17
Moreover, in 2019, the regions of Auvergne-Rhône-Alpes, Bourgogne-Franche-Comté, Grand Est, and Occitanie –through the public railway operator SNCF – signed the first order of 12 dual mode electric-hydrogen trains in France.18 Recent updates confirmed that these trains will make their first trial runs in 2024.18
France published its national hydrogen strategy in 2021 with a clear focus on domestic production of green hydrogen in regional valleys, as opposed to importing.16 President Emmanuel Macron has also come out more recently in support of hydrogen produced via nuclear energy; however, this shade of hydrogen does not directly feature in the national strategy.19
In southern Europe, countries such as Spain and Italy follow Germany’s lead in focusing largely on green hydrogen. All three countries have released hydrogen strategies and roadmaps with electrolyser capacity targets at 4 GW and 5 GW by 2030, respectively.
These countries have strong potential to play an instrumental role in importing hydrogen into Europe –especially from the African continent – as well as exporting generation. At present, technical and regulatory issues related to grid transportation of hydrogen pose a barrier to the successful implementation of this role. In Spain and Italy, the issue is largely regulatory, with a lack of suitable legislation to permit the injection of hydrogen into gas networks. By contrast, technical expertise is relatively strong in both countries with leading companies and organisations already operating in the area.20 In order to harness this advantage and take action on their potential for transporting hydrogen across Europe, focus needs to be on addressing the regulatory limitations for hydrogen networks.
Ireland is yet to publish its hydrogen strategy, but recent months have seen a boost of activity. On the policy and regulation side, the government announced in July 2022 that it had opened a consultation for a hydrogen strategy. 21 In May, on the project development side, energy firm EI-H2 announced that it is seeking planning permission for a 50 MW electrolysis plant in Aghada, County Cork, Ireland. Shortly after, GH2, a consortium of firms and organisations including NUI Galway, the Port of Galway, and SSE Renewables, announced plans for a hydrogen valley. 22
The country is well placed to take advantage of its ample offshore wind resources and access to water. In addition, Ireland has a modern gas network with polyethylene pipes which, early studies indicate, 23 are capable of injection and transportation of hydrogen through the grid. As with other European states, work needs to be done now to develop the regulation and technical expertise to match.
Across Europe, plans for low-carbon hydrogen scale-up are progressing at pace. Given recent concerns around gas supplies and impending target deadlines for net zero commitments taken by countries across Europe, the need to develop the low-carbon hydrogen sector is increasingly urgent. In order to respond to this, it is vital that countries ensure that regulations are appropriately updated and technical or supply chain limitations addressed. While each country will have its unique issues to respond to, ensuring the scale-up of infrastructure for demand-side and hydrogen networks are critical areas for focus in countries across the continent.
A comprehensive list of refences can be found of the Energy Global website: www.energyglobal.com/ special-reports/
Several factors are driving the creation of new opportunities to optimise wind farm operations. These include innovation in wind turbine technology and a new generation of sensors, as well as the ongoing acceleration in machine learning and artificial intelligence (AI).
Leveraging these developments in a new area of digitalisation will be key to success. Arguably the most crucial factor of all will be the ability to accurately capture and transport a growing volume of data in real time – both within wind farms and onward to data centres and their operators.
The growth in the volume of data is significant, and it is driving the requirement to provide low-cost wireless connectivity for new Internet of Things (IoT) devices. Where operators were previously relying on VHF, Tetra, and satellite-based technology solutions,
Daniel Sangines, Energy Portfolio & Solutions, Nokia, USA, explores how using LTE/4G technology can help to optimise wind farm operations.
they are now supplementing, or replacing, those systems with industry standards-based LTE/4G technology.
This exciting new generation of private wireless network technology has proved its worth in other challenging, complex, industrial environments, including mines, ports, and factories. The ability of LTE/4G to provide fast, accurate, and robust data delivery in these contexts implies significant operational and commercial benefits for operators.
Construction and operation of wind farms, onshore and particularly offshore, presents many obstacles similar to those that have been addressed and overcome in other challenging industrial environments.
The process of constructing an offshore wind farm is a challenging and hazardous endeavour. It begins with the laying of subsea cables that connect the area where the turbines are to be erected with the shore, and with an offshore substation that sits at the heart of the wind farm and transmits the generated power back to dry land.
Monopiles that will effectively act as the foundations for each wind turbine then have to be hammered into the seabed to a depth of up to 30 m. Once this process is complete, the cables, platforms, ladders, and all-important wind turbines can be installed.
It is worth emphasising just how hazardous this construction process is – and how important it is that the health and safety of the teams carrying out the work is treated as a top priority. At the same time, workforce enablement to ensure maximum productivity and the most seamless and efficient installation and ongoing maintenance process is also a key consideration.
Many of the tasks described above are carried out using remote-controlled subsea vehicles. The teams operating these machines and working onsite need the most robust broadband data capabilities, as well as high-definition video to ensure the efficient delivery and installation of all the components that make up a wind farm.
The boosted quality of communications delivered by the new generation of LTE/4G technology also brings benefits to voice communications between team members, as well as between the operatives on site and those in the operations centre on land.1
Once the wind farm is operational, the safety of on-site teams working many kilometres out to sea must continue to be a priority. The distance involved and the challenges posed by stormy weather conditions and high seas can pose a threat to the efficient performance of turbines and blades. Any delay during construction and interruption in ongoing operation is likely to have severe commercial implications.
Wind farm owners and operators have been quick to realise the benefits that can be delivered by digitalisation and automation. Fast, accurate data collection relating to the performance of the wind farms, and the use of that data to improve asset management, are extremely positive developments for operations working in this sphere.
Figure 1. Where operators were previously relying on VHF, Tetra, and satellite-based technology solutions, they are now supplementing or replacing those systems with industry standards-based LTE/4G technology.
The migration from VHF and Tetra to private wireless networks represents a significant upgrade in this context. Teams working to maintain the efficient operation of turbines and blades now have more efficient and reliable communication networks. The ability to monitor environmental conditions such as vibration, temperature, and humidity is a significant factor in the optimisation of operations, as well as safety and security.
Although Wi-Fi has seen notable improvements in the wake of the Wi-Fi 6 standard, private wireless networks represent a significant step forward for industrial operators such as the owners of wind farms. LTE/4G delivers extremely robust systems with high reliability, low latency, and elevated security standards.
Figure 2. LTE/4G technology can deliver pervasive connectivity for the entire site which can cover an extremely large area of land or sea. In the case of offshore wind farms, that coverage can be extended over the corridor from the site to the port.
This makes private wireless a logical choice for an installation such as a wind farm. LTE/4G technology can deliver pervasive connectivity for the entire site – which can cover an extremely large area of land or sea. In the case of offshore wind farms, that coverage can be extended over the corridor from the site to the port.
With the urgency to fight climate change and to end dependence on fossil fuels steadily growing, low-grade heat recycling has become a viable option to close formerly open energetic loops and enable a sustainable, circular economy. Industrial and high-performance heat pumps using turbocompressors support the upgrade of a waste or ambient heat source by increasing the temperature for further use in your process.
process
Utilization of ambient and process heat sources between 0-150°C
Serving capability of process heat range 10-50 MW (thermal) per unit and 80-300°C
QR code
learn more
Given their efficacy in other challenging industrial environments, it should be no surprise that private wireless networks offer a considerable performance upgrade for wind farm operators. Even as wind turbines grow in number and capacity, and are deployed ever further from shore to meet ever-increasing demand for energy, the suitability of reliable, robust private wireless networks becomes ever more apparent.
Such networks can connect assets many kilometres apart while facilitating the transfer of data with low latency and high bandwidth. Private wireless antennae are installed on the offshore platform, while data is transferred back to shore, initially by a microwave radio link and then by an undersea fibre optical cable. The microwave link is retained as a back-up to ensure communication continues if there are any issues with the cable.
Workers at the substation, turbines, or on service vessels can access expert input from the shore via real-time video calls. The enhanced connectivity also enables faster diagnosis and repair of issues, as well as underpinning the health and safety of those located at the site. Field technicians can, for the first time, provide a clear picture from inside turbine structures that enable operations staff to monitor conditions and effect any repairs required.
The requirement for ever more robust solutions to match the growth in size and number of increasingly remote wind farms is unlikely to slacken any time soon. Operators of these super-sized farms will be eager to keep maintenance windows small and costs manageable, whilst still delivering optimal performance and avoiding expensive technical hitches.
Private wireless networks represent a major stride forward in providing the required connectivity to enable enhanced remote monitoring capabilities beyond those with existing SCADA networks. The application of smart technology, such as AI, machine learning, and digital twins, ensures the data collected remotely can be used to upgrade maintenance programmes.
For instance, data can be analysed in real time and compared with business-as-usual operations to highlight any anomalies so that they can be addressed before any equipment is damaged or any outages occur. An operative can work in the nacelle of a turbine and, through a pair of smart glasses, analyse a particular component while receiving real-time data from the device.
However, the benefits delivered by private wireless networks to the operators of offshore wind farms are not restricted simply to costs and maintenance. They can also deliver performance improvements in the turbines themselves. A new generation of sensors and monitoring equipment is in the pipeline, which will collect operational data that can in turn be used to further optimise the performance of those assets.
With a private wireless network, it is possible to monitor the turbine sensors several times each second and use the collected data to highlight and address areas including structural changes, acoustics, temperature, and even lightning strikes.
A further significant consideration is the quality of life of the staff who might spend several weeks at a time living on service vessels far from shore. The private wireless network that covers the wind farm and its associated corridor back to port should also extend to those vessels, allowing those on board to make video calls home, access the internet, and stream a TV programme or movie.
One of the most reassuring benefits of private wireless networks is that they incorporate a significant degree of future-proofing that will ensure the seamless integration of new data collection, automation, and maintenance capabilities. Evolution to 5G will be straightforward once the use cases arise that necessitate such a switch.
As new wind farms are deployed, or as new turbines are added to installations that already benefit from LTE/4G efficiencies, they will be easily assimilated into the network. That means maintenance work can be planned that uses digital twins and AR technology to simulate scenarios and alterations that can then be deployed in the real world.
Training of staff can also take place on site. New recruits get the opportunity to familiarise themselves with the equipment they will be working on, while experienced staff can refresh their knowledge of existing turbines and other pieces of kit.
This leads on to another important consideration. Private wireless networks are ideal, not only for new wind farms, but for those that have been in place for some time. Their presence addresses challenges when retrofitting older wind turbines with a new generation of monitoring capabilities by easily getting higher frequency data to applications and users. Existing installations can be retro-fitted with the latest technology to ensure that their owners and workers enjoy the same enhancements in safety and performance – and that the existing farms operate as efficiently as the newer facilities in the same ownership.
The phrase ‘a perfect storm’ is unlikely to find much favour with people who own, construct, and work on wind farms. However, it describes perfectly the circumstances surrounding such installations now and in the near future when it comes to private wireless networks.
The remote location of wind farms – particularly in the case of offshore sites – presents a challenge to operators who need robust communications and an ultra-reliable delivery of data that can be used to ensure optimal ongoing generation, as well as the maintenance of high health and safety standards.
Utilising a robust, cutting-edge solution with LTE/4G and, in the imminent future, 5G, ensures that such targets will be met and exceeded through the use of this evolutionary and revolutionary technology.2
1. ‘Renewable energy’, Nokia, (Accessed on 12/07/22).
2. Nokia, ‘Keeping Belgian North Sea wind farms connected’, Power Technology (October 2020).
he solar market in the US has been continually ramping up over the last handful of years. According to the latest update from the Solar Energy Industries Association, at the end of 1Q22, the US had installed enough solar photovoltaic (PV) capacity to power 22 million American homes.1 Additionally, of all the new electricity-generating capacity added in the first quarter, solar accounted for 50%.
Despite the impressive numbers, the solar market was not able to reach its full potential in 1Q22 due to an unexpected tariff investigation on the import of solar cells and modules originating from Cambodia, Malaysia, Thailand, and Vietnam. This uncertainty – along with increasing costs for materials, labour, and transportation – increased the overall capital cost of new solar installations.
In June 2022, however, solar companies welcomed the federal government’s announcement of emergency measures to increase critical supplies to US solar manufacturers, declaring a two-year tariff exemption on solar panels from Southeast Asia.
Adam Bernadi,Burns &McDonnell,USA, outlinesthewaysinwhichthe solar industry is booming in
With this change, new solar projects can achieve the full-speed-ahead rate that the industry had previously been operating under in the last few years, and can continue supporting the increased decarbonisation goals that are ramping up all over the country.
Widening the door for solar Companies and investors are becoming more driven to support businesses that prioritise environmental, social, and governance (ESG) opportunities. This new development is changing the way some companies with larger carbon footprints, such as oil and gas companies, operate, and is expanding what they spend their time and energy on, such as renewable generation.
From cost savings and competitive options, to investment tax credits and attraction of investors, companies from all industries are looking to expand their typical operations to include the integration of renewable generation assets. These renewable projects can also offer carbon tax reductions that can help offset some emissions from the oil and gas facilities.
discusses
grow furth er .
Although the benefits that can be gained from implementing renewable sources such as solar are clear, making the transition can be a little more complex than it may seem. For example, heavy industrial and manufacturing companies cannot rely on solar 24/7 because the sun is not always shining. This means these companies would likely need to include dispatchable sources to bridge those gaps when solar cannot be utilised.
With the vast increase in interest surrounding new solar projects, it is crucial that efficiencies are created that can support fast and seamless implementation. With some solar projects covering hundreds and sometimes thousands of acres, efficiency is key.
There are many moving parts and pieces involved in completing a successful solar project. For example, a standard solar project can include site selection, geotechnical investigation, pile design, pile installation, pile corrosion design, underground cable sizing, equipment selection, module installation, wire management, construction, SCADA requirements, and more.
As more solar projects are completed, tailored applications and proficiencies can be used for the repetitive actions involved in installing hundreds of PV panels. Oftentimes, an engineering-led EPC team can help utility owners navigate the challenges and difficult-to-meet schedules involved in these projects.
Integrated EPC has a demonstrated track record for improved safety, quality, cost control, and schedule certainty. As of late, it is also evolving to drive innovations in digital technology applications applied to work on solar project sites.
The engineering team often will begin with a geospatial survey of the topography, which is then loaded into AutoCAD to build a comprehensive layout with detailed locations of each pile. From there, the team can utilise digital instructions to set the bracket heights for the piles, eliminating the need for the typical lasers and string line processes.
Using integrated EPC and updated digital technology applications can help improve pile installation for solar projects by reducing the amount of rework needed, shaving time from the schedule and reducing costs because fewer workers are needed on site.
Additionally, one of the greatest benefits of EPC combined with innovative technology applications is the improved communication throughout the project. With the flexibility of EPC contracting and the data at hand, the construction and engineering teams are enabled to collaborate and develop solutions that are continually improving project deliverables.
As both need and demand for solar continue to increase, energy storage systems are being installed to meet electrical grid challenges that come with an increase in renewable sources.
Solar and storage together are a very powerful combination. This is in part because storage can be used to better
Figure 1 . Construction of Vistra’s 65 MWdc Brightside solar facility. Burns & McDonnell served as the integrated EPC contractor for this project, which helped alleviate supply chain and logistics challenges. Figure 2 Solar projects are not just being constructed in warmer environments. Burns & McDonnell is currently the EPC contractor for Wisconsin Power & Light’s solar programme as they look to construct approximately 1100 MW/dc of solar in Wisconsin as part of the company’s larger Clean Energy Blueprint.allow solar to contribute to the power supply even when the sun is not shining. Storage is also important in helping to fill in the gaps as more baseload units start to retire.
There are many types of energy storage options attracting attention in today’s market, including lithium-ion batteries, redox flow batteries, hydrogen, thermal, and LNG. The electric vehicle market continues to be the driving force for lithium-ion technology advances and market pricing. As the power industry evolves and works toward meeting decarbonisation goals, storage technologies – and solar – have enormous opportunities for growth and utilisation on the horizon.
Alliant Energy, a public utility that provides power to businesses and residents in Iowa and Wisconsin, the US, hired Burns & McDonnell to provide EPC services for its solar programme in Wisconsin. Alliant Energy’s Clean Energy Blueprint programme was developed to help accelerate the transition to renewable energy in Wisconsin. In total, the programme involves 12 projects, with nine of the 12 projects being executed by Burns & McDonnell. The solar development programme is estimated to create thousands of new construction jobs in the area, while providing reliable energy for years to come.
For one of those projects – a 1200 acre, 150 MW solar project in Wood County, Wisconsin – Burns & McDonnell developed electrical designs and environmental studies for
and civil designs for transmission and distribution power lines, PV modules, and substations. The PV modules in the solar panels are made up of silicon semi-conductors that absorb sunlight and produce electric current.
As part of the project, AZCO, a Burns & McDonnell subsidiary, is providing pile driving, equipment and electrical installation, and steel fabrication services.
With the rareness of one firm providing the full spectrum of services from start to finish, Burns & McDonnell was able to keep this and other projects within the programme on schedule despite challenges involving the COVID-19 pandemic, market volatility, and supply chain issues. The EPC approach allowed the firm to utilise internal procurement, installation, and commissioning experience and insights in order to maintain and streamline processes.
Beyond the typical project responsibilities, such as maintaining the timeline and staying on budget, the firm was dedicated to building positive relationships with members of the Wood County community. This includes educational opportunities and tours for those who want to learn more about the project and the overall programme.
Upon completion, the 12 Alliant Energy solar projects will span nine Wisconsin counties and be able to generate approximately 1100 MW of power, enough to serve nearly 300 000 homes.
Martin Vogt, CEO, MPC Energy Solutions, the Netherlands, explores how decarbonisation can help small island developing states achieve energy independence, focusing specifically on solar photovoltaics and battery energy storage systems.
itigating climate change has become a top priority for countries worldwide.
For those small island developing states (SIDS) who are the most exposed to climate change impacts, it constitutes a requirement for survival. The intensifying weather patterns present a climate emergency for these most vulnerable of lands, as hurricanes, flooding, landslides, and rising sea levels cause imminent threats to infrastructure, communities, and businesses. Although, on average, SIDS only contribute 1.5% towards the world’s greenhouse gas (GHG) emissions, this vulnerability to climate is galvanising SIDS’ governments to take action and mitigate emissions and decarbonise, including transitioning to cleaner energy generation.1
SIDS face a unique set of challenges, such as being burdened by some of the world’s highest energy costs, due to their remote locations. 2 In fact, this has contributed to them being among the most indebted countries in the world. 2 Many islanders are also among the estimated 10% of the global population that still does not have electricity, due to the challenging topographies of their homeland. 3 Particularly in the Pacific Island nations, approximately 7.5 million people live without access to electricity. 4
Additionally, despite being enriched with renewable energy sources such as sunlight and wind, SIDS are still heavily reliant on foreign oil and gas imported from exporting nations to meet growing power demands. Some islands already possess a significant share of hydropower or solar energy, such as Dominica, Papua New Guinea, and Kiribati; however, across SIDS, fuel imports constitute an average of 5 – 10% of their GDP. 5 SIDS spend over 30% of their foreign exchange earnings per year on energy imports, 6 which account for their highest share of imports, and their transportation and electricity generation is still almost completely contingent on oil imports.7
During a global crisis (such as those caused by the COVID-19 pandemic or the war in Ukraine), this reliance on fuel imports makes SIDS even more vulnerable to international supply chain disruption, volatility in oil and gas prices, and access to reliable supply. Furthermore, trade and business have been disrupted, with the tourism sector upended,
productivity taking a hit, and, ultimately, causing major losses across revenue streams.
When taken together, island nations’ decarbonisation efforts, rising global inflation, potential disruptions to oil supplies, and exposure to unstable fossil fuel prices all make it imperative for SIDS to transition to green energy sources. Breaking with legacy fuels and taking advantage of solar power and energy storage systems will provide greater energy independence and continued sustainable development.
Decarbonising with solar photovoltaics and battery energy storage systems
Renewable energy sources across the Caribbean and Pacific Island regions provide the potential to boost decarbonisation and green energy investment. There are clear, direct economic advantages to reducing imported supplies of non-renewables, increasing the role of solar photovoltaics (PV) in their energy mix and successfully transitioning towards a net zero emission future.
According to the policy institute climate analytics, in the world’s sunniest regions, 1 MW of solar power produces approximately 1.3 – 1.7 GWh/yr, with each GWh replacing 1 GWh of oil-based energy, for which 250 000 l of fuel are required at a representative cost of US$00.60/l. As a result, each 1 MW of solar power installed saves approximately US$150 000/yr throughout its minimum 25-year lifetime, compared to fuel-based energy.5
Additionally, between 2010 and 2020, the clean power industry reported a strong fall in overall generation costs, with electricity costs from utility scale solar PV decreasing by 85%.8 This means renewables are now more cost-effective than the cheapest fossil fuels, and the trend is expected to continue. Despite the initial CAPEX costs of solar PV infrastructure, the cost-benefit long-term analysis is extremely favourable. As an example, the cheapest source of power in Jamaica is a 51 MWp solar park. This solar park sells its power at approximately US$8.60/kWh, which is approximately 50 – 60% cheaper than the average thermal generation costs on the island. It is no surprise that Jamaica wants to expand its renewable energy capacity from approximately 120 MW today to 450 MW by 2025.
It is true that solar PV often cannot provide the level of baseload capacity that fossil fuels can. Energy generation from renewable sources can be unpredictable and output can vary depending on the availability of natural resources. In answer to this, variable energy sources can work in tandem with battery storage, which with dispatchable technology provides power stability by enabling the storage of excess energy produced by intermittent sources. Battery energy storage systems (BESS) can also increase renewables capacity potential and help grid operators balance supply with demand.
For the SIDS who struggle to secure universal energy access, such decentralised clean energy systems can prove a crucial ally to communities who live in remote locations without access to energy from a centralised electric power grid. Decentralised energy supply also usually means higher resilience in the event of adverse weather, such as hurricanes.
Many Caribbean islands have storage projects in the operation or planning stage. A ‘lighthouse’ project for the Caribbean is currently underway in St. Kitts and Nevis. This will become the first operational utility scale solar PV and battery storage hybrid project in the region. The nation is committed to diversifying energy generation away from the 94% of its electricity that comes from diesel. The small nation has an ambitious drive to boost its current 6% from green sources to one-third decarbonisation of the island’s energy supply, achieved through a 35.7 MWp solar PV and 18.2 MW BESS project.
The costs of battery storage have also seen a sharp drop in recent years. Solar PV combined with storage is already competitive with fossil fuel generation, considering the high fuel costs and often outdated power generation facilities in SIDS. BESS continues to hold major deployment and cost-reduction potential. There are clear opportunities for energy transition and investment in SIDS nations to be capitalised on. The utility in St. Kitts will save approximately 25 – 30% of annual costs receiving and storing power from its combined solar PV and battery project compared to the diesel-fuelled generators used to date.
SIDS offer favourable conditions and considerable market potential for solar PV and storage systems. This type of hybrid project requires a detailed analysis of the application case and is ultimately a tailor-made solution to meet the
local requirements of the utility grid operator and regulator. To create a win-win situation for all stakeholders, it may be challenging to find the optimum balance between their advantages and costs. Small states must consider a competitive usage of limited land and the system’s cost. It is also equally important to reflect upon the resilience of their energy systems, grid stability, flexible power production, and long-term planning. These are essential to meet higher energy demand, as well as guaranteed energy supply during events that change the typical consumption behaviours.
Technology such as BESS can have significant initial investment and infrastructure costs. Additionally, BESS allows a significant percentage of variable but cost-free natural resources such as sun, wind, or hydro to be integrated into the grid.
Independent power producers (IPPs) can play an important role in the deployment of hybrid systems. Across the Caribbean, including Jamaica, Barbados, and the Dominican Republic, the vast majority of renewable energy assets are owned by IPPs who often have significant experience in designing, financing, building, and operating renewable energy and storage facilities in their portfolio. Therefore, they offer economies of scale compared to standalone operations. For instance, the lower costs of debt financing, insurance, operations, maintenance, and asset management ultimately reduce the power and storage costs, resulting in the lowest possible levelised cost of energy (LCOE) and reduced prices for the end consumer. Private sector investment also helps local job creation and stronger community engagement.
To create a favourable environment for hybrid energy solutions in SIDS, it is also imperative to establish encouraging market conditions for renewables or BESS as well as strong government support. Strategic long-term planning is needed here, including the formulation of clear roadmaps and stable, long-term oriented regulatory frameworks that encourage confidence among the private sector. Lifting regulatory barriers will also free small, clean power developers to plan and finance lasting projects.
Across SIDS, public-private partnerships are needed to enable investors to deploy private sector capital in battery system projects. This, paired with stable tax waiver policies, such as VAT exemptions for the import of renewable
Figure 1 CGI image of 35.6 MW solar energy plant and 44.2 MWh battery storage facility currently under development in the Basseterre Valley, St. Kitts.Figure 2 The 35.6 MW solar energy plant and 44.2 MWh battery storage facility currently under development in St. Kitts will be able to convert intermittent renewable energy to baseload power through Leclanché’s energy management system (EMS).
energy systems, can offer great opportunities for IPPs looking to capitalise on the islands’ energy transition.
There is significant potential for further investment in the sector, which can be achieved by creating an attractive environment for foreign investors. By doing this, governments can lift the burden of public sector spending.
Crucially, clean energy empowers SIDS with greater autonomy and self-reliance, meaning governments can vastly reduce fossil fuel imports, thereby creating greater stability and resilience for the island nations. The Cayman Islands provides a fine example of opening new tenders through a recent auction scheme to stimulate an investment wave in solar and wind power production over the next 10 years. This is angled toward the islands achieving carbon neutrality and meeting a target of 70% of renewable energy by 2037.
The support and involvement of local communities is also absolutely essential to ensure the continued sustainable development of SIDS. In the path to a low-emission and climate-resilient future, decarbonisation provides a pathway to greater self-determination and freedom from the vulnerabilities of foreign-owned oil and gas. Conversely, it may be daunting and politically challenging to rely on a renewable energy system managed by foreign parties.
Private sector investors must therefore provide a compelling case for further investment into projects. They can achieve this by demonstrating how sustainable energy
for all cannot only help combat climate change and promote sustainable development, but also provide a better standard of living by creating economic opportunities and eradicating poverty. A dialogue with the island public is essential, and priority should be given to transparency through, for example, public consultations, workshops, and training.
With a holistic approach, renewable energy projects are able to power entire island communities, aiding their transformation, reducing their overall carbon footprint, and sending a strong signal to the world that a zero-carbon future is possible.
1. ‘Climate change, small island developing States,’ United Nations Framework Convention on Climate Change, (2005).
2. FEINSTEIN, C., ‘SIDS – Towards a Sustainable Energy Future,’ World Bank Group, (June 2014).
3. ‘SDG7: Data and Projections – Access to electricity,’ International Energy Agency, (2022).
4. CAVANOUGH, E., ‘Islands of Opportunity: Stepping Up to the Pacific’s Energy Challenge,’ The McKell Institute, (May 2019).
5. ATTARD, M-C., BRECHA, R., FYSON, C., KIM, J., SINDT, J., FULLER, F., and JONES, D., ‘Long-term strategies in SIDS: blueprints for decarbonised and resilient 1.5˚C compatible economies,’ Climate Analytics, (May 2021).
6. ASARIOTIS, R., ‘Climate Change Impacts and Adaptation for Coastal Transport Infrastructure in Caribbean SIDS – Background and introduction of the project objectives and context,’ UNCTAD National Workshop Jamaica, (June 2017).
7. MEAD, L., ‘Small Islands, Large Oceans: Voices on the Frontlines of Climate Change,’ International Institute for Sustainable Development, (March 2021).
8. ‘Renewable Power Generation Costs in 2020,’ International Renewable Energy Agency, (2021).
he growth of intermittent renewable energy across the globe has necessitated the deployment of energy storage technologies to fully replace fossil fuels with clean, dispatchable, and reliable power. According to IHS Markit, the share of co-located onshore wind, solar, and energy storage is projected to increase from 14% in 2021 to 35% by 2030 across the globe. Co-located systems combine two or more energy assets, a grid connection, land, substation, and power electronics, as well as permitting procedures, operations, and maintenance work. This set-up can provide several operational and financial advantages for asset owners while accelerating renewables deployment and improving grid stability.
The untapped value of co-location Developers in markets with high shares of wind and solar in the power mix can benefit from the additional revenue streams afforded by co-location. Capture prices for renewable energy are expected to decrease significantly as capacity grows, meaning that the price captured on the market by solar or onshore wind generators is eroded over time. The term ‘capture price’ relates to the actual electricity price achieved by a generator in the market, as compared to the wholesale price. Co-location mitigates this price cannibalisation, as energy storage allows asset owners to sell power during high-value peak periods and get paid for performing ancillary grid services.
This can be seen in Germany, where capture prices for standalone solar assets are expected to fall by 73% between 2022 and 2030, according to IHS Markit. Energy storage systems can help to recoup losses. For example, a 22 MW storage facility developed by Enel Green Power, ENERTRAG AG, Leclanché, and Wärtsilä in Cremzow, Germany, provides frequency regulation, energy arbitrage, and reactive power services.
As demand for clean energy 24 hours a day increases, many of the world’s top renewables developers are pursuing hybrid developments as a key competitive advantage. Hybrid plants lower the risk of underperformance because output is not limited to a single generation source. This offers a future-proofed investment that is better equipped to handle market changes and could result in additional financing for the energy transition.
Co-location can also help accelerate the deployment of renewable assets in markets with land-use constraints and high grid connection costs. This is the case in the US, where there is a 1300 GW backlog of clean energy projects awaiting interconnection to transmission grids.1 Since 2015, interconnection upgrade costs have grown from approximately 10% of project costs to between 50 – 100%.2 Developers can save up to 10% of balance-of-system costs compared to standalone projects, according to analysis by IHS Markit, by leveraging synergies across grid connection, land acquisition, and materials procurement. Co-location increases the utilisation of a single point of grid connection, which is a more efficient use of existing infrastructure – especially in places that desperately need more transmission and distribution investment.
Solar and wind facilities can be co-located with energy storage as new-builds or added retroactively. For example,
Wärtsilä and Clearway Energy Group are developing two new solar-plus-storage facilities in San Bernardino, California, the US, totalling 482 MW of solar and 275 MW/1.1 GWh of energy storage. Combined, they will be among the largest solar-plus-storage facilities in the world. At the same time, Wärtsilä is retroactively connecting a 147 MW/588 MWh energy storage system to one of Clearway’s operating solar facilities in Kern County, California.
Repowering existing wind plants, the process of replacing older turbines with newer ones also presents an opportunity to retroactively co-locate storage. IHS Markit predicts approximately 21.7 GW of wind capacity is expected to be repowered in the US and Europe. Advancements in turbine technology has allowed for the use of fewer turbines or smaller turbines to generate equivalent or even greater capacity than that of the original wind farm. This can reduce the amount of space needed for the wind farm, freeing up already permitted areas for more renewable energy assets.
Co-located configurations are also particularly attractive for islands and off-grid energy systems, such as remote mining, which typically rely on imported fuels, such as oil or diesel, for power generation. Last year, the members of the International Council on Mining and Metals, which consists of 28 top global mining companies, announced plans to achieve net zero emissions from mining operations by 2050. Employing renewables and storage can significantly reduce greenhouse gas (GHG) emissions while supplying a more stable source of electricity.
Independent power producer Zenith Energy demonstrated this approach in Australia, one of the world’s largest markets to produce mining materials. The company is leveraging
Figurea 9.2 MW/8.7 MWh energy storage system to decarbonise operations for mining companies. Adding storage saves the existing dual fuel engine power plant from frequent ramping by managing the power fluctuations of the mining site, therefore improving the operational efficiency, reducing fuel costs, and lowering the mine’s carbon footprint.
Many islands, such as Hawaii, the US, have dramatically increased their share of renewable energy to achieve decarbonisation targets and lower costs of fuel imports. As of 2018, Hawaii meets 28% of its energy demand from renewable energy. Energy storage will play a key role in balancing the power system in Hawaii and maximising the value of renewable resources. The island of Oahu welcomed its first large scale solar-plus-storage facilities this summer and will bring on a second by the end of the year to ensure reliable delivery of electricity and contribute to Hawaii’s goal of 100% renewable energy by 2045.
Cumulative installations of co-located wind, solar, and storage hybrid capacity is expected to reach 560 GW across eight key markets between 2021 and 2030, based on research by IHS Markit. In each market, there are more planned solar-plus-storage projects than wind-plus-storage. For example, of the 365 GW of hybrid renewables that will be installed in mainland China, 24% are solar-plus-storage and 10% are wind-plus-storage. In the US, wind-plus-storage only represents 4% of total planned hybrid renewable installations, whereas solar-plus-storage represents 67%.
Although wind-plus-storage facilities offer similar benefits, deployment thus far has been significantly lower than solar-plus-storage. One reason for this disparity is the difference in intermittency levels between solar and wind power. Wind generation has greater variability in output and does not follow predictable patterns like solar – since the sun rises and sets each day. Wind plants face longer periods of low or no production that are not always easy to forecast. The amount of energy that must be stored and shifted from a wind plant to offer an economic use of energy storage is high, given the longer periods of low production and the fact that wind plants are typically most productive during off-peak hours.
Regulatory policy and market frameworks also play an important role in ensuring the maximum value and benefit of hybrid plants. In the US, for example, most wind facilities take advantage of the federal production tax credit (PTC), a per-kilowatt hour (kWh) credit for electricity generated by eligible renewable sources. The US also offers an investment tax credit (ITC) for renewable energy projects which reduce upfront costs. Developers can claim the ITC for energy storage projects that are co-located with wind and solar. But developers cannot claim both, and the PTC is almost always more lucrative for wind projects. This explains why 63% of storage capacity planned to come online from 2021 to 2024 in the US will be paired with solar.3
The PTC was phased out at the end of 2021, which would have made adding energy storage more attractive to wind developers for its ability to participate in competitive markets and generate additional sources of revenue. But under the new Inflation Reduction Act, the PTC has been extended to renewable projects
beginning construction before 1 January 2025. It also increased the ITC from 26 to 30%, and its definition has been expanded to include standalone energy storage projects for the first time. The revamped tax incentives will certainly accelerate overall renewables and energy storage deployments, but it remains to be seen how they will reshape the make-up of hybrid projects.
Developers and integrators have uncovered new ways to improve the design of co-located facilities in response to growing popularity. For example, increasingly solar-plus-storage projects are DC-coupled instead of AC-coupled. In an AC-coupled system, the battery system and solar plant are connected to separate inverters in parallel. In a DC-coupled system, solar generation flows directly to the battery via a DC/DC converter. Reducing the number of AC/DC conversions makes DC-coupled systems approximately 3% more efficient than AC-coupled systems. However, AC-coupling allows for more market revenue flexibility since the storage system can be used directly for market-bidding if not needed for the solar plant. This same trend is not being seen towards DC-coupling with wind assets and energy storage, likely because wind turbines naturally produce an alternating current.
Still, innovative developers are steadily making headway with co-located wind and storage developments. Enel Green Power recently completed its first large scale hybrid wind project called Azure Sky in Texas, the US, which consists of 350 MW of wind capacity and 137 MW/205 MWh of battery storage capacity provided by Wärtsilä. Enel plans to develop a total of 12 battery facilities in Texas totalling 1290 MWh of storage capacity by early 2023.
Co-location reinforces the need for sophisticated software to seamlessly integrate and manage multiple types of renewable assets. Energy management software plays a key role in the successful management of hybrid renewable-plus-storage facilities. Wärtsilä’s sophisticated GEMS Digital Energy Platform monitors, controls, and optimises individual resources and entire fleets, including energy storage technology, renewables, and balancing thermal generation assets.
Software is needed to oversee and control hybrid plant functions in optimised ways to maximise the value of the facility. Plus, as developers seek to stack multiple revenue streams with hybrid facilities, software can navigate dynamic market operations according to objectives, portfolio constraints, market trends, and uncertainties.
It is expected that there will be additional innovative applications of GEMS as more developers discover the benefits of energy storage co-location and hybrid renewable-plus-storage systems. Wärtsilä continues to share these learnings with power producers and developers to highlight the role of energy storage in the transition to a 100% renewable energy system.
1. ‘Record Amounts of Zero-carbon Electricity Generation and Storage Now Seeking Grid Interconnection’, Berkeley Lab, (April 2022).
2. CASPARY, J., GOGGIN, M., GRAMLICH, R., and SCHNEIDER, J., ‘Disconnected: The Need for a New Generator Interconnection Policy’, Americans for a Clean Energy Grid, (January 2021).
3. LINGA, V., ‘Most planned U.S. battery storage additions in next three years to be paired with solar’, U.S. Energy Information Administration, (September 2021).
Matt Roberts, Director of Renewable Projects, Emerson, USA, outlines the importance of energy storage within the renewable energy industry, highlighting the ways in which energy management can be made more efficient in order to maximise performance and profitability.
he energy landscape is changing as governments and businesses alike work toward ambitious sustainability goals, including net zero. A December 2021 International Energy Agency (IEA) report titled Renewables 2021: Analysis and Forecast to 2026 bears this out, predicting renewables will comprise approximately 95% of the increase in global power capacity through 2026.1
But producing energy only when the sun shines, the water flows, and the wind blows is simply not enough. The world’s increasing reliance on renewables comes with new challenges inherent when working with intermittent power sources, bringing into sharp focus the need for energy storage solutions, such as green hydrogen and utility scale lithium-ion batteries, that can turn variable sources into reliable, dispatchable generation. Consider this:
F Adding battery energy storage to wind and solar photovoltaic (PV) allows variable clean energy to be utilised during off-hours, providing greater value.
F Adding battery energy storage to run-of-the-river hydropower plants creates ‘virtual reservoirs’ that can act like conventional hydropower plants to provide baseload stability, or even a microgrid that supports local sustainability and power needs during blackouts.
F Short- and long-duration storage of green hydrogen derived from purely renewable sources enables dispatch of the gas when required to generate clean electricity from hydrogen-fuelled turbines.
BloombergNEF predicts that the global energy storage market is set to hit 1 TWh by 2030, with the US and China leading with over half of the global installations by the end of this decade.2 Energy storage installations around the world will reach a cumulative 358 GW/1028 GWh by the end of 2030, more than 20 times larger than what was online by the end of 2020.
The projected growth of the global hydrogen energy storage market is just as staggering, reaching US$119.2 billion by 2027 according to the latest MarketsandMarkets research report.3 Commercialisation of power-to-gas technologies is the next big thing, adding flexibility to energy systems and allowing efficient integration of unstable renewables in the energy markets.
With so much at stake, from standalone energy storage systems to hybrid applications that include solar, wind, hydropower, and green hydrogen, green energy power producers are looking for ways to better manage storage operations. The foundation for better management lies in a solution that centralises and integrates critical information to provide full operational visibility across all assets, thereby making it possible to achieve increased performance, improved efficiency, and reduced costs.
The gathering, contextualising, and secure delivery of real-time and historical asset data to key stakeholders provides accurate, actionable intelligence that enables better decision-making and higher revenues.
A closer look at battery energy storage reveals the many ways that energy management software and technologies can contribute to maximising performance and profitability.
F Energy arbitrage: Automatically scheduling the battery energy storage system (BESS) to charge during low-cost periods and
discharge at high-price times optimises energy arbitrage and maximises revenue.
F Load-levelling: Efficiently co-ordinating the dispatch of battery-stored energy reduces the load on peak-generating sources by directing the battery management system to charge and store power during periods of excess generation, and discharge or deliver the power during periods of excess demand.
F Grid stability: Using battery storage for grid services such as frequency response and voltage regulation contributes to grid stability, as it makes it possible to quickly respond to short-term imbalances in supply and demand using active (frequency) or reactive (voltage) control.
F Reserves: Deploying emission-free battery storage as a form of spinning, non-spinning, or supplemental reserves makes it possible to maintain reliable power system operations. Close monitoring of the state-of-charge confirms dispatch readiness to quickly deliver stored power in response to contingency events, such as an outage, or during periods of high demand.
Figure 1 . A single platform integrates many data sources across an entire renewable portfolio, enabling efficient asset management that optimises the operational dispatch of generation and storage capacity.
F Black start/back-up power: Using on-site battery energy enables power generators to avoid fuel costs and emissions from conventional black-start generators. Furthermore, on-site battery energy makes it possible to quickly energise generating assets or use stored power to avoid costly disruptions and continue normal operations after a system-wide failure or blackout.
Efficient management of generating and storage assets, each with different software and control systems servicing a variety of original equipment manufacturer (OEM) equipment, is challenging. A solution lies in adopting a single OEM-independent comprehensive digital foundation with the ability to integrate many data sources into one system.
Figure 2 . Battery energy management systems benefit multiple stakeholders by maximising performance and profitability.
The use of one platform eliminates data silos, enabling efficient asset management that optimises the operational dispatch of generation and storage capacity to meet load across bulk power systems. This platform eliminates complexity and risk by serving as an integrated source for capturing and placing into context the massive amounts of data generated from embedded control and monitoring applications or third-party software and systems. This translates into greater visibility through a single, unified view of all operations that reduce costs while optimising the financial and energy performance across an entire renewable portfolio. Furthermore, this approach provides a pathway to easily and cost-effectively add innovative software for future applications such as remote operations or data analytics.
Figure 3 Applying a defense-in-depth approach to cybersecurity protects energy storage and renewable generation infrastructure without disrupting operations.
Organisations, such as Burns & McDonnell, understand the benefits of an integrated digital foundation. The company recently completed the construction of three lithium-ion BESS to provide support for the Texas, the US, power grid. Emerson’s automation technology was selected to monitor and control the three energy storage projects, which deliver 60 MWh of capacity. According to a news release issued by Burns & McDonnell, the software
increases visibility into battery operations and simplifies system management, allowing the owner/operator to view, optimise, and modify the controls even years after commissioning if system requirements change.4
Additionally, Mitsubishi Power America’s Advanced Clean Energy Storage hub will leverage an integrated digital automation stack to ensure safe and reliable generation, storage, and transmittal of green energy.5 The first-of-its-kind facility will use a common control and safety platform for the end-to-end process of producing green hydrogen, storing it in salt caverns and dispatching the fuel when needed to the nearby 840 MW Intermountain Power Project (IPP Renewed).
There are a number of digital solutions using advanced software and technologies that enable seamless visualisation and actionable insights.
Predictive analytics is integral to realising the promise of efficient and enlightened energy management, as it enables immediate resolution of impending process or equipment anomalies to avoid downtime or catastrophic damage, and enhances operator situational awareness and decision making. Expert rules, complex modelling, artificial intelligence (AI), and machine learning work together to alert and guide operators to take immediate, specific action, dispatch maintenance personnel with the correct equipment for replacement, or direct the control system to automatically make corrective changes.
Remote, real-time access to operations is more critical than ever with the increase of geographically dispersed and often unmanned renewable and distributed energy resources. With remote
gain faster and more precise responses to critical issues, and proactively address maintenance issues to minimise costs and improve the safety of their teams.
And of course, no discussion about power generation would be complete without mentioning cybersecurity. For energy management systems, it is vital to use a depth-in-defence approach to cybersecurity that protects critical infrastructure without disrupting the controlled processes.
The growing integration of renewables with traditional energy sources is reinventing the power business model and infrastructure. As utilities continue diversifying their energy portfolios, they are looking to energy storage systems to turn intermittent energy into reliable, dispatchable power. Using automation software that provides full operational visibility across all assets enables utilities to stay competitive, keep the public safe, and maintain grid stability.
1. ‘Renewables 2021: Analysis and forecasts to 2026,’ International Energy Agency, (December 2021).
2. ‘Global Energy Storage Market Set to Hit One Terawatt-Hour by 2030,’ BloombergNEF, (November 2021).
3. ‘Hydrogen Energy Storage Market by State (Gas, Liquid, Solid), Technology (Compression, Liquefaction, Material Based), Application (Stationary Power, Transportation), End User (Electric Utilities, Industrial, Commercial), Region – Global Forecast to 2027,’ MarketsandMarkets, (June 2022).
4. ‘Burns & McDonnell Completes Construction of Three West Texas Energy Storage Projects Delivering 60 MWh of Capacity,’ Burns & McDonnell, (January 2022).
5. ‘Mitsubishi Power Selects Emerson Software and Technologies for Advanced
f electric grids are to meet societal needs and phase out carbon by 2050, they will need to take on a diverse approach to energy storage. That is according to a study published last month by MIT researchers, who go on to note that integrating those diverse options will enable
grids to absorb the burgeoning power generated by renewable sources, many of which deliver variable real-time supply.1 The researchers add that incorporating diverse storage options can also benefit grid flexibility and resiliency.
Hydrogen Infrastructure, and Tom Cornell, Senior Vice President for Energy Storage Solutions, combining centralised and decentralised energy storage will help future-proof electric grids.
Energy storage allows for “cost-effective deep decarbonisation of electric power systems that rely heavily on wind and solar generation,” the authors write.1 And it does so without sacrificing system reliability – which is to say, rolling brownouts when the neighbourhood heating,
ventilation, and air conditioning (HVAC) outstrips prevailing supply.
The world is encountering a Cambrian proliferation of energy storage technologies. On one side are large, centralised energy storage facilities, in particular,
green hydrogen hubs which will store energy for days, weeks, or even seasons. On the other are decentralised forms of storage, namely batteries, which are often co-located with renewables or are located closer to the point of use. Combining the two could promise a future grid that is robust, stable, and resilient, especially as wind and solar integration accelerates.
Projects underway in Southern California and central Utah, the US, are already offering a glimpse into how a combination of centralised and decentralised storage will enable the post-carbon grid of the future.
The rise of renewable energy amid growing electricity demand makes it essential to develop energy storage systems with ever greater size, flexibility, and responsiveness. For one, the rise in power generation from wind and solar – two sources whose real-time productivity hinges on weather conditions – greatly amplifies the intermittency challenge for grid operators.
Solar is being put everywhere: behind the meter, in front of the meter, on rooftops, and available flat land. This is
going to have a huge, dramatic effect on the grid, and is also going to drive greater curtailment of solar electricity.
In California, at just 30% renewable integration, there is so much renewables overproduced in the winter and spring months that we’re shutting down these carbon-free resources in massive quantities. Meanwhile, there have been energy shortages in the summer and fall months where people have had to rely on carbon intensive resources to keep the grid from blackout conditions. The solution to this involves shifting carbon-free over-generation to seasons which are typically relying today on carbon intensive resources to keep a stable grid.
In 2020 alone, the California Independent System Operator (CAISO) curtailed 1.5 million MWh of its utility scale solar production, equivalent to 5% of total utility scale solar generation. 2 Operators have added renewables at a furious pace since then – and not just in California. Solar and wind accounted for approximately 90% of new electric generating capacity across the US in 2021 and in 1Q22, 3 and the International Energy Agency (IEA) projects that renewables will account for approximately 95% of the increase in global power capacity through 2026. All told, the IEA expects grids to add 50% more renewable capacity between 2021 – 2026 than it did in 2015 – 2020.
Curtailment of renewables is the most obvious manifestation of the intermittency problem. The most compelling solution to manage intermittency while meeting rising electricity demand is to integrate a variety of storage technologies into the grid – and it is a process that is already underway.
Storage options are often categorised as short duration (for example, batteries) or long duration (such as green hydrogen). Batteries today generally offer 4 – 6 hrs of electricity at maximum draw, although technological advances are likely to increase their duration rapidly in the coming years. By contrast, hydrogen’s ability to continue producing electricity is limited only by the volumes of the storage facilities that hold it.
Duration is a key consideration, but characterising storage options based primarily on that characteristic obscures important, potentially complementary distinctions. Storage technologies differ in other critical ways as well: reaction time, location, infrastructure, investment required, degradation, digital automation capability, security, and more.
FigureIt may be more useful to characterise storage as centralised or decentralised rather than long or short duration. This distinction better captures the range of characteristics available among storage technologies and relevant for grid operators as they weave centralised and decentralised energy sources into cohesive networks that are resilient, flexible, responsive, and valuable.
Hydrogen, for example, can be used as a primary centralised storage option for renewable energy. Global demand for green hydrogen – hydrogen produced using electrolysis powered by renewables – is projected to grow 50% over the next decade.4
Renewable energy is variable; when the sun shines or the wind blows cannot be predicted. At very large penetrations of renewables, this variability moves beyond daily imbalances into seasonal imbalances. When hydrogen is used to help store that energy, it can be shifted over greater time horizons.
With the increase in variable production from renewables, generation will exceed demand more frequently and by greater amounts. Rather than curtailing the excess, power systems can use it to produce hydrogen at virtually no incremental cost, transforming curtailment from underutilised to an opportunity.
Moreover, centralised hydrogen storage can capitalise on existing energy infrastructure to store, use, and transport the gas, helping manage the cost of scaling up. Consider the Advanced Clean Energy Storage hub currently under construction in Delta, Utah, the US.5 This joint venture by Mitsubishi Power and Magnum Development will produce green hydrogen and store it in enormous salt caverns.
The hub is designed to initially use 220 MW of electrolysers that convert renewable energy into 100 tpd of green hydrogen. With capacity to scale up to 100 salt caverns, the initial two-cavern facility will initially store the equivalent of more than 9 million bbl of hydrogen, or approximately 300 GWh of storage capacity, making it the world’s largest single storage site for hydrogen.
Adjacent to the hub is the Intermountain Power Agency’s IPP renewed project, a retiring coal plant that is being replaced with an 840 MW, combined-cycle power plant with two M501JAC gas turbines which will run on a blend of 30% green hydrogen and 70% natural gas starting in 2025 and 100% green hydrogen by 2045.
Centralised storage with hydrogen offers a range of benefits:
F Volume : The quantity of storage possible with hydrogen is unmatched by other options.
F Economies of scale : Centralised hubs’ large size creates efficiencies that can bring down costs. For example, one facility with 100 MW of electrolysers would produce the same amount of hydrogen using one transformer as five facilities at 20 MW apiece, but the latter group would need five transformers. The Advanced Clean Energy Storage hub is expected to help cities in the Western US achieve 100% carbon-free targets at 20% lower cost for the overall system than if they did not have centralised energy storage.
F Long-term viability : Hydrogen stored in salt caverns has minimal losses and does not degrade over time, enabling long duration, seasonal storage.
F Sector coupling : Storing, using, and transporting hydrogen at scale will enable progress in other hard-to-decarbonise
Figure 3 A rendering of salt caverns in the Advanced Clean Energy Storage project. The project is expected to be the world’s largest industrial production and storage facility for green hydrogen. FigureF
industries, such as transportation, cement, and steel. Hydrogen is needed not just to decarbonise power, but to decarbonise the hard-to-abate sectors.
Flexibility: Scaled integration of storage, transport, generation, and transmission systems gives operators the latitude to use hydrogen in ways that deliver the greatest value with the greatest impact.
Solar and wind power are proliferating. 15 US states have 1500 or more solar installations, with Florida (9000), Texas (14 000) and California (more than 35 000) leading the way.3 New solar installations increasingly come with batteries: nearly one in three behind-the-meter solar systems are expected to pair with battery storage by 2025,3 compared to fewer than one in nine in 2021. For their part, utilities have commissioned or announced combined solar and storage projects representing more than 50 GWh of storage capacity.
Bloomberg reports that battery power now makes up 6% of California’s maximum on-peak capacity, or 60 times what it did in 2017.6 Batteries’ contribution to maximum on-peak capacity now exceeds both wind’s and nuclear’s.
Last year was a breakout year for the sector, to prove that on a utility scale basis, battery storage is a viable, resilient, and dependable source of energy.7 If you look at the transmission queues in all regions of the US, they are getting saturated with battery energy storage projects. For example, Texas’ battery installations could rise from approximately 2300 MW to more than 7000 MW by next June, according to Bloomberg.
Mitsubishi Power is also seeing increasing demand for such projects in Latin America, Europe, Southeast Asia, and Japan, and expects the utility scale battery market to double in 2022 and again in 2023.
These decentralised options offer distinct, complementary qualities relative to centralised storage. They have smaller footprints and fewer constraints on their locations, so they can often reside near end users to reduce transmission and infrastructure costs. Moreover, geographic dispersion can bolster resilience by enabling grid operators to overcome problems in a subset of locations.
Batteries offer a reaction time that is measurable in milliseconds. That quality, combined with the ability to locate near end-users, makes them ideal for fast-frequency response. Indeed, Mitsubishi Power has installed several battery systems for just that reason for the Electric Reliability Council of Texas. Alternatively, batteries can be geared for peak applications, time shifting, and energy arbitrage. They can maximise value by managing charging and discharging in increasingly sophisticated ways.
Much as centralised storage offers cross-pollination with heavy industry, decentralised storage benefits from synergies with the electric-vehicle (EV) market. EV batteries are replaced after they lose 20% of their original peak capacity. These batteries can be repurposed for usage on the grid, where modest degradations in peak storage are more manageable. Likewise, automakers’ intense focus on improving battery technology has tripled the rated energy for mass-market EVs in roughly a decade. Those breakthroughs, in turn, stand to benefit utility scale battery users.
FigureAnd grid storage is not constrained by some of the limitations that challenge EV battery technology, particularly the need to minimise weight and size. As a result, grids may be able to employ battery technologies that are not suitable for electric vehicles. This point of distinction may prove valuable if certain components of EV batteries, such as cobalt, become scarce due to product demand or geopolitics.
Decentralised storage with batteries offers a range of benefits:
F Renewables integration: Batteries, due to the smaller size, can be co-located with renewable energy to reduce curtailments and located where the storage is needed.
F Grid resiliency: Able to provide electricity quickly at the point needed, preventing supply disruption or congestion in transmission.
F Fast response: Sub-zero second response time to respond to fluctuations in grid frequency.
F Microgrids: Allows for the islanding or to disconnect from the larger network grid as needed and powering a smaller network using storage reserves. Often in the event of an unplanned outage for critical infrastructure systems.
F Flexibility: Batteries can provide peak shaving to offset energy demand during peak periods and shift energy loads by charging when electricity costs are low and discharging when costs are high.
For example, San Diego Gas & Electric (SDG&E) used to employ a distributed backup system of turbines that ran on simple-cycle gas, quick-start natural gas, or oil. The company has replaced such generation with five battery energy storage systems (BESS), making it a leader in battery usage among US utilities. San Diego’s sunny climate – with 266 sunny days per year,8 30% above the US average –contributes to relatively high levels of solar curtailment. SDG&E now uses this excess electricity to charge its batteries, which it charges and discharges strategically to maximise their value and manage interruptions of up to 4 – 6 hrs. As battery storage proliferates, SDG&E will be able to adapt to a hitch in the distribution or transmission system by switching in real time from one battery to another nearby.
Once energy starts being stored at a massive scale, there is going to be the ability to move power in a lot of different directions.
The integration of centralised and decentralised storage is starting to take shape in California. Battery systems are mushrooming around the state, piggybacking on the rise of solar power and EVs, and utilities such as SDG&E are using curtailed generation to charge a distributed network of battery-energy storage. Meanwhile, the Utah hydrogen
facility is beginning to create longer-term green storage to decarbonise the majority of the Western US.
This evolution will accelerate over time in California and elsewhere as batteries become ubiquitous, green hydrogen production ramps up, and power turbines are converted to run on the zero-carbon fuel that is stored. Such conversions are already underway: Mitsubishi Power, Georgia Power, and the Electric Power Research Institute recently validated blending 20% (by volume) hydrogen fuel with natural gas to power an advanced-class gas turbine in Smyrna, Georgia, the US, at both full and partial load.9 Importantly, they were the first partners to do this on an advanced-class turbine.
The rise in complementary storage systems will present a continuous stream of opportunities for energy arbitrage. With options tailored to the situation, grid operators will be able to store energy to discharge at high-value times that may last seconds, minutes, hours, days, or even seasons.
Hydrogen can store green energy over longer time frames than lithium-ion batteries, which can do that more effectively on shorter time horizons. The two of these technologies together are a perfect match to help more widely integrate renewable energy onto the grid.
Integration remains the biggest challenge. It could well become a decades-long endeavour between public and private partnerships to incorporate this diversity of storage with the transmission infrastructure. But the resulting overhaul could create a cohesive, smoothly functioning grid that can respond to demand in real time, maximise efficiency, and guarantee resilience, especially since system operations pose the most difficult challenge.
Stakeholders throughout the power industry will need to collaborate more vigorously than ever to build a smooth-functioning system.
Ultimately, the resulting grid will look very different than it does today. It will be more resilient, adaptive, and consistent. It will be more reliable and efficient, too. Most importantly, it will be carbon-free.
1. ‘The Future of Energy Storage: An Interdisciplinary MIT Study’, MIT Energy Initiative, (June 2022).
2. ANITI, L., ‘California’s curtailments of solar electricity generation continue to increase’, U.S. Energy Information Administration, (August 2021).
3. ‘Solar Industry Research Data’, Solar Energy Industries Association
4. ‘CEO Coaltion to COP26 Leaders: Hydrogen to Contribute over 20% of Global Carbon Abatement by 2050 – Strong Public-Private Collaboration Required to Make it a Reality’, Hydrogen Council (November 2021).
5. ACES Delta, aces-delta.com
6. BULLARD, N., ‘Batteries Are Already Helping Power Grids Weather a Hotter World’, Bloomberg UK, (July 2022).
7. MURRAY, C., ‘‘Getting ahead of the market’: Mitsubishi Power Americas on Li-ion, long duration and green hydrogen storage’, Energy Storage News, (April 2022).
8. ‘Climate in San Diego, California’, BestPlaces
9. ‘Mitsubishi Power, Georgia Power, EPRI Complete World’s Largest Hydrogen Fuel Blending at Plant McDonough-Atkinson’, Mitsubishi Power Americas, (June 2022).
Alastair Morris, Chief Commercial Officer, Powerstar, UK, considers how behind-the-meter storage can generate new revenue for commerical end users and support the National Grid, exploring why energy storage is so vital for the growth of the renewable energy industry.
n a world where net zero commitments and the other elements of the energy trilemma appear to be increasingly competing agendas, the efficient storage and use of renewables is a critical issue. Exactly how end user companies and organisations can capitalise on opportunities – by supplying energy generated and stored on-site back to the grid – is a vital aspect of both agendas. Efficient energy storage combined with contracts to manage a relationship with the grid can offer new revenue streams to such companies.
Battery energy storage system (BESS) technology is a cornerstone of the changing energy landscape, in part because of its proven flexibility which allows for optimum effectiveness in a range of scenarios and for the efficient use of other technologies. For commercial end users, battery energy storage also offers the possibility of offsetting rising energy costs by generating additional revenue from their assets through engaging with grid balancing services. The ongoing and rapid increase in the amount of inflexible, renewable power generation in the UK has made the grid increasingly unstable, often struggling with either too much or too little electricity generation, fundamentally dependent upon weather conditions. For the UK, in unfavourable weather, the country is still largely reliant on gas generation to make up the shortfall, therefore contributing to the
ongoing energy crisis. Within this context, the National Grid are increasingly reliant on balancing services to manage supply and demand more effectively and, in the long-term, this flexibility will be critical in maintaining a robust grid supplied predominantly, or entirely, by renewables.
In essence, BESS technology works by storing electricity so that it can be used when required or when
most beneficial, depending on the company’s operational demands and strategic priorities. If combined with control software, this operation can be automated, using artificial intelligence (AI) to learn what generation and what usage should be prioritised. Grid scale battery energy storage is well documented and is factored into large scale operations and the wider utilities and power generation markets. However, there is a significant role for the concept in behind-the-meter (BtM) scenarios, where its commercial application can provide an integrated energy management and microgrid solution, one which allows for greater control and flexibility of energy usage. For these commercial end users, there are a range of benefits, including: power resilience through uninterruptible power supply (UPS), cost savings through shifting times when a company uses grid-supplied electricity, and the capability to buffer large loads, such as electric vehicles (EV) charging from the grid, so that they can be more easily and more cheaply connected. However, in terms of the potential of BtM solutions and how they impact on grid supply for the benefit of in front-of-the-meter (FtM) suppliers, the key question to address, to really engage commercial end users with the benefits of investment in BESS, and therefore help to support grid stability, is: how can investment in BESS equate to revenue generation?
While grid scale operators will be thoroughly familiar with the mechanism of selling energy stored in a BESS back to the grid, for smaller scale operations – those that are being defined as commercial end users – this contracting will require the services of a third-party aggregator. Here, there are two contracting options for these smaller scale companies – demand side response (DSR) and firm frequency response (FFR). For FtM, of course, the objectives of each of these options differ – DSR being the process whereby surplus energy is released back to the grid, while FFR is used to rapidly reduce demand or to increase available power in scenarios where a large deviation in system frequency would otherwise result in disruption across the network.
DSR gives the grid the ability to intelligently manage supply and demand, and this is increasingly important as intermittent generation from renewables grows as an overall percentage of power generation. These DSR grid contracts, ‘balancing services’, are facilitated both through end users varying their overall energy demand and, increasingly, via BESS technology. Battery storage allows end users to fulfil DSR contract obligations by turning up, turning down, or offsetting their own demand in real time, to help the grid smooth out peaks and troughs in overall demand. The storage capability of a BESS also means that companies can secure a better price for their surplus power by selling it when it is most in demand. As a critical part of how National Grid balances the grid in real time, FFR contracts can be the most valuable balancing service for end users to provide DSR but can also be the most challenging to engage with. FFR providers must meet
contractually binding response times, requiring companies to be able to respond fully to movements in system frequency within 30 secs. or fewer. FFR uses pre-approved assets to rapidly reduce demand or to increase generation in scenarios where a sudden imbalance, such as a power station unexpectedly shutting down, to keep the frequency of the grid within prescribed limits and prevent the power outages that large deviations in system frequency can cause.
In addition to capability, and how balancing services may fit with a commercial end user’s main business objective, the issue of payment from the National Grid in return for providing FFR is an issue to factor in. These are typically split across two different elements. The availability fee is calculated by the number of hours that a provider makes themselves available, and whether their services are called upon or not, while the nomination fee is an additional payment made available when their asset is utilised. Payments will vary depending upon the framework agreement and the level of agreed capacity, but the instantaneous requirement does make them substantially higher than other forms of DSR. BESS are one of the most capable and appropriate technologies for FFR, and particularly dynamic FFR. Their ability to rapidly draw energy from the grid and to deliver it back, when necessary, makes BESS a powerful asset – both for the end user and for the National Grid. The instantaneous response times available with BESS lends perfectly to the flexibility demanded by grid balancing. For businesses, especially those operating in energy-intensive sectors such as healthcare, high value/high volume manufacturing, and data centres, many continue to struggle in the current energy crisis. DSR services represent a way to offset energy costs by generating new revenue streams, while also helping the National Grid to balance the network and mitigate against supply disruption.
All this said, there needs to be a word of caution. Battery energy storage was originally able to offer a compelling return on investment from grid revenue services alone. However, the current landscape is somewhat different. Changes to schemes and to payments available, coupled with the rapid growth of battery storage as a sector in its own right, indicate that – outside of grid scale projects – revenue from the grid is unlikely to provide sufficient return on investment as a sole justification for investment in BESS. For these end user commercial organisations, new revenue from balancing should, arguably, be viewed as a secondary benefit. Fortunately, there are numerous scenarios where this secondary benefit is proven to be a case for investment. For example, Powerstar were contracted by a global leader in aviation, manufacturers of precision, high-value landing gear and suspension. Energy disruptions to their plant were causing halts in operations, meaning the destruction of eight material blocks per year, each worth more than £150 000. The application of uninterruptible power supply (UPS) as an
innovative capability of BESS has protected the site from any production disruption, giving a payback period of less than two years. This payback period does not factor in their new capability to provide balancing services to the grid. Similarly, for an NHS hospital project in South Yorkshire, UK, the overriding requirement was for security of power supply through UPS. Powerstar specified a new BESS, which incorporated the rapid switching speed and reliability required, but also offered much lower losses, at less than 1% of capacity, than a traditional UPS system. This solution offers cost savings of approximately £225 000 per year, eliminating approximately 190 t of CO 2 e and allows for the generation of approximately £100 000 of additional revenue through sale of energy back to the grid.
For the UK to meet its net zero targets and for wider, global, carbon emission reduction ambitions, an increase in uptake of BESS technology must show a positive impact, particularly if such organisations could become a larger slice of the capacity market. Within the UK, the capacity market works as a buffer to protect against supply disruption as the country’s energy mix becomes more varied and more unpredictable. Using a descending clock format, auctions have been widely criticised. Starting at £75/kW and reducing until a minimum price is reached, the 2019 top-up auction reached £00.77/kW, making it almost impossible for smaller scale companies to compete. Effectively, the capacity market has been subsidising fossil fuel generators – who are often the lowest bidders – to remain on standby in case they are needed by the grid.
One case study from Powerstar that perfectly combines both the net zero agenda, and the cost reduction imperative is a project for The Design Museum, UK, a leading museum devoted to contemporary design in any form. With a core mission to promote sustainable development and design, and to lead by example, a review of their energy strategy found average monthly energy costs rising by approximately 30%. A BESS has allowed the Design Museum to reduce overall energy bills through storing energy to use at peak times, while also generating additional revenue from grid contracts. These greater energy efficiency and new revenue streams reduced the museum’s overall energy costs by 21%, and reduced energy consumption on site by more than 8%, allowing the registered charity to reinvest savings back into exhibits and visitor experience.
Given the above, and to summarise the issues raised here: to achieve net zero targets, the shift to renewables must be continued. While this move creates significant problems for supply and demand and for the stability and security of power generation, a nuanced approach which uses more localised virtual power plants – the networks where BtM energy management strategies and investment in BESS contributes to overarching FtM supply – offers a positive pathway to emission reduction, cost reduction, and supply security, the three arms of the energy trilemma. BtM can and should have a significant, albeit micro, role in a macro approach to energy security and sustainability.
lobally, 65% of greenhouse gas (GHG) emissions are caused by the burning of fossil fuels.1 As the climate crisis intensifies, it is essential that alternative energy sources are searched for. Managing waste contributes to the climate crisis: even in a landfill, organic waste decomposes into methane, a potent GHG, and can leak into the atmosphere. Kore has developed a technology to solve both challenges by converting waste into carbon negative renewable energy, reducing the need for fossil fuels.
The process, known as pyrolysis, begins with wastes such as demolition wood, agricultural residuals, forest thinnings (to reduce wildfire risk), and green waste. These feedstocks are continuously fed to the pyrolyser where the temperature is raised to over 1000˚F in an oxygen-free environment. Under these conditions, the feedstock decomposes into a gas and a solid carbon char. The system operates in a closed-loop – the technology produces zero waste, and the feedstock generates heat to power the process without supplemental energy. Since the feedstock is biogenic (it originated as a plant), these products are called biogas and biocarbon.
After spending over 60 mins. in the Kore reactor, the biogas is sent to a gas conditioning system where it first passes through a hot cyclone to remove fine solids, then is quenched in a venturi
scrubber to reduce the temperature. Finally, the gas is dehumidified before being conveyed into a storage vessel. Condensable gases produced in the venturi scrubber can be recycled to the reactor, used to generate process heat, or upcycled to methanol and acetic acid for offtake.
Biocarbon exits the reactor through dual knife gates to preserve the airtight seal in the reactor. The biocarbon enters a screw conveyor equipped with a cooling sleeve that reduces the temperature to near ambient as it lifts and discharges the biocarbon into a storage and offloading hopper.
Approximately half of the carbon in the feedstock converts to elemental carbon (biocarbon). Biocarbon makes an excellent soil amendment because it retains moisture (less irrigation), retains nutrients (less fertilizer), and improves soil microbial growth, all of which contributes to higher plant yield. Think of biocarbon as a sponge that holds onto water, not only reducing irrigation but increasing drought tolerance. The biocarbon produced by a standard Kore process can reduce irrigation needs by up to 60 million gal./yr. Biocarbon also absorbs fertilizer, allowing the plant to access nutrients when it needs them. This provides two benefits: less fertilizer is applied, and less fertilizer runs off into the environment, causing nutrient pollution.
In addition, because the biocarbon is produced at a high temperature, it is biologically stable – it will not biodegrade to methane (CH 4 ) or carbon dioxide (CO 2 ), and will remain in the soil for centuries. And, because the carbon originated in the atmosphere (biogenic feedstock), the process is carbon negative. Biocarbon also has an energy value comparable to coal, and can be substituted for coal in steel and cement production, both notoriously difficult to decarbonise.
The carbon negative biogas produced has an energy value approximately half that of fossil natural gas. It can replace natural gas to produce heat, power, and/or steam using a client’s existing burner technology. Composed primarily of hydrogen (H 2 ),
carbon monoxide (CO), CH 4 , and CO 2 , the biogas can also be upgraded to carbon negative hydrogen using proven technology such as pressure swing adsorption, or it can be converted into carbon negative renewable natural gas via methanation. Below is a list of typical applications for the energy products derived from Kore’s biogas.
F Heat: The biogas and biocarbon can be used to generate heat for heat intensive processes such as cement manufacturing.
F Power: The biogas can be used to generate power for electric utilities, college campuses, and factories to help sustainably meet electricity needs. Biogas can also generate uninterruptible power for large server farms and data centres to lessen the burden on local grids.
F Steam: The biogas can be converted into steam for applications such as food and beverage production.
F Renewable natural gas (RNG): The biogas can be upgraded to RNG which is molecularly indistinguishable from fossil natural gas. This carbon negative RNG can replace fossil natural gas in many applications, including: compressed natural gas (CNG) vehicles, traditional hydrogen produced by steam methane reforming, and natural gas fired power plants.
F Hydrogen: The biogas can be upgraded to fuel cell quality hydrogen which is 99.999% pure, also known as 5-9s hydrogen. This UltraGreen Hydrogen TM is suitable for fuel cell electric cars, trucks, buses, and trains. Because the carbon intensity of this hydrogen is very low, the credits available through policies such as the low carbon fuel standards (LCFS) in California, the US, and soon other states, are very valuable. Other uses for UltraGreen Hydrogen include warehouse and distribution centre forklifts, steel manufacturing, and ammonia production.
Hydrogen is one of the more interesting energy products that Kore can produce. Hydrogen use is rapidly growing in California, where policy is driving the transportation sector to decarbonise. The state currently has 54 light-duty hydrogen refuelling stations,
with another 117 in development. A standard Kore system can produce up to 1000 kg/d of UltraGreen Hydrogen, enough to fuel over 1400 fuel cell electric cars. This standard design, skid-mounted technology can fit into tight spaces almost anywhere, reducing feedstock and hydrogen transport costs and increasing accessibility to low-cost green vehicle-grade hydrogen. UltraGreen Hydrogen can also be used by warehouse operators to power quick fuelling, zero-emission fuel cell electric forklifts and Class 8 heavy-duty trucks.
The Kore process also complements other waste management technologies such as anaerobic digestion. Anaerobic digestion converts high moisture organic wastes such as food waste, animal manure, and wastewater treatment sludges into biogas containing methane and carbon dioxide. The methane can be separated and processed into RNG. But the anaerobic digestion process is incomplete – approximately 40% of the incoming waste remains as a semi-solid digestate that must be managed, typically by land application. However, the presence of dangerous chemicals and excess nutrients limit the options available for land application of digestate. The Kore process can use this digestate as feedstock, increasing the overall RNG production by 50% or more and eliminating digestate management problems.
The Kore process is a true energy transition technology. The technology can be implemented initially to provide biogas for heat, power, and/or steam generation. Then, a hydrogen separation process can be added to separate UltraGreen Hydrogen from the biogas. The gases left after hydrogen separation are still very energetic, so they can continue to produce heat, power, and/or steam. Hydrogen production can start small and increase as demand grows. Eventually, the facility could convert entirely to hydrogen production, with the tail gas used to meet the process energy demands.
The key to the Kore process is maintaining the feedstock at a very high temperature in the complete absence of oxygen – easier said than done. The Kore system has been mechanically proven to withstand the rigours of continuous, high temperature, 24/7 operation, with an airtight reaction zone. Kore has been successfully operating a commercial demonstration facility for over a year on a site in downtown Los Angeles, the US, owned by Southern California Gas (SoCalGas) to prove the mechanical integrity of the equipment.
“SoCalGas will continue to support companies developing innovative technologies to help achieve
carbon neutrality,” said Neil Navin, Vice President of Clean Energy Innovations for SoCalGas. “The production of carbon-negative RNG and hydrogen could help provide energy security and decarbonise California in our energy transition.”
This demonstration project has been fully permitted in downtown Los Angeles. The South Coast Air Quality Management District (SCAQMD), the air quality regulator for southern California, one of the most restrictive air regulators in the country, issued some of the many permits for the facility. As a show of its support, the SCAQMD is also supporting the project both technically and financially.
The only emissions from the process is flue gas that exits the pyrolyser after indirectly heating the feedstock. These emissions are tightly controlled by the burner design and operation.
The demonstration facility processes 1 tph of feedstock, producing approximately 10 million Btu/hr of renewable energy. Biocarbon production is approximately 20% of the feedstock flow. Because this is a research project, none of the energy products are sold, but instead they must be carefully disposed of. The biogas is completely combusted in a flare, and the biocarbon is removed by a waste management company that uses it as an asphalt additive.
The next steps for the company’s demonstration project is to test a wide variety of feedstocks to collect performance data for commercial applications. Kore will also begin testing hydrogen production using pressure swing adsorption technology. The UltraGreen Hydrogen produced will be available to fleet, retail, and other off-takers in southern California for testing and use.
Kore believes in circular solutions that benefit a wide range of customers and industries. The company’s technology is at the cutting edge of the energy transition and is changing the way people think about waste.
David Richardson, Business Development Manager Global Renewables, Yokogawa, UK, details how to improve the performance operation and profitability of waste-to--energy plants.
waste-to-energy (WtE) plant is a complex process involving numerous interconnecting systems and processes. Unlike the conventional coal and/or gas power producing plants, the hugely varying calorific value of the waste being burned generates considerable
problems for the operators of such plants, with the combustion process being considered somewhat unstable. To prevent plants from tripping, many WtE plants run below their design setpoints, and therefore project returns on investment subsequently suffer. Compounding the problem and contributing further to the process
instability are issues associated with fuel feeding where overfeeding and starvation are both common.
A WtE plant can be split into a number of constituent processes/operations: fuel feeding, combustion control, boiler efficiency, corrosion, and emissions. There is considerable overlap between how these elements relate to each other, and total process understanding is necessary if the performance of either new-build or existing plants is to be firstly addressed and then improved. A simple example can be seen from the relationship between combustion control, flue gas temperatures, and both boiler efficiency and corrosion. Corrosion is inextricably linked to temperatures. Whilst it is easy to clean open pass boiler sections, cleaning of the congested superheater sections of a boiler is less straightforward, and with sticky and corrosive fly ash accumulating on superheater tubing, explosion cleaning is an expensive and necessary operation to be undertaken by many plants. Combustion and temperature control, flue gas temperatures, and open pass boiler section cleaning solutions all impact on the
rate of boiler corrosion. Without a detailed understanding of the interactions of the plant sub systems/processes, the improvement in one area can easily have a detrimental impact elsewhere in the plant.
WtE plants represent a considerable financial investment. Suppliers of such plants feature considerable pricing pressures when bidding to supply new-build plants. These pressures impact upon margins, and so plants will feature the technologies that are necessary to pass plant performance tests. Additional solutions and technologies that could be integrated into the plants and which would maximise plant operation performance and profitability, unfortunately, are often missing. To the owner of a WtE plant, a 5% increase in the amount of waste processed can lead to significant additional earnings. In many cases, and particularly as plants increase in size, such performance improvements can lead to considerable additional revenues. As the price of power also increases within the open market, these annual increases to the revenue stream are repeatable and consistent throughout the lifecycle of the plant. They are not a one-shot single benefit.
It has previously been stated that the WtE plant is a difficult process to control. The varying size, shape, and calorific composition of the waste being processed all contribute to considerable instability to the heart of the process – the combustion. There are many techniques and solutions that have been developed and implemented over the years with varying degrees of both sophistication and success. The conventional methodology for controlling the combustion process involves the traditional three-term PID controller. Whilst this works well for steady-state operational conditions, this type of control struggles to deal with the significant process delays seen by many of the control loops. Some of which, following a change to a particular control action, will fail to register a change in process conditions for prolonged periods of time (sometimes anything from 5 – 15 mins).
Some solutions have implemented very complicated evolutions of the PID controller, others have tried a more conventional approach using techniques from other industries. Model predictive control, fuzzy logic control, adaptive self-tuning controls, and artificial intelligence are all solutions that have been tried and tested to varying degrees of success. In almost all cases, the final solution has been complicated, requires
significant mathematical prowess from the author/designer, and the solution has been a ‘secret’, meaning that only the supplier can make changes/adaptations/modifications. And, of course, such solutions are normally accompanied by a significant financial consideration.
When investigating solutions for the improvement of WtE plant combustion control, truly open solutions should be investigated as a matter of course. A WtE plant is complex, meaning its performance and operation will change during its 20 – 25 year lifecycle. Complex control situations are difficult to understand and even more complex to modify. Simpler is best. Solutions exist within the marketplace where plant process engineers can be trained to implement performance improvement projects and where project/solution implementation and ownership can be retained in-house.
The heart of any power plant is the combustion process and the production of steam. Instability in steam production can be improved typically by approximately 20 – 25%. Improving such steam stability allows setpoints to be raised and will typically see an increase of 5 – 10% in the amount of waste processed, 3 – 5% increase in the amount of both steam and power generated, and significant improvements into the plant emissions levels. Nitrous oxide (NOX) reduction levels of 15 – 20% are readily achievable, and in a number of cases improvements to NOX emissions through the combustion control process have allowed a number of existing plants to retain and renew their operational permits in the light of tightening national emissions levels, without the need to provide complex and costly selective catalytic reduction (SCR) technology. The use of advanced controls to operate existing NOX reduction equipment (SNCR) has also proven to be a considerable success where again temperature is a critical contributing factor to the successful operation of such equipment.
As a conventional WtE plant ages, lifecycle costs become a very serious consideration. A huge area of interest, focus, and attention can be found in the prevention and/or reduction in the levels of boiler performance and corrosion. Waste is often clearly acidic in its nature. Featuring varying levels of chloride content, sticky corrosive fly ash adheres itself to boiler walls and superheater tubing. Over time, this fly ash builds up on the boiler surfaces and heat transfer rates are seriously impacted, boiler efficiency deteriorates, and levels of corrosion accelerate. Furthermore, chlorides, especially zinc chlorides, form low melting eutectic liquids which are extremely corrosive for superheater boiler tubes.
An efficient ‘shower’ or water jet cleaning system can be effectively utilised to clean the open pass sections of the boiler, water jets impact upon the boiler walls, flash off to steam, and break up the fly ash deposits. An added benefit from such a cleaning process is then a reduction in flue gas temperatures and a corresponding reduction in temperature-related corrosion.
Such technology, however, does not work in the congested superheater section of the boiler. Here, the most
widely accepted and practiced solution is the use of explosion cleaning. This is an established technique for cleaning the restrictive areas within a WtE plant boiler, but it is an expensive and time-consuming process.
Following extensive development over a six year period, a patented solution for cleaning the congested superheater section of a boiler has emerged. The results have shown reductions in corrosion within boiler superheater sections in excess of 55%. This patented solution utilises the principle of sulfatation. Sulfur is injected alongside the secondary air of the combustion process. This results in a chemical reaction taking place in which the chlorides within the flue gas are transformed into sulfates. The rate of conversion depends upon the concentrations of sulfur used, and the conversion is designed to take place prior to the sulfates making contact with the boiler tubes. The reaction takes place in the initial part of the boiler, and requires high temperatures and a high local oxygen excess rate. The results are significant, with considerable reductions in both corrosion levels and the necessity and expense for explosion cleaning.
The operation of a WtE plant is carried out by a number of operators and a plant-wide computerised control system. There are a number of manufacturers with experience in having delivered such systems but there is a huge variety with respect to performance improvements to the operation of such plants and the necessary accompanying process knowledge. For new-build plants, this is something the plant builder/EPC contractor is responsible for, but once the plant has met its post-contract performance requirements and the plant builder has moved on to the next project, performance improvements become the responsibility of the plant owner/operator. Performance improvement projects can be carried out by interfacing to almost any plant-wide computerised control system, regardless of manufacturer, and do not require significant additional replacement costs to be a consideration.
Yokogawa Electric has invested considerably in technologies and solutions for improving the performance operation and profitability of both WtE and biomass power plants. Recently, Yokogawa Electric acquired the shares of Dublix Technology, a Danish company with approximately 30 years of expertise in both product and solutions dedicated to the performance improvement of such plants. Yokogawa solutions improve the performance of WtE and biomass plants with solutions dedicated to:
F Increasing the stability of the combustion process.
F Increasing the tonnage of waste processed.
F Increasing both the steam and power production.
F Reducing plant emissions, specifically NOX and CO.
F Improving boiler efficiency.
F Reducing levels of plant corrosion.
aste incineration is the last resort of recycling management and is becoming more and more important. What cannot be reused or recycled is burned. Only little residual quantities should be dumped. The energetic utilisation of waste and residual materials has a long tradition; however, the inhomogeneous fuel involves some challenges. These can be mastered by means of digitalisation.
Waste-fired combined heat and power (CHP) plants, which are a variety of thermal waste treatment plants, currently have high utilisation rates. After all, a waste-fired CHP plant works just like other power plants. The fuel is transported to a furnace where it is burned continuously by means of a combustion grate while air is supplied. The heat drives generators that produce electricity; the waste heat is used in heat grids, and the steam is directly applied for industrial purposes.
In most cases, a higher energetic utilisation rate is only feasible by increasing the throughput. This, however, is only possible by optimising the combustion process. Thus, downtimes can be reduced and the hours of operation can be increased. One technique for this purpose consists in condition-based maintenance, which has been tried and tested owing to digitalisation and artificial intelligence (AI).
A largely trouble-free process of this kind is only possible by means of a process quality monitoring and condition monitoring of the thermal waste treatment plant. For this, all relevant process data has to be captured, processed, and provided to the control system according to the principles of big data and machine learning in real-time. This also means that anomalies can be detected, as well as creeping changes, and they can be reacted to as quickly as possible – also automatedly.
The fuel of waste-fired CHP plants requires pre-processing, such as grading and drying, in order to neutralise some of the inhomogeneity. In spite of this, it is impossible to
achieve a gross calorific value similar to that of oil, gas, wood, or coal. Moreover, external factors such as the weather, quality, load, or contaminants contained in the waste, as well as others, need to be considered.
Here, only a continuous monitoring of the combustion process and regular interventions help to generate the products electricity, heat, and steam continuously and with due quality.
In 2009, STEAG Energy Services implemented a visual and digital monitoring of the combustion process at a waste-fired CHP plant in Spain. The PiT Navigator Waste applied here is a system developed by STEAG on the hardware and software side. It consists of a combination of a video camera and a thermographic camera in a refractory housing. The cameras are oriented from the rear towards the end-most combustion zones, the fire end line.
The data is sent to the process control system, which uses AI methods for the computationally intensive tasks, via a standard interface. At the same time, it is shielded from possible cyber-attacks from the outside. If the process control system has to be replaced, only the interface will be adjusted. The furnace capacity control will remain unchanged, which ensures an immediate and reliable operation.
The user interface of the PiT Navigator Waste enables programming either on a separate monitor or as an additional input screen in the process control system. Thus, all customer requirements for a clear visualisation can be fulfilled.
The benefits consist in:
F
A lower fluctuation of the steam output.
F An improved burnout.
F Decreased emission peaks for carbon monoxide (CO) and nitrous oxide (NOX ) emissions.
Simon Geiger, STEAG Energy Services, Germany, details how digitalisation can make waste incineration more efficient in waste-fired combined heat and power plants.
This, in turn, optimises the furnace capacity control. Owing to neural networks, an advanced process control, and intelligent data processing, the data thus gained enables an advanced steam projection for the following 2 – 5 mins. in each case. This again allows downstream controllers to react to a steam peak before it occurs by means of the actuating variables and to minimise the deviation of the generated steam quantity from the reference value. Thus, the system follows the methods of artificial intelligence (AI). However, it is possible to manually intervene in the process at any time. The procedure described here can also be applied to other important process variables of the plant.
This use of data, which is ground-breaking for waste-fired CHP plants, enables the simultaneous setting of parameters such as steam output and combustion temperature by means of the air quantity, air temperature, or grate speed. All controls are completely adaptive. They are retrained and optimised fully automatically at regular intervals. Grate trimming, air distribution by means of primary air and secondary air fan, as well as other parameters can be manually influenced by the plant operator at any time.
In 2021, an adjustment of the plant in Spain was carried out that mainly affected the hardware and software. The operation has been simplified significantly. Several options are now suggested to the plant operators, depending on the situation; they can still enter information manually.
One problem that can be solved this way is that of wet waste or waste of bad quality, which frequently occurs in rainy Spain. It would impede the combustion process. Owing to weather data, which are imported by AI-based systems as well and influence the control of the plant, it is now possible to detect when bad waste occurs.
The system of the PiT Navigator Waste is subject to continuous development. It has been implemented at other plants all across Europe. One advantage consists in the fact that this can be done during operation.
F Reduction of standard deviation of steam fluctuations by 25% (achieved: 45%).
F Increase in steam quantity by 3% (achieved: 5.9%).
F Reduction of the standard deviation for steam to 3.3 tph (achieved: 1.8 – 1.9 tph; 2021, after the update: 1.2 tph).
F After the update of 2021, the steam reference value of 44 t can be increased by 4 t or 10%.
General benefits owing to the PiT Navigator Waste:
F Stabilisation of the steam output, resulting in 3% higher waste throughput (control deviation for steam smaller than 3%).
F Improved combustion process, resulting in better greenhouse gas (GHG) emissions (40% less CO peaks, 10% less NO X standard deviation).
F Return on investment is achieved in less than one year.
Alongside the PiT Navigator Waste, the early warning system SR::SPC Predictive Analytics developed by STEAG also ensures the early detection of creeping and critical changes by monitoring processes and main components of a waste-fired CHP plant. SR::SPC combines state-of-the-art data analysis with machine learning and AI via big data in real time. From historical, sensor-based data, the system develops digital twins and continuously compares them to the current data. This also allows to immediately detect potential faults and anomalies in the operation or damages
in the plant. This, in turn, enables predictive maintenance and repair of the plant, e.g., during scheduled downtimes or at times with low load.
SR::SPC Predictive Analytics offers the following benefits:
F Transparency owing to processed data and derived key performance indicators (KPIs).
F Early detection of inefficiency and damaged infrastructure.
F Prevention of consequential damage.
F Improved maintenance planning, as well as spare part supply chain.
F Optimisation of the staff deployment.
F Improved availability and efficiency.
F Increasing the availability, and reducing OPEX.
In the case of waste-fired CHP plants in particular, plant components are subject to faster wear and fouling due to the inhomogeneous fuel. This, in turn, leads to downtimes and losses.
Such a system is already in operation at the utility company, MVV, in Mannheim, Germany. Most of the 467 MW electrical power plant output is generated by eight waste-fired CHP plants and four biomass-fired CHP plants. The production processes are fully automated. Several thousand sensors and actuators serve this purpose in each plant, providing approximately 25 000 measured values every 2 secs. since 1991.
Owing to these data, implementing SR::SPC Predictive Analytics was possible and reasonable here as the arising amount of data made an estimation of the parameters regarding both the process and the condition of the plants only by the staff difficult. Therefore, from October 2018 on, STEAG Energy Services implemented this early warning system at the thermal waste treatment plants of MVV at the Mannheim site over a period of six months.
In a first step, the process connections were upgraded to standardised interfaces and moreover, a central Industrial Internet of Things (IIoT) platform was set up.
The sites process control systems were connected to the respective IIoT network segment via an OPC-UA server. Here, each segment has its own linking computer. It transmits the data from the control system to the central long-term data archive via virtual private networks (VPN). Basically, this data can be accessed from each office. The embedded data management system ensures an adequate visualisation by means of which all processes can be monitored. Deviations will result in an automated alarming.
Essential benefits include:
F Compliance with the KPI nomenclature.
F Activation of all outlier filters.
F Creation of suitable selection filters.
F Existing load indicator.
F Input neurons of all relevant values available.
F Selection of a suitable training period.
F The number of the hidden neurons is consistent with the complexity.
F A match of at least 80% for the test data.
F Creation of a realistic documentation.
The following specific implementations were performed for the MVV plant:
F The superheaters of the steam generator were arranged as contact heating surfaces in the flue
Figure 2 HMI camera page (PiT indicator rear).gas stream. This involves a reduction of the flow cross-section due to fouling, which, in turn, needs to be regularly removed by means of explosive cleaning. The alarm values of the plant are adjusted in such a way that this is reported one week in advance.
F KPIs also monitor the bearing vibrations of the ID fan blower, enabling the detection of a higher flue gas humidity and dust load, which can lead to deposit build-up on the rotor of the blower.
F A turbo set of a turbine was analysed with a total of 170 individual values. In doing so, abnormalities of
16 measured values were detected. Their evaluation allowed to carry out a necessary maintenance or complete overhaul early on.
It was proven that AI-based methods and advanced algorithms, such as the deep learning auto-encoder, facilitate the physical modelling for predictive maintenance considerably.
“MVV has evaluated STEAG‘s predictive analytics IT solution, has proven its economic added value, and rolled it out feet-wide. We are delighted with the tool and the co-operation with the team of STEAG,” explained Sascha Schmitt, Planning Engineer for Technology, Engineering, and Digitalisation at MVV Umwelt GmbH in Munich, Germany.
Digitalisation allows the highly complex process of waste incineration and the related production of electricity, heat, and steam to proceed in a more continuous way. A combination of a permanent monitoring of the furnace and the automated control of the combustion process helps in doing so. This is based on the integration of data regarding the gross calorific value and the other properties and condition of the waste delivered and to be burned. This data enables a predictive adjustment of the combustion process. This, in turn, ensures a largely trouble-free operation of a waste-fired CHP plant and also allows for its maintenance, because the data regarding wear is collected automatedly this way as well. This relieves the operation and maintenance engineers as they are notified by the early warning system and can launch a highly efficient maintenance strategy. All this results in an increased plant availability, reduced downtimes, a higher throughput, and the desired longer productive operating times.
Sam Leighton, Managing Director, Bombora Wave Energy, UK, details how harnessing wave energy could aid the world’s transition to a net zero future, highlighting technologies such as wave energy converters and hybrid wind and wave systems.
ounded in Perth, Western Australia, in 2012, Bombora Wave Energy has developed a solution to harness the power of ocean waves. Now on the cusp of delivering a powerful wave energy converter (WEC) – through its flagship 1.5 MW Pembrokeshire demonstration project, Wales – the firm’s utility scale solution is helping to accelerate the energy transition on route to a net zero future.
The word ‘bombora’ comes from the Australian Aboriginal language, meaning an area of large sea waves breaking over a shallow reef some distance from the shore. Now operating from headquarters in Pembrokeshire, it remains a symbolic masthead illustrating the company’s proud heritage and connection to the Noongar people as traditional custodians of the land on which it was founded.
Following a decade of intense research and development, Bombora is now ready to harness one of the world’s largest untapped energy sources – ocean waves –which contain approximately 2 TW of energy, enough to satisfy today’s entire global electricity demand.
Through its novel ‘mWave’ technology, Bombora will combat two key challenges which have hampered wave energy development in the past – the cost of energy production and the ability to withstand mighty ocean forces. Crucially, it has also been designed to capture wave energy at utility scale, producing consistent and cost-competitive renewable energy for commercial power generation.
The mWave structure comprises of a series of air-inflated concave cell modules, fitted with flexible rubber membranes, mounted onto structures below the ocean surface. The cell modules are arranged at an angle to the incoming waves to maximise energy capture.
As waves pass overhead, air is squeezed out of each cell module in sequence, passing through one-way valves and into a duct system fitted with an axial turbine before being recirculated to refill each of the cell modules once the wave has passed. The flexible membrane is made from industrial grade rubber, which is renowned for its reliability and longevity following long-standing use across a range of other marine applications.
Containing no external parts, aside from the flexible and robust rubber membrane, mWave extracts power simply without the need for complex mechanical systems.
The replaceable modular design also helps streamline maintenance processes, minimising downtime and maximising power output. In addition, mWave’s cell modules have a unique ability to shut down in extreme storm events, helping limit design loads and reduce capital costs while improving the all-important cost of electricity.
In terms of site location, the cell modules can be configured to suit either fixed-bed nearshore foundation systems or floating offshore ocean environments.
In July 2022, Bombora entered the final test and assembly phase of its Pembrokeshire Demonstration Project, as it prepared to validate its WEC.
The £20 million initiative has been financially supported by the European Regional Development Fund (ERDF) via the Welsh government. It will catapult the region to the forefront of the global wave energy race, with plans to establish a sustainable industry, engaging local supply chain partners, and creating highly skilled employment opportunities.
Rigorous testing is currently being carried out on key sub-systems as the project progresses at pace, with the
specialist mWave cell membranes undergoing extreme inflation and deflation cycles. This is the culmination of a full-system testing process involving detailed instrumentation, operation of the valve systems, and powering of the power take-off (PTO) module. Throughout the process, Bombora has applied acute attention to detail, pushing its technology way beyond the expected operational limits to ensure high performance levels, robustness, and durability.
In the coming months, the final assembly phase will begin quayside in Pembroke Dock before loadout to the operational site at East Pickard Bay, Wales, where the mWave technology will be validated in the open ocean, advancing it to technology readiness level (TRL) 7/8. The final 1.5 MW mWave device will weigh in at 900 t, with structural dimensions of 75 m long, 15 m wide, and 6 m high.
Once operational, the Pembrokeshire demonstration project will provide Bombora with a blueprint for its future multi-megawatt wave energy projects, proving both
the reliability and competitiveness of its novel mWave technology. The project test plan involves operating mWave for a period of 6 – 12 months, after which it will be removed. While in situ, it will deliver key optimisation data to inform Bombora’s other initiatives, including the InSPIRE project, which is under development with global EPC contractor, TechnipFMC.
The InSPIRE project aims to unlock the enormous potential of hybrid wind and wave arrays, which are set to play a significant role in the future energy transition by combining complementary power profiles to deliver a more consistent and stable clean energy supply to the grid. Adding to the InSPIRE project, Bombora has worked with the offshore renewable energy (ORE) Catapult’s Marine Energy Centre of Excellence (MEECE) on the techno-economic appraisal of the mWave technology within co-located and integrated offshore renewable environments, providing more evidence to support future development.
A separate strategic partnership with Japan’s Mitsui O.S.K. Lines (MOL) is focusing on identifying and delivering wave energy projects across Asia, with funding already secured to conduct a feasibility study in Mauritius for the deployment of a utility scale wave farm leading to a 30+ MW project.
Transitioning to a net zero world is one of the greatest challenges humankind has faced, requiring a complete transformation of how people produce, consume, and move around. The energy sector ultimately holds the key to averting the worst effects of climate change, currently responsible for producing approximately three-quarters of greenhouse gas (GHG) emissions.
A growing coalition of countries, cities, businesses, and other institutions are pledging to reach net zero emissions. More than 70 countries, including the biggest polluters – China, the US, and the EU – have set a net zero target, covering approximately 76% of global emissions. More than 1000 cities, educational institutions, and over 400 financial institutions have joined the ‘Race to Zero’, pledging to take rigorous, immediate action to half global emissions by 2030.
However, commitments made by governments to date fall far short of what is required. Current national climate plans – for all 193 parties to the Paris Agreement taken together – would lead to a sizeable increase of approximately 14% in global GHG emissions by 2030, compared to 2010 levels.
Getting to net zero requires all governments – first and foremost the biggest emitters – to significantly strengthen their nationally determined contributions (NDCs) and take bold, immediate steps towards reducing emissions now. The Glasgow Climate Pact is calling on all countries to revisit and strengthen the 2030 targets in their NDCs by the end of 2022 to align with the Paris Agreement temperature goal.
United Nations Secretary-General, Antonio Guterres, is also calling for further commitments at the forthcoming COP27 climate conference in Egypt, in November 2022, to deliver a global reduction of emissions by 45% by 2030 in order to reach net zero emissions by mid-century.
Figure 2 mWave features a series of air-inflated rubber membrane covered concave cells mounted to a structure below the ocean’s surface, creating an air-filled volume. To maximise energy capture, the structure is arranged at an angle to the incoming waves. As waves pass overhead, air is pushed out of each cell through a series of valves into a one-way air-duct. The cells are refilled once the wave has passed. The flexible membrane is made from industrial grade rubber. The longstanding use of similar materials in a range of marine applications has demonstrated the material’s reliability and longevity.
It is now possible to look to the oceans with hope and optimism on the race to net zero. The International Energy Agency (IEA) believes the sea offers a vast, untapped natural energy resource which will deliver 10% of the world’s electricity needs by 2050, equating to approximately £75 billion annual CAPEX investment into the market.
Up until now, the adoption of wave energy has been slow due to poor survivability of past wave technologies and a relatively high projected levelised cost of energy (LCOE) compared with other renewables. However, a new era has arrived for ocean energy, and Bombora’s pre-eminent technology has been carefully designed to address the survivability challenge, and is projected to be cost-competitive with other marine renewables and fossil fuel energy options in the near-term.
When effectively harnessed, wave energy has the potential to provide continuous power 24-hours a day, with much higher predictability than wind and solar. As well as increasing power output from seabed lease areas through combined wind and wave arrays, it also diversifies the renewable energy supply, reducing the need for storage whilst minimising any impact on the environment.
According to the U.S. Energy Information Administration, the waves around the US coasts could provide 66% of the country’s electricity. And as Bombora now prepares to deliver a powerful WEC in the UK, many other countries around the world are ramping up their interest and support in wave energy projects – including Australia, China, Denmark, Italy, Korea, Portugal, and Spain – amounting to a tidal wave of fresh interest in the sector, and a clear vote of confidence in its potential contribution to the future clean energy mix.
With every stakeholder attached to the energy value chain seemingly seeking accelerated transitions to a net zero carbon future, energy suppliers and consumers around the world face unprecedented uncertainty and opportunity.
Decarbonisation of traditional liquid and gaseous fuels will continue to advance, but the initial ‘default’ response to decarbonisation is focused on green electrification solutions. This has created an insatiable demand for variable renewable energy (VRE) solutions, which require short-, medium- and long-term energy storage to maintain system stability and energy security when the sun does not shine, and the wind does not blow. The demand for energy storage is accentuated by the planned accelerated retirement of base load thermal plants.
This article explores the case for pumped storage hydro to meet the medium- to long-term storage system and market need, investigating its role in the generation mix and the benefits with regards to system security. It also explores the challenges governments and the private sector will need to navigate to realise pumped storage’s value stream in a net zero carbon future energy mix.
Why we need pumped storage hydro Thermal baseload power plants were historically constructed and operated to provide dispatchable power (when it was needed) at the lowest per unit cost
outlines the need for storage technologies in the energy specifically on pumped storage hydro.
of energy. However, the accelerated adoption of VRE has significantly reduced the cost of solar photovoltaic (PV) and wind power, making both technologies cheaper than the short-run marginal cost of baseload coal. Pumped storage hydro can play a significant role in this transition. Paired with VRE, it can now compete on cost with new-build baseload power plants (such as supercritical coal) and provide a decarbonised and secure energy system solution for consumers.
Pumped storage hydropower is not new – it is a tried and trusted solution dating back more than 100 years. Reversible hydropower turbines pump water from a lower reservoir to an upper reservoir during periods of low energy demand (when energy prices are cheap), releasing it again to generate electricity when demand rises. Colloquially, this is referred to as a ‘water battery’.
Markets and power systems globally are currently adopting battery energy storage systems (BESS) at unprecedented rates. But, compared with pumped hydro storage solutions, BESS only solves VRE intermittency issues for relatively short durations. By contrast, pumped storage hydropower is currently the largest-capacity form of grid energy storage that is proven and available. The International Hydropower Association estimates that there are more than 130 pumped storage hydropower plants globally, which can store up to 9 TWh of electricity.
Originally, the economic driver for pumped storage was ‘energy arbitrage’ – storing energy during cheaper off-peak periods for use during peak periods, when prices are high. In a renewable energy system, pumped storage hydro can play additional roles such as regulating system voltage and responding to the intermittency of renewable energy sources (maintaining frequency) by providing dependable, flexible, fast-responding generating capacity to help stabilise low-carbon grids.
The business case for pumped storage hydro is strong. It is currently the only proven economic solution for bulk energy storage at utility scale. Further, the lifespan of pumped storage hydro is often several times longer than most BESS. The Kidston pumped storage hydro project under construction in Queensland, Australia, for example, has a projected lifespan of at least 80 years. When compared to BESS, many cycles of battery deployment would be required to come close to this duration.
Hydro storage facilities are also a generator of inertia. Power networks need inertia to ensure system security. The accelerated retirement of thermal power plants reduces system inertia, thus increasing the risk of system events, including blackouts. Pumped storage hydro provides a proven physical inertia to provide resistance to system frequency changes triggered by system generation and demand mismatches.
It also offers better security or reliability of supply. Supplies of coal, gas, and liquid fuels are often sensitive to geopolitical risks, whereas pumped storage is a dispatchable renewable energy source that is independent from the impacts of conventional fuel supply chain risks and market pricing.
Pumped storage hydro is also considered ‘off-river’ – utilising or recycling essentially the same allocation of water – and means it is not susceptible to the water allocation and supply risks caused by climate change or other geopolitical risks that can affect conventional run of river hydro projects.
Many existing hydro sites are already suitable for use as pumped storage hydro sites with minimal adjustment. The Australia National University (ANU) in its Global Pumped Hydro Energy Storage (PHES) Atlas estimates that there are 616 000 existing facilities with feasible potential to be turned into pumped storage hydro sites, with a storage potential of 23 million GWh.
The wider societal benefits should also be recognised. Renewable technologies have the potential to promote economic development through job creation in construction, maintenance and operations, industry and supply chains, and indirectly in local commerce. For example,
Figurethe Chaglla hydropower dam Mott MacDonald helped work on in Peru saw the creation of 12 500 direct jobs and 10 000 indirect jobs and improved road access for rural communities. Pumped storage hydro backs up these renewable technologies while contributing itself to local economic development.
Pumped storage hydro solutions play a critical part in enabling a secure and cost-effective transition to a decarbonised energy future and helping governments and businesses achieve their net zero goals. Planning and incentivisation is required, and the pace of both needs to accelerate. Governments should start by incentivising and supporting the development of the industry and projects locally through grants, contracts for difference, and other support mechanisms.
This would help overcome some of the barriers to progress. For instance, much of the lifetime cost of pumped storage hydro is incurred during initial construction. Historically, governments or publicly owned organisations invested in pumped storage hydro because they were able to consider the whole-life benefits beyond the high initial CAPEX. In a market with high levels of energy privatisation and without long-term revenue certainty, investors are unwilling to invest in pumped storage hydro.
High upfront costs were initially also associated with wind and solar PV technologies, but these have successfully been implemented into energy markets, including private residences and small businesses, through policy interventions to support deployment. Without similar support for long-duration storage, it will become steadily more difficult to maintain grid reliability.
Revenue generation from pumped storage differs from solar and wind, however. Pumped hydro is always consistent in its output if there is water in the reservoir ready to pump.
More work needs to be done to assess the revenue of pumped hydro sites to encourage investment, and tools such as the International Hydropower Association’s (ISA) sustainability tools can be utilised as a regulatory aid to provide the assurances that many investors need.
There are also several government-supported revenue streams that other energy generation technologies can take advantage of that are not open to pumped hydro. In many markets, long storage solutions that are charged by renewable sources are treated the same as those charged
by traditional carbon-generating infrastructure. This should be re-evaluated, and governments can and should encourage change through financial interventions.
Policymakers should also assess the long-term storage needs of their future power system in the first instance, so that the opportunity to build more efficient options –including new hydro storage facilities or adapting existing hydro facilities into pumped storage hydro sites – is not lost. These policymakers have the power to support a technology that will prop up their markets and provide security for generations to come. If green recovery and ‘build back better’ initiatives are to be properly supported, pumped storage hydro should be a vital part of global grids, strengthening resilience in energy markets and meeting enshrined net zero targets. Without the necessary support, governments will open their markets up to the vulnerabilities associated with energy transition.
Figure 4 . At the Kidston site, Australia, surplus energy will be stored using pumped hydropower, which can respond rapidly to meet spikes in demand or to cover short dips in solar or wind power output. Figure 3 . 50 MW solar project aerial view. Source: Genex Power.Chris Poynter, President, ABB System Drives, Switzerland, emphasises how the entire value chain must be decarbonised in order to reach the world’s net zero goals, discussing how these alternatives need to be cost-effective, cleaner, and more sustainable in order to be viable solutions.
he journey to net zero carbon emissions is one of the most complex, ambitious projects in human history. There is no one technical solution that will break the world’s reliance on fossil fuels. Instead, every link of the value chain must be reviewed.
Only a comprehensive, collaborative approach will enable the world to achieve its goals. For instance, electrification within one industry is an excellent step forward, but if the power is still coming from carbon-intensive sources such as coal, the impact will be limited. Instead, electrification must be paired with low- or zero-carbon electricity generation. The same is true across every industry – a narrow focus is not enough.
Simultaneously, the changes must be financially viable. In a competitive market, expensive changes will see limited adoption, but those that create commercial value while also supporting sustainability objectives will be widely embraced.
A clean start to the value chain: renewable electricity Today, almost all industrial processes require electricity, so electricity generation is at the start of most value chains. Historically, electricity generation has involved extremely high levels of carbon emissions, such as from burning coal or natural gas. For electrification to be sustainable, electricity generation processes must reduce carbon emissions significantly.
Fortunately, renewable solutions for electricity generation – such as solar, hydroelectric, and onshore and offshore wind – are taking over. They already account for a significant proportion of
total power generation, and their global market share continues to increase every year.
Despite this, few countries have fully phased out carbon-intensive power sources so far. This is in part because renewable power output can fluctuate depending on the weather or other unpredictable conditions. Fluctuation can result in grid instability, so operators use predictable fossil fuel plants to ensure a consistent supply of electricity.
These high-emission facilities will need to be phased out in the decades ahead to reach net zero. Even with the contribution of technologies such as batteries, pumped storage, and other energy storage options, as well as grid stability technology such as synchronous condensers, maintaining a stable, predictable energy supply will be a complicated balancing act.
To overcome these challenges, researchers are already conducting important grid stability experiments. A partnership between ABB and the Fraunhofer Institute for Wind Energy Systems (IWES), for instance, is currently constructing the world’s largest mobile grid simulator. It will begin operating in 2023, enabling researchers to reliably test the performance of new equipment, so that they may better understand the potential issues involved in shifting to renewable wind energy and how to address them.
Further along the value chain, there is also potential for decarbonisation. Many industrial processes still rely on carbon-intensive fossil-fuelled equipment. In many cases, this equipment can easily be replaced with electric alternatives. When powered by electricity from renewable sources, this greatly reduces the carbon emissions associated with a given process. In addition to its sustainability benefits, electrification is often better in terms of overall efficiency, reliability, and safety.
The operators of the Vafos pulp plant in Kragerø, Norway, for example, cut emissions significantly through electrification. The plant produces unbleached pulp for cardboard production, and uses an energy-intensive drying process that involved burning oil.
However, the plant has since switched to electric air heaters. These heaters use a significant amount of power and are paired with ABB’s specialised power controllers to ensure they run as safely, precisely, and efficiently as possible, minimising their impact on the grid. Since 98% of Norway’s electrical production comes from renewable sources, this means that the plant has eliminated 14 000 tpy of carbon dioxide (CO 2) emissions. This is equivalent to removing approximately 7000 combustion-engine cars from the roads, and represents one of the community’s
contributions to reaching net zero. The facility also benefits from the fact that it no longer needs complex infrastructure for storing and transporting oil.
Many electric options are drop-in replacements, but in some cases, a clean-sheet design delivers better results. This was the case in the Danish port of Esbjerg, which wanted to electrify the heating of homes and businesses. ABB delivered integrated electrical infrastructure for a seawater heat pump.
The electric solution relies on a system of electric motors, drives, and other technology to deliver carbon-free heat to over 100 000 people. In Denmark, over 67% of the grid’s electricity comes from renewable sources, meaning that Esbjerg’s electrification project has significantly reduced carbon emissions.
In addition to electrification and sustainable electricity generation, industries must also become more energy efficient. The International Energy Agency (IEA) calls energy efficiency the “first fuel” when it comes to reaching net zero.
From pumps and compressors to fans, conveyors, mixers, and even robots, there are few facilities that function without electric motors. In fact, electric motors
Figurealone are responsible for 45% of all global electricity consumption.
Adopting more advanced motors further contributes to energy efficiency. Motor efficiency in many countries is rated by the International Efficiency (IE) standard, with IE5 motors currently denoting the best in class. Each increase in IE class, such as from IE4 to IE5, represents a 20% decrease in losses. Despite this, many facilities still use older, less efficient motors that were installed before these regulations came into effect. Switching these motors to more modern models, as well as pairing them with drives, would significantly reduce their energy use.
Motors that can deliver the same output while using less electricity – the goal of efficiency –reduce both operating costs and emissions. Plus, by replacing existing electric motors with modern models equipped with a variable speed drive (VSD), operators can further cut the operating expenses associated with their motors by approximately a quarter.
This is, in part, because VSDs match the motor’s speed and torque to the requirements of the task. Without a drive, motors always run at full speed. Operators control the speed by opposing it mechanically – much like applying the brakes in a car while keeping the other foot on the accelerator. By comparison, a VSD enables precise speed control.
The result of adopting a drive is electricity savings, because any time the motor is not running at full speed, it is using less power. This is particularly significant due to the non-linear relationship between speed and power consumption: a motor running at half speed uses just one-eighth the electricity of one running at full speed. Despite their energy saving potential, just 23% of all industrial motors are currently paired with a drive.
Data-driven digital tools and services also contribute to efficiency. For example, digitally connected motors and drives within equipment can transmit data on variables such as temperature and vibration directly to operators in real time, helping them to identify areas where energy is going to waste.
These same technologies also provide maintenance benefits by automatically alerting operators to risks and prompting them to take proactive action. This helps facilities to avoid costly downtime – and in some cases, maintenance can be performed remotely, saving labour and logistics costs.
In addition to the many steps that can be taken today, there are also technologies in earlier phases of development that will likely contribute to reaching net zero.
One promising innovation is the use of green hydrogen as a low- or zero-carbon fuel. Hydrogen is already in use in existing industrial applications, and there is a real ongoing effort to expand its industrial, infrastructure,
and transportation applications. As the technology progresses, successfully rolling out hydrogen will depend on creating a new infrastructure for production, storage, distribution, and, ultimately, end use.
An example of a pioneer in the green hydrogen industry is Plug Power Inc., a company which develops hydrogen fuel cell systems that replace conventional batteries in vehicles and equipment. The company has recently announced plans to electrify two new hydrogen plants using an Electrical House (eHouse) solution from ABB. These plants will produce a combined 60 tpd of green hydrogen, replacing 170 t of fossil fuels in the logistics and transportation sectors. The pre-tested and prefabricated eHouse substation enables the careful management of the full electrical system, bringing down costs and ensuring reliability.
Carbon capture, utilisation, and storage is another development that could contribute to decarbonisation. Capture and storage technology continues to advance at a rapid pace, with recent breakthroughs in hydrocarbon recovery and direct-air CO 2 capture. If carbon can be safely captured, it can either be sequestered or current technology can be used to put it to use as alternative fuels or building materials.
Today, fossil fuels still form the backbone of the global industrial economy. Replacing this resource with cleaner, more sustainable, and cost-effective alternatives will undoubtedly be a challenge. However, by reviewing the entire value chain and acting collectively, significant strides can be taken towards achieving net zero.
In addition to a big picture approach, this will require collaboration between individuals, businesses, industries, and governments. There is no single action that will resolve the climate crisis – only a combination of many ambitious activities by organisations in many industries will deliver the results that are needed.
Figure 3 Adding variable speed drives to motors plays a key role in boosting industrial energy efficiency.Independent power producer (IPP) Kenlov Renewable Energy has confirmed the signing of its first ‘cornerstone’ power purchase agreement (PPA) as it progresses its 1.5 GW renewable energy development pipeline in the US.
The PPA covers 60% – or 235 MWdc – of the output of the 400 MW Tierra Bonita solar PV project in Texas, the US, for a period of 20 years. The offtaker is CPS Energy (Moody’s Aa2), the largest municipal electric utility in the US, which supplies 900 000 residents of nearby San Antonio, and the agreement will ensure a steady long-term supply of clean energy to the city.
It also constitutes a further milestone for Tierra Bonita – a 2200-acre site, co-developed by Kenlov and Ashtrom, which has already secured land rights and grid connection approval. Following the successful signing of this landmark PPA, the project is expected to enter construction during 2Q23, with the start of commercial operation anticipated at the end of 2024.
The remaining 165 MWdc output of the project and its green certificates will be sold on a merchant basis in the open electricity market in Texas.
For Kenlov, this news represents a further step forward as the business progresses its substantial pipeline of solar and wind projects in the US.
erentica Renewables has announced the launch of a renewable energy platform in India. This green energy company will focus on providing round-the-clock carbon-free power to large consumers of energy in India. It will specialise in providing end-to-end green solutions to the consumers, including connectivity to the appropriate transmission network. Serentica plans to install approximately 1500 MW of solar and wind power across multiple sites in the states of the Karnataka, Rajasthan, and Maharashtra, India, where the company has already acquired connectivity approvals. A large part of this capacity will be used to deliver close to 600 MW of round-the-clock clean energy to various entities of the Vedanta Group. The capacity will be commissioned in 24 months, subject to statutory approvals. Serentica will also participate selectively in government tenders subject to the project having synergies with its overall focus of providing direct green energy solutions to commercial and industrial customers.
In the medium-term, Serentica aims to install 5000 MW of carbon-free generation capacity coupled with different storage technologies. Eventually, it aims to supply over 15 billion units of clean energy annually and displace 20 million t of CO2 emissions.
H Global Sustainable Energy Opportunities (GSEO) has agreed to acquire three solar photovoltaic (PV) sites in Australia for £21.2 million as part of the existing £50 million commitment to its Australian solar PV and storage investment programme (the ‘Australian Energy Transition Programme’).
The Australian Energy Transition Programme was originally announced in August 2021 with operating partner Birdwood Energy. Phase I, announced in late 2021, consisted of the acquisition of two operating solar PV sites with a combined capacity of 17 MW. Phase II, being the construction of co-located battery energy storage systems (BESS) has already commenced on one of the two Phase I sites. The addition of BESS will allow greater operationality to capture positive power price movements, prevent over-exposure to negative power prices, and allow them to earn revenues from providing frequency response services to the local grid.
Phase III of the Australian Energy Transition Programme comprises an initial three new solar PV sites of 5 MW each, located in New South Wales, Australia. The construction of these three sites is expected to begin 3Q22, with completion and commissioning expected 2Q23. The company retains exclusively over a further two sites of 5 MW each under Phase III.
As part of a potential Phase IV of the Australian Energy Transition Programme, the company is also evaluating, subject to further due diligence, the ability to construct BESS alongside the Phase III assets. All solar PV generation assets are expected to combine merchant sales and long-term corporate PPA revenues as well as large scale generation certificates. In addition, investors are expected to benefit from revenues stemming from profitable energy arbitrage opportunities and frequency services revenues.
Global energy storage platform provider, Powin LLC, has announced the acquisition of EKS Energy, a leading provider of advanced power electronics and energy management solutions for energy storage and renewables. This transaction ensures new and existing Powin customers will receive enhanced product and technology offerings, enabling Powin to deliver a fully integrated grid scale energy storage experience with supply chain security.
As the world transitions to cleaner energy sources, global demand for energy storage will continue to surge to an estimated market of US$49 billion by 2028, according to Bloomberg New Energy Finance. To better serve this fast-growing market, this acquisition expands Powin’s offering to include the EKS Power Conversion System and Power Plant Controller, enabling Powin to deliver a complete AC energy storage platform capable of advanced control functionality to support microgrid applications while interfacing with multiple generation assets.
Other new products include PV inverters, DC-to-DC converters, and multi-port inverters, which are used to maximise performance and profits for utility scale solar-plus-energy-storage projects.
wedish energy company, Ellevio, is expanding its scope to help industries and businesses become fossil-free through electrification. Its first project includes Alfen’s 10 MW modular energy storage system, TheBattery ElementsTM, which enables optimal use of renewable energy and stability in the power grid.
Sweden is one of the first countries in the world to adopt a net zero emissions target, which it intends to achieve by 2045. Sweden expects to more than double its electricity consumption over the next 25 years, while using wind power as its main source of new electricity generation. Depending on how much wind there is, the amount of electricity fed into the grid will vary. If the balance is disturbed, there is a risk of disruptions in the electricity system that can be both costly and lead to dangerous grid outages.
Alfen’s TheBattery Elements can help balance these disruptions. It is an industrial and modular energy storage system equipped with fast frequency reserve control technology that can handle the fluctuations (low inertia situations) in the Nordic grid using synthetic inertia. It requires very fast response times (<0.7 secs.) which are also supported by Alfen’s in-house developed software platform. TheBattery system can also be used for other grid services and/or energy trading.
Conrad Energy has recently secured planning consent for a 50 MW 2 hr battery energy storage system (BESS) in North Petherton, Somerset, UK. The approval demonstrates Conrad Energy’s commitment to enabling the UK’s ambitious net zero plans and reflects their continuing investment in energy storage.
The project will use local topography and innovative design to conceal the scheme within the landscape. Additional planting
and landscaping will boost the local habitat with an estimated biodiversity net gain score of 22.3% and 100% for hedgerow units, enhancing the local landscape and providing shelter for birds, animals, and insects.
This announcement comes as Conrad Energy’s 15 MW 4 hr BESS in Plympton, Devon, the UK, recently achieved planning approval, as well as the planning approval for the company’s first strategic solar development at Larport, Herefordshire, the UK, in May.
Solar & Storage Live 2022
Floating Offshore Wind
Global Hydrogen Conference 2022
Pattern Energy Group LP and its affiliate in Japan, Green Power Investment Corp., have completed financing and begun full construction of its 112 MW Ishikari offshore wind project, located approximately 3 km from the shore of the Ishikaro Bay in Hokkaido, Japan. Ishikari Wind will feature a battery storage component with 100 MW x 180 MWh of capacity.
The Ishikari offshore wind project and accompanying battery storage component is expected to reach commercial operation in December 2023. The project has a 20-year power purchase agreement with Hokkaido Electric Power Network, Inc. for 100% of the power output.
Ishikari offshore wind will utilise 14 Siemens Gamesa 8 MW wind turbines, which are built specifically for offshore use. The SG 8.0-167 DD offshore turbine is designed to meet local codes and standards regarding typhoons, seismic activities, 50 Hz operation, as well as operation in high and low ambient temperatures. The turbines and its supporting structure (pile foundation, jacket, and tower) received ClassNK certification, confirming it meets the stringent technical standards required by the Japanese government to approve construction.
With the inauguration of the Dunowo project, ENERTRAG has commissioned one of the largest wind projects in Poland and the largest ENERTRAG project to date.
The Dunowo wind project is located near the city of Koszalin in northwestern Poland and was successfully commissioned in the course of this year. The official opening ceremony followed, which was celebrated with representatives of local institutions, project partners, land owners, grid operators, and investors.
The two sub-projects (Dargikowo and Karlino), with a total capacity of 186 MW, will generate approximately 600 000 MWh/yr of clean energy. The Dargikowo wind field includes 43 wind turbines with a capacity of 133 MW, while 16 turbines with a capacity of 53 MW generate electricity at the Karlino wind field.
Both sub-projects feed their energy into the Dunowo substation of the Polish transmission system operator PSE S. A. A total capacity of 250 MW is available there, offering the possibility to connect further projects.
Certex UK, based in Doncaster, the UK, has won a major framework to supply statutory inspection and maintenance of Balance of Plant (BoP) equipment at the 114 turbine, 1075 MW Seagreen offshore wind farm, located off the coast of Angus, Scotland, in the North Sea firth. The framework, won via tender, is for three years, plus a two-year extension.
A £3 billion joint venture between TotalEnergies (51%) and SSE Renewables (49%), Seagreen, located approximately 17 miles offshore, will be Scotland’s largest, and the world’s deepest, fixed-bottom offshore wind farm. V164-10 MW turbines have been positioned on each of the 114 bases. First power was recently announced.
Certex UK’s work will primarily relate to the transition jackets in addition to personal protective equipment (PPE), including fall arrest systems, davit cranes, anchor points, and ladders.
Certex UK, which will also deliver services to an operations and maintenance site in Montrose, Scotland, will commit a two-person team, working in rotation via a service operation vessel covering offshore scopes all year round. The company will leverage resources from its own Montrose facility.
Everfuel A/S has announced its plan to build a hydrogen hub in Holstebro, Denmark, to support regional energy transition and accelerate the scale-up of green hydrogen as a zero-emission fuel for industry and mobility. The hub will be developed in two phases in close collaboration with partners within industry and mobility, with the aim of establishing safe, reliable, and efficient local supply of clean energy.
Everfuel, the local municipality, and the other hub partners will work towards a greener Holstebro city by exploring the potential for using green hydrogen in industrial processes and in mobility. By realising all three phases, the hub will enable a significant reduction in the municipality’s CO2 emissions by feeding carbon-free hydrogen into a large share of the municipalitiy’s activities.
In phase one, expected to be commissioned in 2023, the hub-partners will explore production of e-methane and the use of hydrogen in industrial gas burners to produce e-methane at local biogas facilities and start testing using hydrogen in the current natural gas pipelines. In phase two, Everfuel intends to build a 100 MW electrolyser, a hydrogen terminal, a distribution centre, and a hydrogen refuelling station for both heavy-duty and passenger vehicles. The location has already been secured for the electrolysis facility, and the development is planned to commence in 2025.
Air Products and Associated British Ports (ABP) have announced their intention to partner in bringing the first large scale green hydrogen production facility to the UK.
The facility would import green ammonia from production locations operated by Air Products and its products around the world. This would be used to produce green hydrogen, which would decarbonise hard-to-abate sectors such as transport and industry.
The UK government has plans for 10 GW of low-carbon hydrogen to be in production or construction by 2030. Currently, there is no significant domestic production of such hydrogen in the UK. Air Products, as a first mover, aims to help drive progress towards the government’s targets, addressing the urgent task of decarbonising hard-to-abate sectors, and reduce the UK’s dependency on fossil fuels.
The project will bring a wide range of benefits to Immingham and the UK, including eliminating up to 580 000 tpy of greenhouse gas emissions, the equivalent of taking 20 000 diesel heavy good vehicles off UK roads, as well as reducing nitrous oxide and particulate emissions. In addition, the project will bring up to US$4.6 billion in growth and financial benefits to the region, and provide approximately 1400 direct jobs and 1600 indirect jobs for supply chains and local businesses.