The Rise of Digitized Wind Turbine Farms
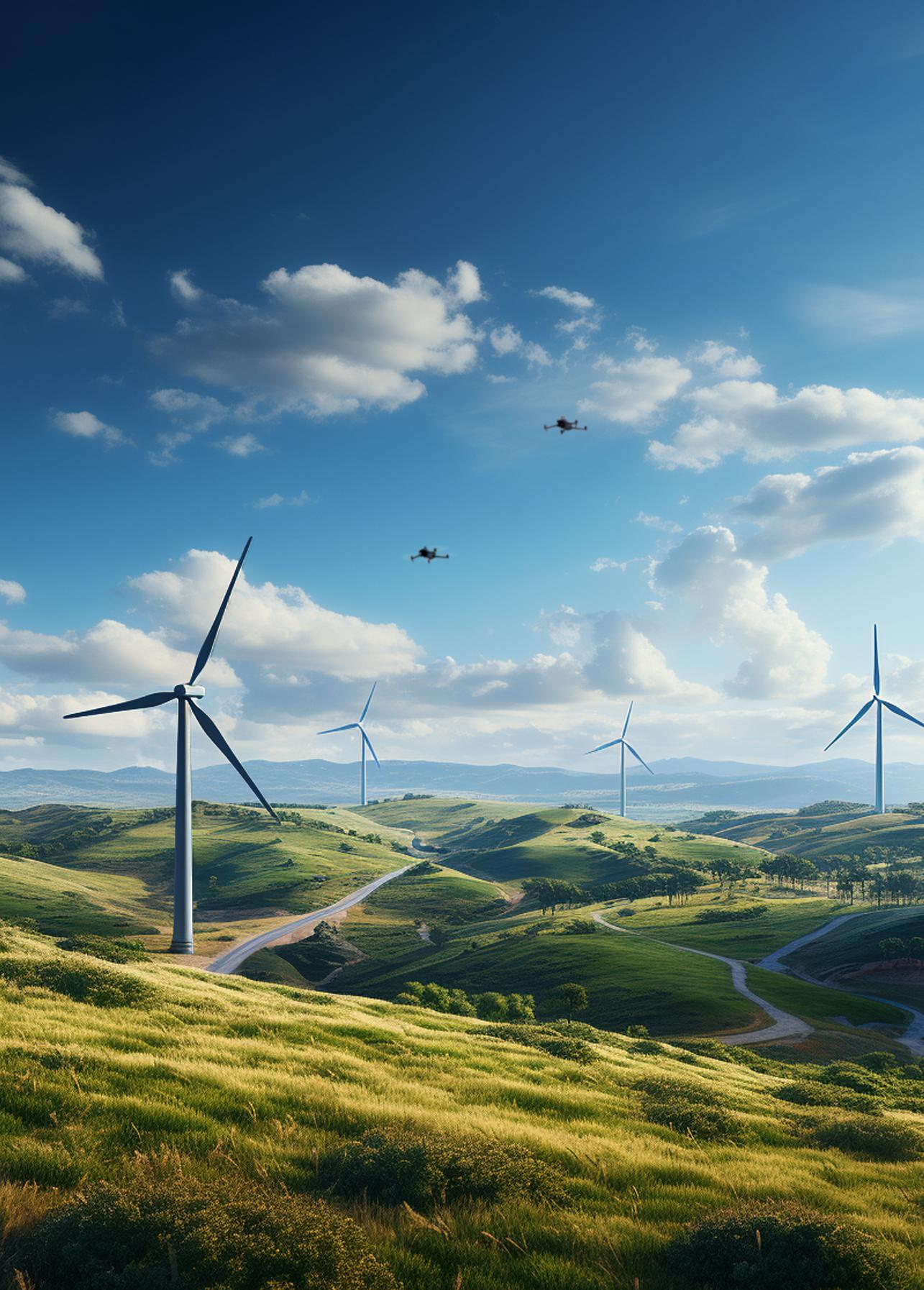
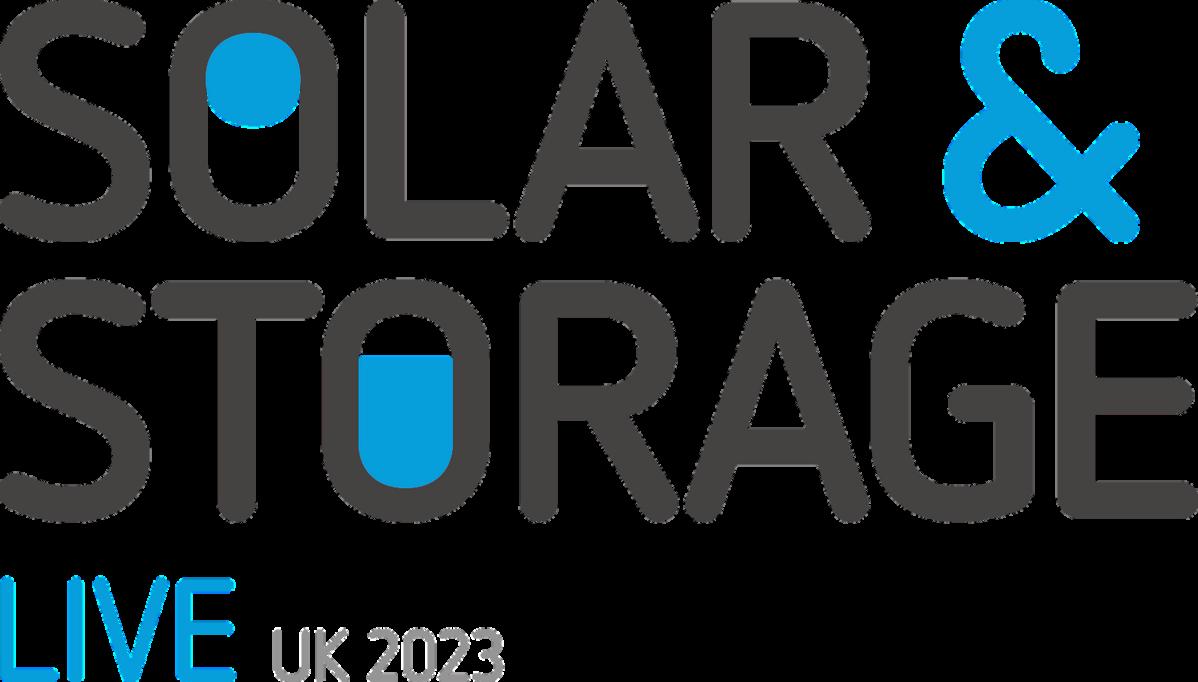
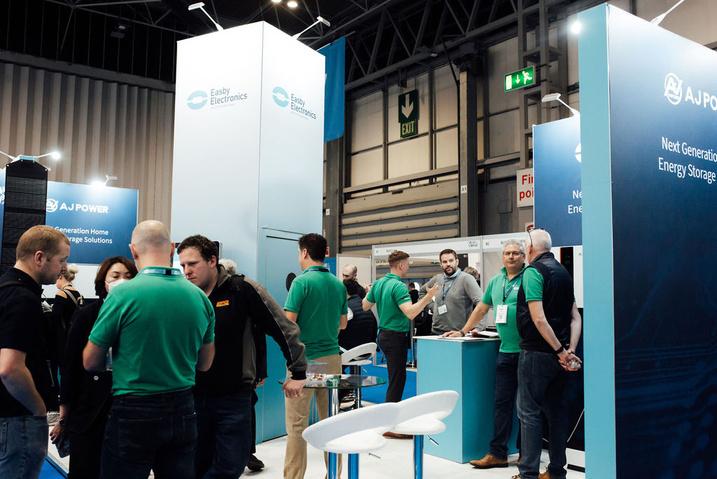
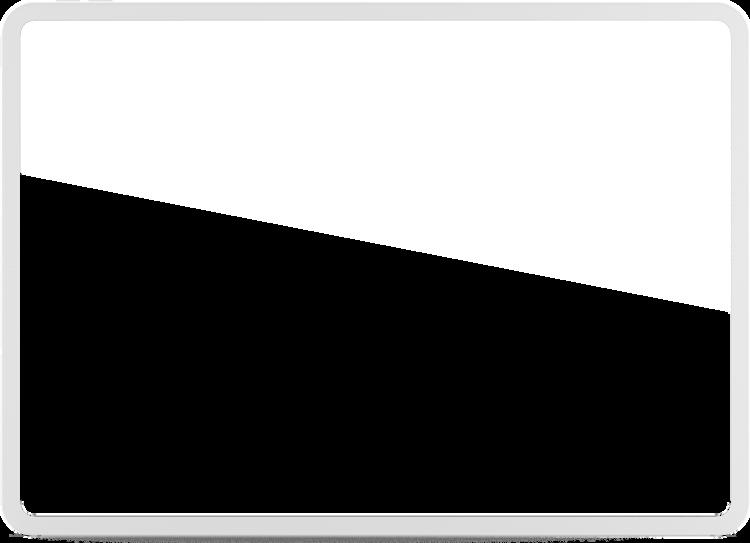
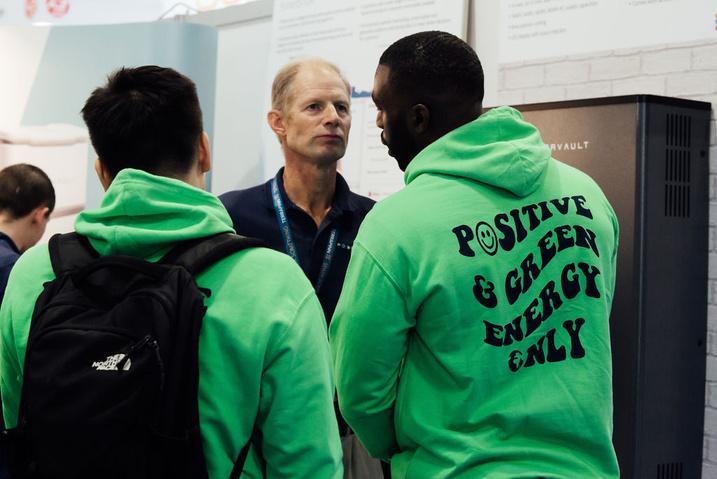
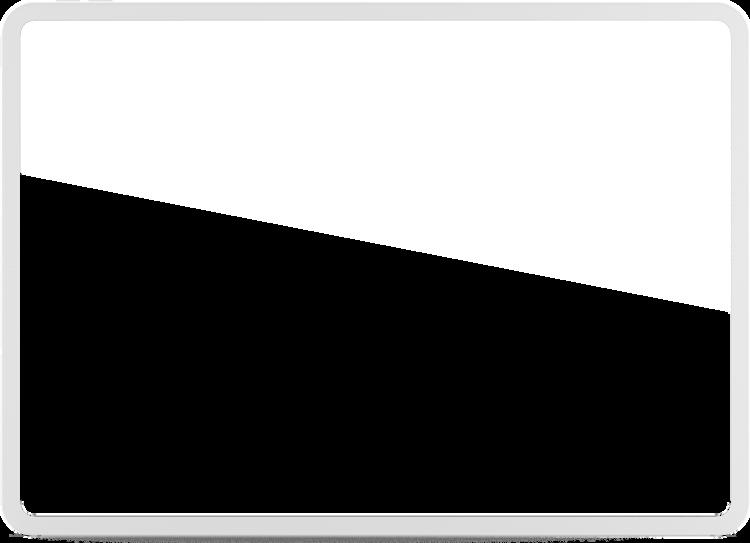
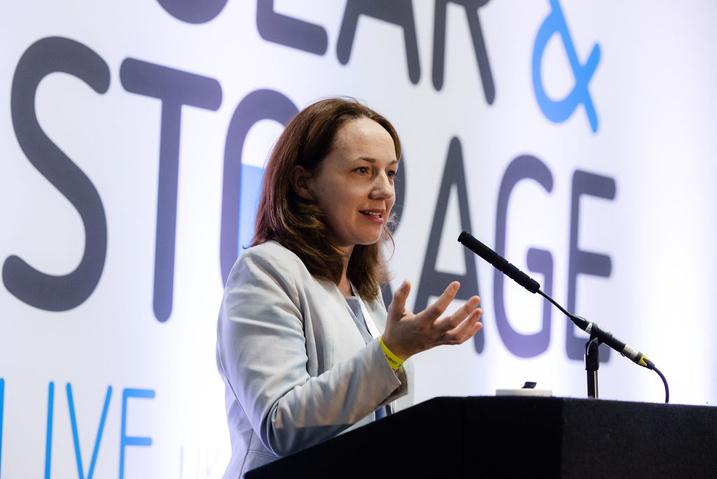
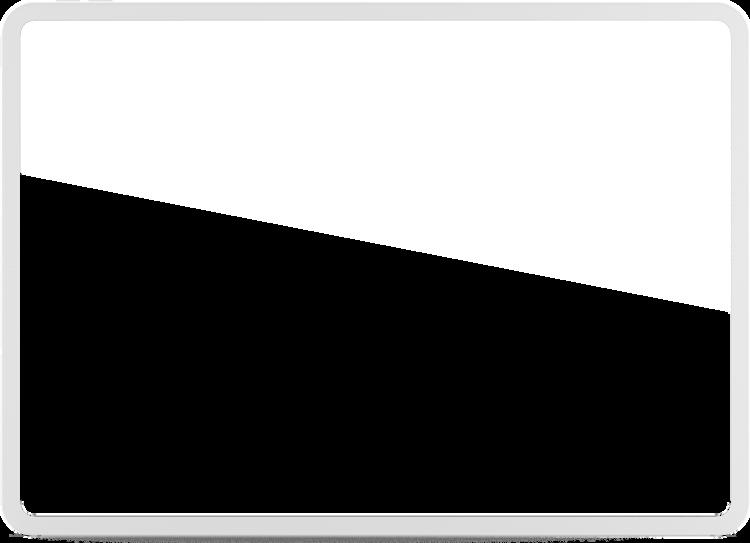
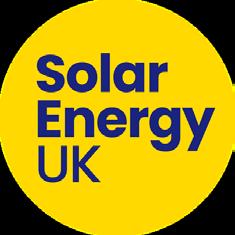
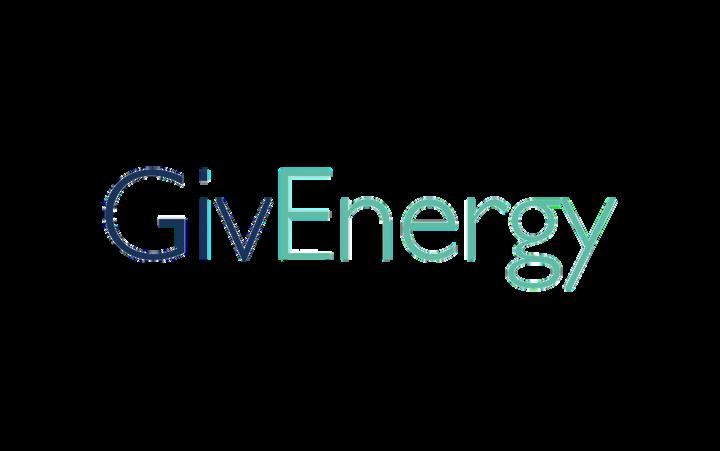
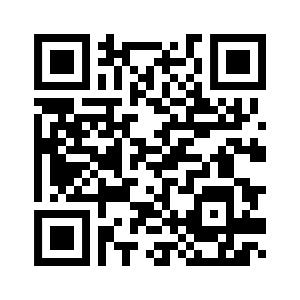
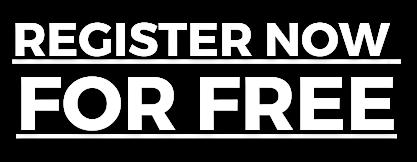
CONTENTS SUMMER 2023
03. Guest comment
04. Exciting times for Europe?
Jessica Casey, Deputy Editor, Energy Global, UK, provides a snapshot of Europe’s growing renewables sector.
26. Progress towards change
Matt Harper, Invinity, Canada, highlights the importance of battery storage solutions in order to achieve a ‘just transition’ in the energy industry.
30. Scale up
Brian D. Jønson, Nature Energy, Denmark, considers how power-to-X is boosting the green transition, with biogas and biomethane presenting viable alternative fuel solutions.
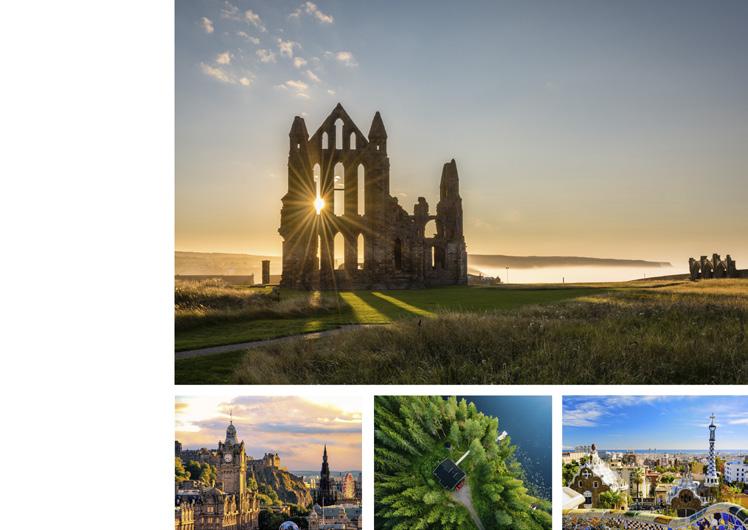
36. Powering sustainable fuels
Sander van Donk, Sulzer Chemtech, Switzerland, looks at how renewable resources are key to decarbonising the transport sector and what technologies are best suited to ensure a reliable supply of quality fuels.
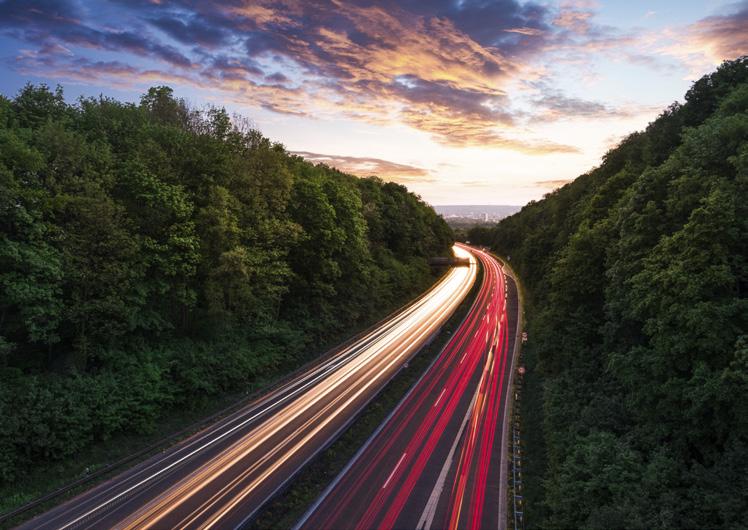
08. Getting ahead of the curve
Dr Jyoti Roy, GreenEnco, UK, discusses how optimisation of solar assets is essential to increasing confidence of green investment in order to reach net zero targets.
12. Unlocking the steps to net zero
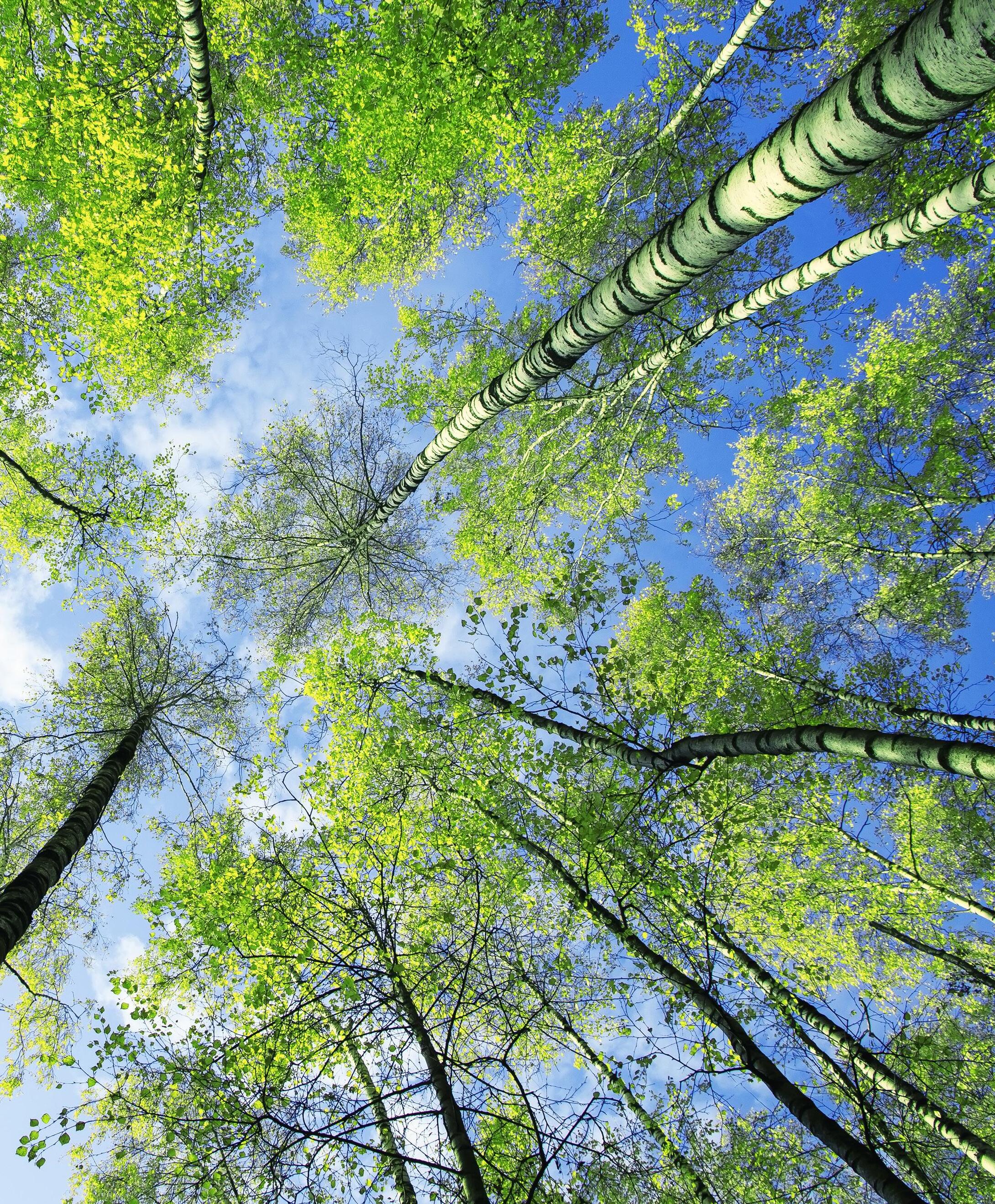
Piotr Loga and Mikołaj Konieczka, Menlo Electric, offer a perspective into how to meet targets in the 'net zero by 2025' scenario.
18. From nice-to-have to a competitive advantage: digitising wind turbine farms
Sharon Imber, CMO, vHive, focuses on the profound impact of wind turbine farms digitisation and how it is reshaping the entire value chain of wind energy, ushering in a new era of efficiency, sustainability, and profitability.
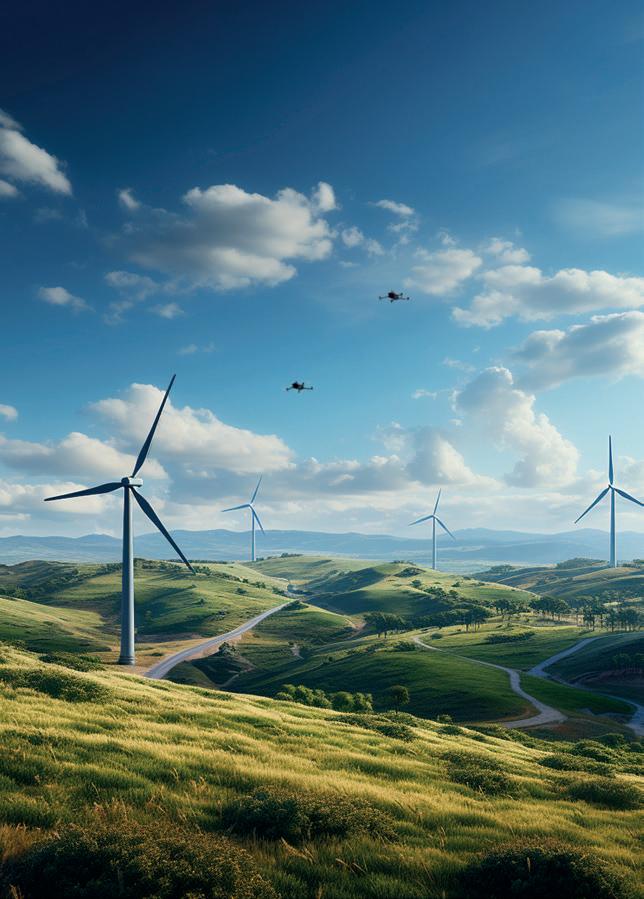
22. Regulating risks
Kristoffer Eldin, Dafo Energy Storage Protection, Sweden, examines the various risks associated with energy storage, namely fire, and the measures needed to control them effectively.
Reader enquiries [enquiries@energyglobal.com]
ON THIS ISSUE'S COVER
40. Taking geothermal to a new level Matt Houde, Carlos Araque, and Kevin Bonebrake, Quaise Energy, USA, detail how accessing the superhot rock deep beneath the ground could help power the world.
44. Global news
GL BAL
vHive is a global leader in digital twin software solutions. The company accelerates enterprises’ continuous digital transformation, enabling them to make better decisions based on accurate field data, analytics, and insights. vHive’s leading software solution enables enterprises to deploy autonomous drone hives to digitise their field assets and operations. The company’s solution is making an impact in various industries by seeking to dramatically cut operational costs, generate new revenue opportunities, and boost employee safety.
Learn more at www.vHive.ai
of Digitized Wind Turbine Farms
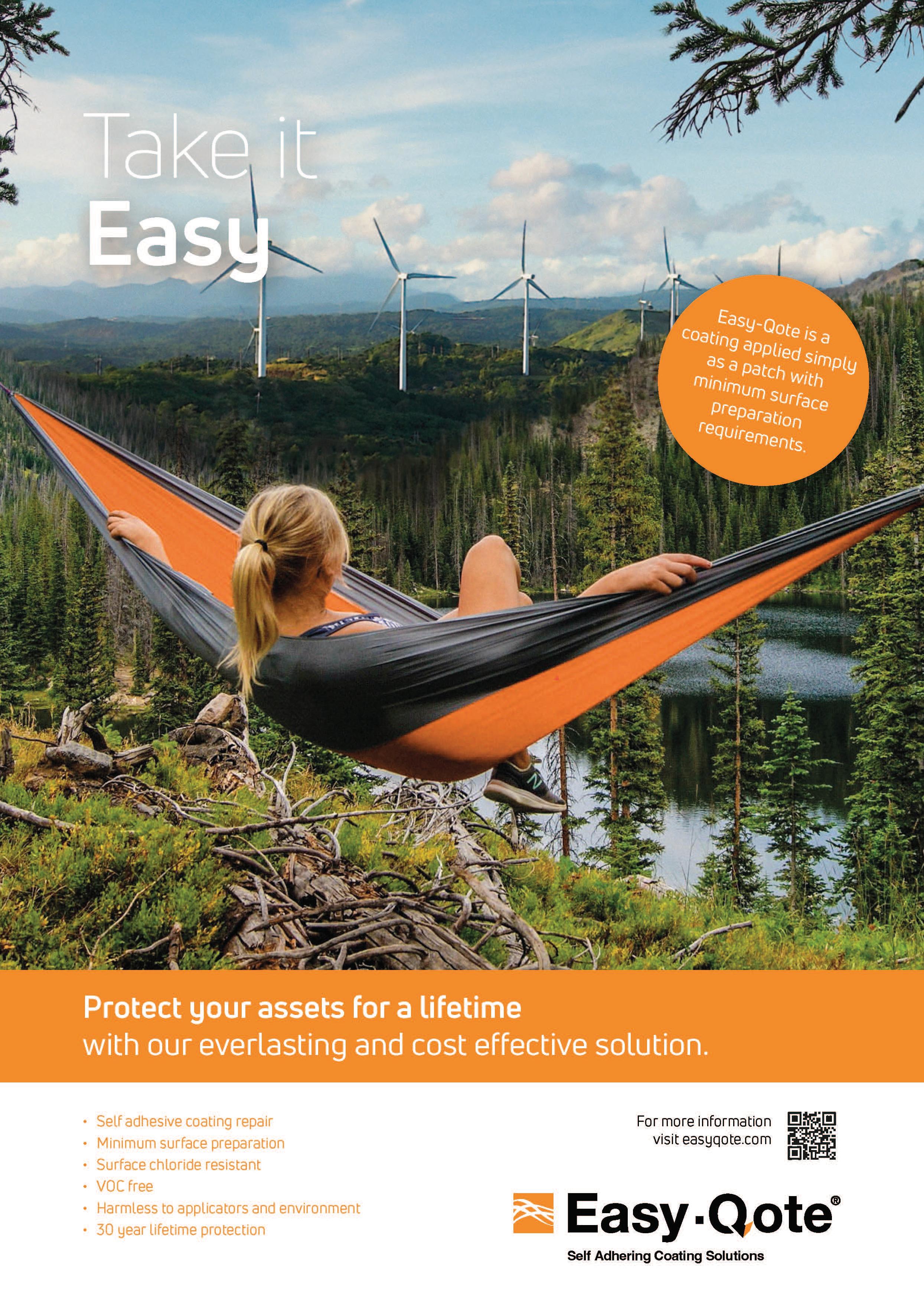
MANAGING EDITOR
James Little james.little@palladianpublications.com
DEPUT Y EDITOR
Jessica Casey jessica.casey@palladianpublications.com
EDITORIAL ASSISTANT
Théodore Reed-Martin theodore.reedmartin@palladianpublications.com
SALES DIRECTOR
Rod Hardy rod.hardy@palladianpublications.com
SALES MANAGER
Will Powell will.powell@palladianpublications.com
PRODUC TION MANAGER
Calli Fabian calli.fabian@palladianpublications.com
DIGITAL EVENTS MANAGER
Louise Cameron louise.cameron@palladianpublications.com
DIGITAL EVENTS COORDINATOR
Stirling Viljoen stirling.viljoen@palladianpublications.com
DIGITAL ADMINISTRATOR
Leah Jones leah.jones@palladianpublications.com
DIGITAL CONTENT ASSISTANT
Merili Jurivete merili.jurivete@palladianpublications.com
ADMINISTRATION MANAGER
Laura White laura.white@palladianpublications.com
Editorial/Advertisement Offices:
Palladian Publications Ltd
15 South Street, Farnham, Surrey, GU9 7QU, UK +44 (0) 1252 718 999 www.energyglobal.com
COMMENT
Anja Frada COO, Energy Business at Wärtsilä
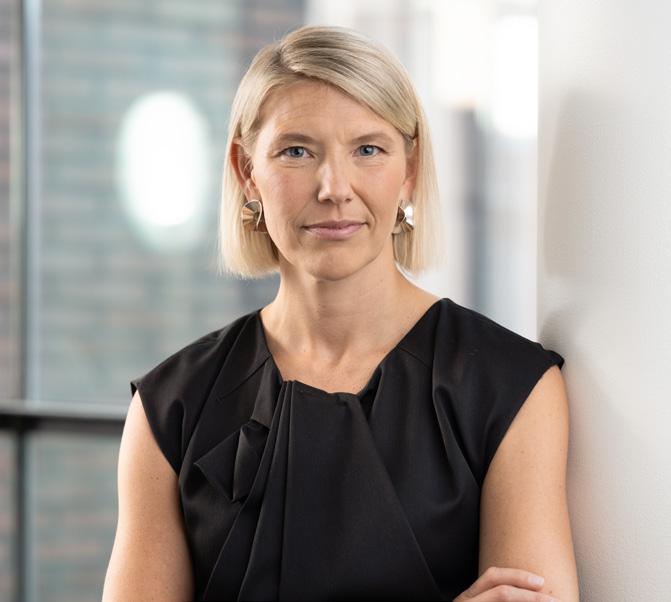
The Inflation Reduction Act in America and REPower EU in Europe are set to supercharge the renewable transition for the next decade with a combination of subsidies, tax cuts, and incentives. But, to make these policies as impactful as possible, roadblocks around energy market designs and permitting still need to be addressed.
In a landmark moment for climate legislation, US President, Joe Biden, signed the Inflation Reduction Act, freeing up US$370 billion in federal funding to turbocharge the US economy and accelerate the shift to clean energy. The act will make use of grants and tax credits for domestic manufacturers and has been a game-changer for clean energy funding.
Following Russia’s invasion of Ukraine, many countries across Europe face coming to terms with their long-term dependence on Russian hydrocarbons and legacy baseload power systems leading to spiralling costs for consumers. The central aim of REPower EU is twofold: to end dependency on Russian gas, and secure affordable renewable energy via €300 billion in loans and grants.
Going forward, Europe will require at least 1100 GW of renewable capacity by 2030 to continue on its decarbonisation journey and achieve its future net-zero goals. That will require us to redesign and reform the EU market, and Wärtsilä has identified three policy recommendations needed to enable this.
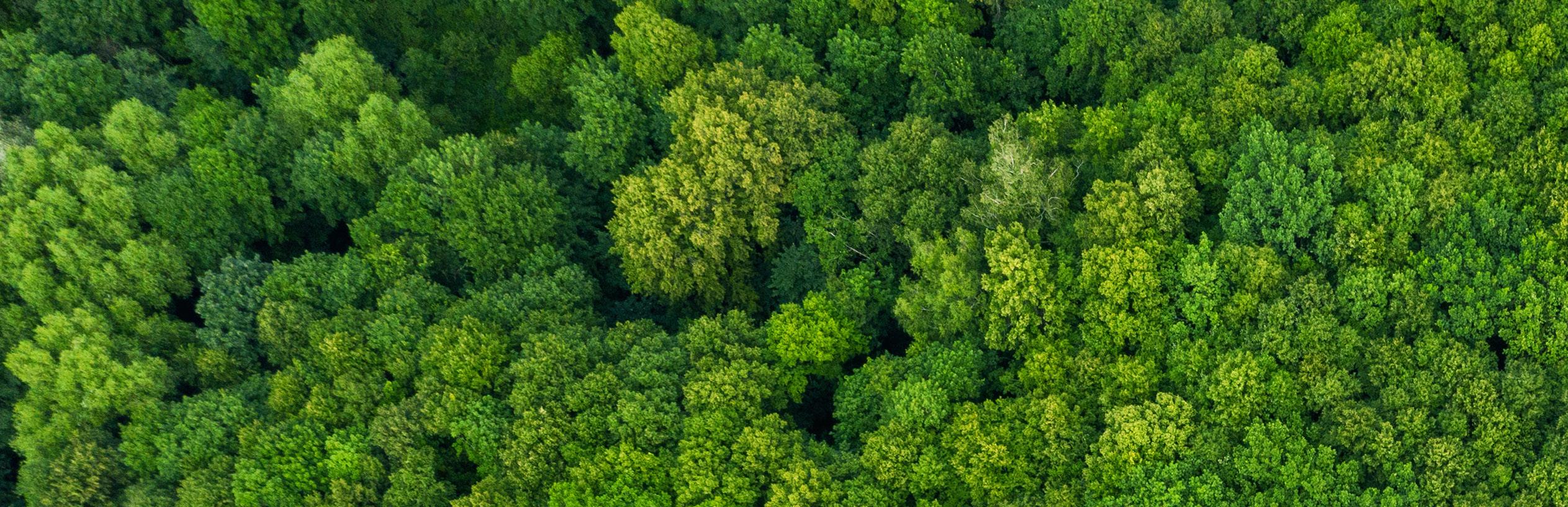
Firstly, an attribute-based capability market is imperative for adding capacity that can sustainably fulfil adequacy and system requirements, which is designed to attract investment in flexible capacity to support renewables, is needed. Similarly, attribute-based
capability addition would also greatly benefit the US market. Secondly, we need to ramp up the deployment of flexible technology to support a renewable-based grid that can dynamically ramp up and down to balance the intermittent nature of renewables. Finally, strong, granular price signals are required to more accurately reflect the physical realities of the grid.
Europe also needs to tackle the issue of permitting and could look to the US for guidance on this. At present, the current system is too slow, with 80 GW of wind currently stuck in planning.1 Unlocking faster permitting will be key for the EU to excel at deploying wind farms and solar panels and help the bloc to remain competitive with the US.
For the energy transition to be successful, competition in the market needs to be recognised and encouraged. This will accelerate the deployment of green technologies and lower costs, making it a no-brainer for consumers and countries.
The 2020s are a pivotal decade in our race against climate change and its impacts on not only the environment but also economies across the globe. The Inflation Reduction Act and REPower EU show the right level of ambition needed to help create the renewable energy future we want; now we need to address market designs and permitting to get renewable energy sources up and running at scale as quickly as possible.
References
1. ‘EU wind installations up by a third despite challenging year for supply chain’, WindEurope, (11 January 2023), https://windeurope.org/newsroom/pressreleases/eu-wind-installations-up-by-a-thirddespite-challenging-year-for-supply-chain
The role of renewables has become increasingly important around the globe over the past 1 – 2 years, especially across Europe. The large majority of European countries have started to decrease their reliance on Russian gas imports and move away from traditional fossil fuels. Along with the EU’s recent agreement in March 2023 on stronger legislation to increase its renewables capacity (the EU’s binding target for 2023 was raised to 42.5%, which would almost double the existing share of renewable energy in the region),1 it is clear to see that the energy transition has been widely accelerated.
Over the past year, and for the first time, Europe generated more electricity from wind and solar than gas.1 There has also been an increase in capacity, with a record 41 GW of new solar energy capacity installed and an increase in wind capacity by 16 GW.1
While some of the world’s top renewable energy producing countries can be found in Europe, including Iceland, Norway, and Sweden (mostly consisting of geothermal, hydropower, and wind energy), 2 it could be argued that some nations were not taking advantage of its natural resources, notably sun and wind. However, many countries are now taking steps to meet decarbonisation goals by increasing the amount of installed renewable capacity.
The UK Offshore wind
As an island, the UK is surrounded by predominantly open seas (i.e. the North Sea), which can reach
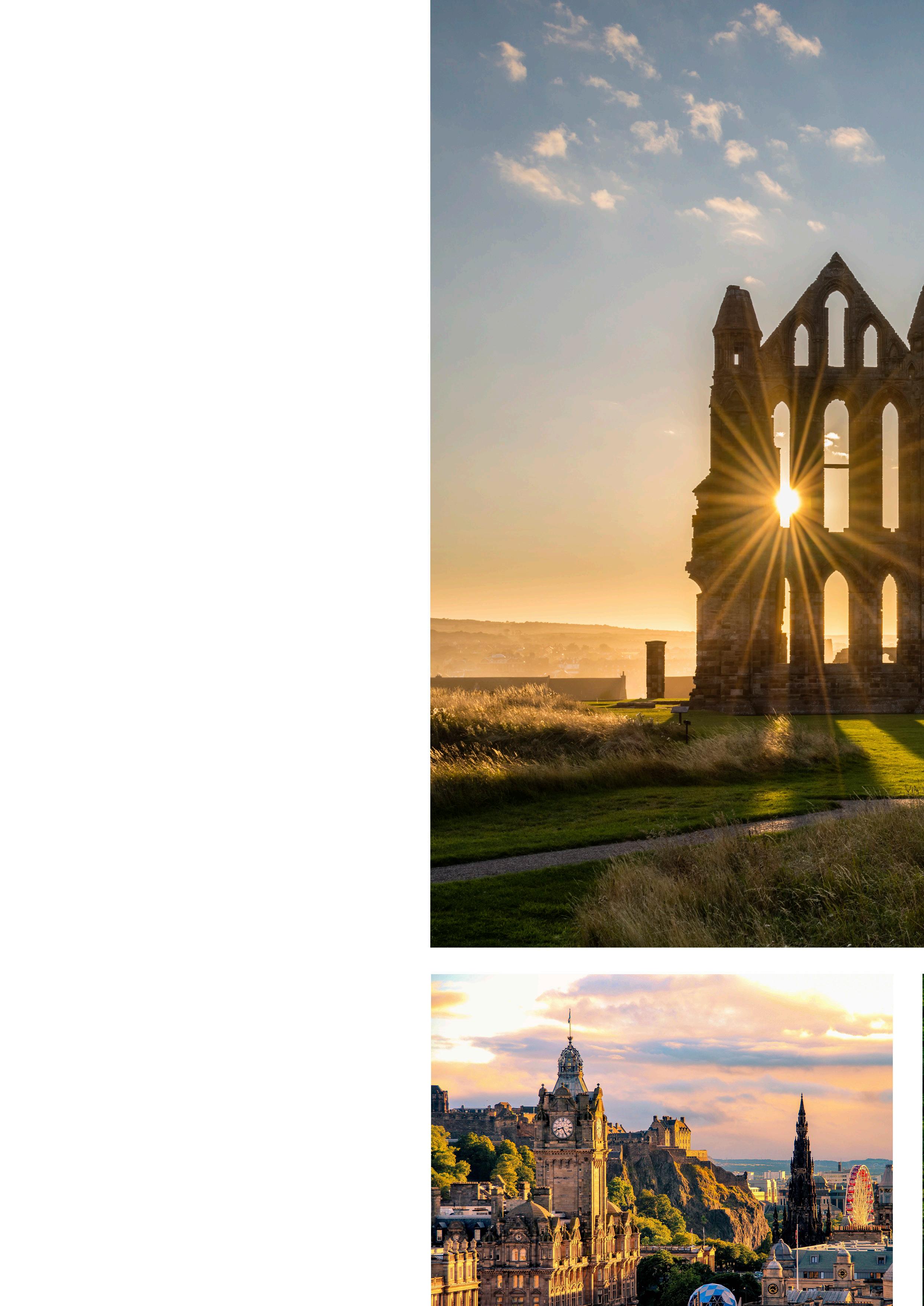
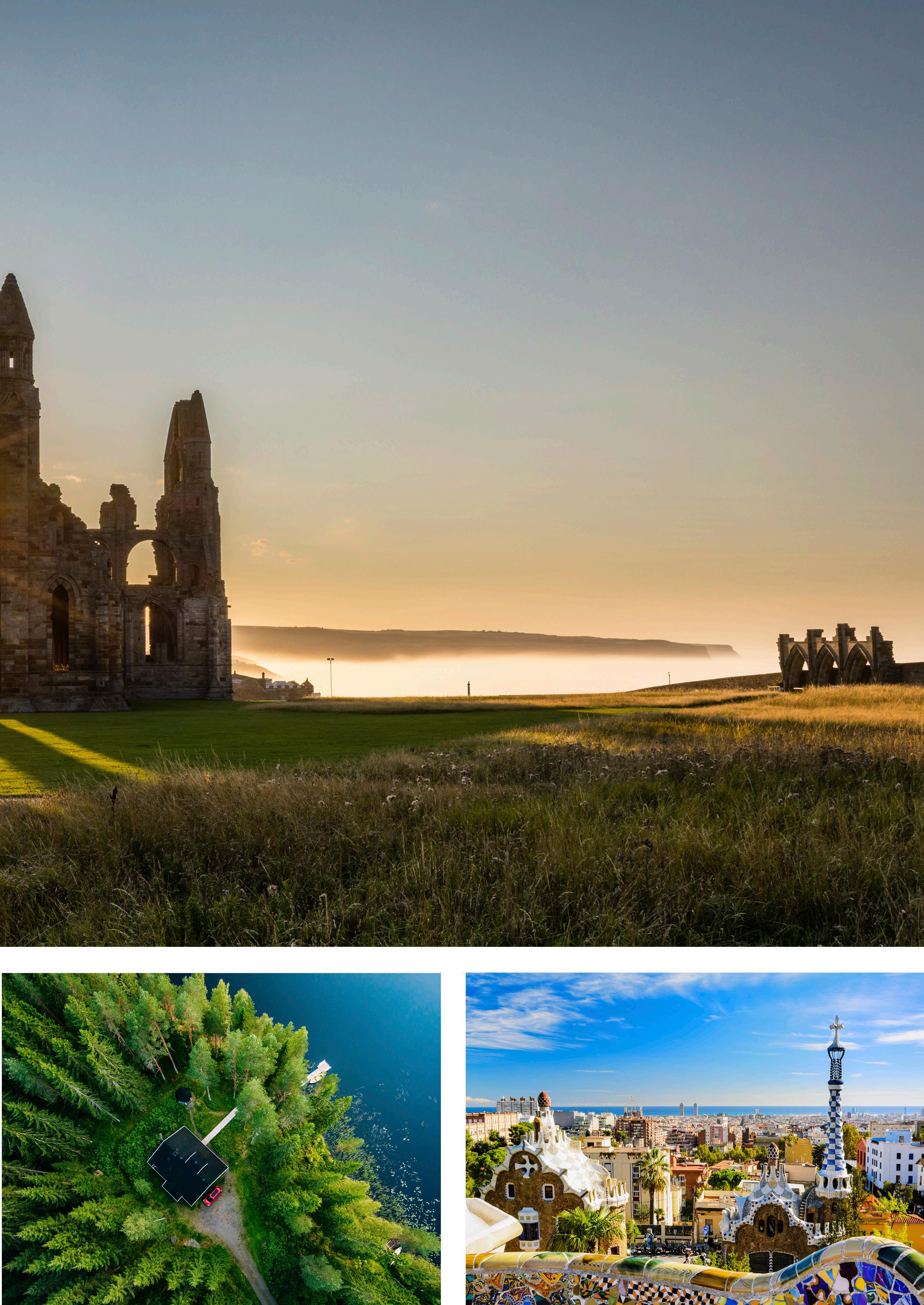
wind speeds of over 10 m/sec. 3 This makes the UK well positioned to capitalise on the available wind resources, something which seems to be the case. Since 2019, offshore wind has dominated new installed capacity, representing 57 of the total. 4 In terms of renewables’ share of electricity generation, offshore wind also continues to dominate with a 4.3 percentage point increase compared to 1Q22. 4
In Scotland, there is a significant amount of additional capacity in development, with planned or consented projects totalling over 21 GW. Offshore wind has 3.92 GW already consented, with an additional 4.2 GW in planning. 5 Recently, Seagreen wind farm, a joint venture between SSE Renewables and TotalEnergies, installed the 114 th and final wind turbine at the site, located 27 km from the Angus coast. Once fully operational, this will be Scotland’s largest offshore wind farm and will be capable of generating approximately 5000 GWh/y of renewable energy. 6
In England, Hornsea 2, the world’s largest wind farm, entered full operation in autumn 2022, comprising of 165 wind turbines. The Hornsea Zone, an area of the North Sea covering more than 2000 km 2 , will also include Hornsea 3.7 Earlier this year, Ørsted appointed Havfram Wind to install turbines at the Hornsea 3 offshore wind farm, starting autumn 2026. 8 Moreover, Ørsted has recently signed a contract with Hellenic Cables for the supply of inter-array cables for Hornsea 3.9 Prysmian has also been awarded a contract to supply the inter-array submarine cable system that will connect the wind turbines and the offshore converter station.10 With a capacity of 2.85 GW, Hornsea 3 will be able to produce enough power for over 3 million UK homes, making a significant contribution to the UK government’s ambition of having 50 GW of offshore wind in operation by 2030. When fully online, the Hornsea wind farm – comprising of Hornsea 1, 2, and 3 – will have a total capacity in excess of 5 GW.9
The other major development for the UK offshore wind industry is Dogger Bank wind farm, a joint venture partnership between SSE Renewables (40%), Equinor (40%), and Vårgrønn (20%). The wind farm is being developed in three phases – Dogger Bank A, B, and C – located between 125 – 290 km off the east coast of Yorkshire in the North East of England, and collectively will become the world’s largest offshore wind farm.11 The electrical engineering division of the Correll Group has also been contracted by DEME Offshore NL B.V. to complete the 66 kV inter-array cable termination and testing on 95 wind turbine generators and the offshore substation platform on the first phase of the wind farm, including the installation of a cable monitoring system.12 In addition, the largest offshore jack-up installation vessel ever built, Voltaire, arrived in the port of Able Seaton in June 2023. Jan De Nul Group’s vessel was delivered in 4Q22 and has since been undertaking final preparations for the construction of the Dogger Bank wind farm phases.13 In total, the vessel will be responsible for installing 277 units,
sailing out in early July to install the first GE Haliade-X offshore wind turbine. These turbines are some of the world’s largest and most powerful offshore wind turbines in operation today, and, as the first order, Dogger Bank will be the first time the turbines are installed – one rotation of the turbine blade can power one UK home for more than two days.12
Onshore wind will also play a part in the UK energy mix, particularly in Scotland where onshore wind is currently the biggest single technology. Vattenfall has recently inaugurated South Kyle wind farm, which will have an installed capacity of 240 MW once fully commissioned,14 and EDF Renewables is exploring the potential for the proposed 400 MW Liddesdale onshore wind farm development across two areas of commercial forestry within the Scottish Borders.15
Sweden
Offshore wind
Sweden has recently changed it target of being 100% fossil free to reaching 100% renewable energy production by 2040.16 Currently, nearly 75% of Sweden’s electricity production is renewable (hydropower [43%] and nuclear [31%]).16 However, wind capacity is also expanding in Sweden – the country now has the fifth most onshore wind capacity in Europe, and were projected to have 14 GW by the end of 2022.17
Like the UK, Sweden is also well positioned to capitalise on its wind power potential due to the pocket of Baltic Sea and vast coastline. 3 The country is taking steps to utilise these resources, and invest more in its offshore wind potential. The government has identified three areas ‘suited for’ offshore wind: in the Gulf of Bothnia in northern Sweden, the Baltic Sea, and the Kattegat sea area.17 15 GW of offshore wind capacity is in the process of applying for permits and could come online before 2030.17 In 2022, Vattenfall was granted a construction permit by the Swedish government for an offshore wind farm at Swedish Kriegers Flak.18 If everything goes according to play, the wind farm can be commissioned around 2028, and will generate an annual capacity of approximately 2.6 TWh. Vattenfall has already commissioned the Danish side of Kriegers Flak, which is Denmark’s largest wind farm, and the wind farm on the German side has been in operation since 2015.18
Among the most impressive project proposals is OX2’s plan to build the 5.5 GW Aurora offshore wind farm between the islands of Gotland and Öland, with the potential to cover approximately 17% of Sweden’s electricity consumption alone. OX2 has also applied for permits to build two other gigawatt-scale projects in Sweden’s Baltic Sea – the Galatea-Galene project (1.7 GW) and the Triton project (up to 1.8 GW).17
On top of that, there are also plans to build floating wind turbines. Freja Offshore, a joint venture between Aker Offshore Wind and Hexicon, is proceeding with a pipeline of four floating wind projects with a combined
capacity of 8 GW in Southern Swedish waters.17 Big floating wind projects in Sweden are also being planned by other companies, including Simply Blue Group (Skidbladner floating wind project and GW Herkules floating wind project),19 and Deep Wind Offshore (Erik Segersäll), 20 among others.
Spain Solar
According to Rystad Energy forecasts, Spain is on track to generate more than half of its power from renewable sources in 2023. 21 Spain is already a major player in wind energy, and had 1298 wind farms installed in 2021, second only to Germany in Europe. 22 Between 2022 – 2025, the country is set to install 19 GW of new capacity, according to the latest baseline forecast from industry group, SolarPower Europe. In October 2022, there were over 100 GW of projects with grid approval, and 23 GW awaiting permission. 21 Spain has a bit of problem with permitting new renewables projects, and with the amount of proposed projects constantly increasing and the permitting of solar and wind projects in Europe potentially taking around five years, compared with less than one year for construction, this permitting process must be accelerated if Europe wants to meet its projected decarbonisation goals. 21 In March 2023, Spain’s government did implement new planning rules that shorten the permitting process for projects of capacity below 150 MW that have low or medium impact on the environment. The new rules, in place until 2024, could halve the time it takes to receive permits to approximately two years, but the benefits will depend on the stage of the project and whether companies will prioritise on larger projects. 21
Regardless, there have been a lot of recent solar developments in Spain. RWE has commissioned the Casa Valdes’ sister project, Puerta del Sol, bringing RWE’s operational solar capacity to more than 140 MW. 23 In addition to these two solar farms that were commissioned in 2023 RWE is in the commissioning phase of Las Vaguadas (10 MW), and the Gazules project, which consists of two solar farms. 23 Moreoever, BayWa r.e. and NORD/LB have recently completed the financing of a solar park in Alhendín. The solar farm was scheduled for completion at the end of June 2023, and to be connected to the grid by the end of 2023. Approximately 10% of the solar project will also consists of an agrivoltaic installation, which is becoming increasing popular due to the dual use of land for energy generation and agriculture. 24 TotalEnergies has also obtained a favourable economic impact assessment for an estimated 3 GW of installed solar capacity from the Spanish government. This result relates to the 48 power plants that the company will develop in the Madrid region, Murcia region, Castilla-La Mancha, Andalusia, and Aragon, with the first projects expected to come onstream in 2024. 25
Conclusion
In the end, the whole of Europe will have to be on the same page to work towards its shared goal of zero emissions and reduce reliance on traditional fossil fuels. There is still a long way to go, but the investment in new proposed projects, technology developments, and government agendas suggest European countries, and the continent as a whole, are moving in the right direction.
References
1. ‘REPowerEU at a glance’, European Commission, https://commission.europa.eu/ strategy-and-policy/priorities-2019-2024/european-green-deal/repowereu-affordablesecure-and-sustainable-energy-europe_en
2. ‘Renewable Energy by Country’, Wisevoter, https://wisevoter.com/country-rankings/ renewable-energy-by-country/
3. ‘Global Wind Atlas’, Department of Wind Energy: Technical University of Denmark and World Bank Group, https://globalwindatlas.info/en/
4. ‘Energy Trends: UK, January to March 2023’, Department for Energy Security & Net Zero, (29 June 2023), https://assets.publishing.service.gov.uk/government/uploads/ system/uploads/attachment_data/file/1165982/Energy_Trends_June_2023.pdf
5. ‘Statistics’, Scottish Renewables, www.scottishrenewables.com/our-industry/statistics
6. ‘Final wind turbine installed at Scotland’s largest offshore wind farm’, SSE Renewables (22 June 2023), www.sserenewables.com/news-and-views/2023/06/final-wind-turbineinstalled-at-scotland-s-largest-offshore-wind-farm/
7. ‘Hornsea 2, the world’s largest windfarm, enters full operation’, Ørsted (31 August 2022), https://orsted.co.uk/media/newsroom/news/2022/08/hornsea-2-theworlds-largest-windfarm-enters-full-operation
8. ‘Havfram Wind to install turbines at Ørsted`s Hornsea 3 offshore wind farm’, Havfram (21 March 2023), https://havfram.com/news/press-release
9. ‘Ørsted agrees Hornsea 3 inter-array cable deal with Hellenic Cables’, Ørsted (22 June 2023), https://orsted.co.uk/media/newsroom/news/2023/06/orsted-helleniccables
10. ‘Prysmian to develop a new submarine power cable link for the Hornsea 3 offshore windfarm in the UK’, Prysmian Group, (3 July 2023), www.prysmiangroup.com/en/ media/press-releases/prysmian-submarine-power-cable-link-hornsea-3-offshorewindfarm-uk
11. Dogger Bank Wind Farm, https://doggerbank.com/about/
12. ‘Correll Group secures contract on Dogger Bank Wind Farm with DEME Offshore’, Dogger Bank Wind Farm, (2 June 2023), https://doggerbank.com/supply-chain/correllgroup-secures-contract-on-dogger-bank-wind-farm-with-deme-offshore/
13. ‘Largest jack-up vessel Voltaire arrives in the UK to build largest wind farm in the world’, Jan De Nul Group, (19 June 2023), www.jandenul.com/news/largest-jack-vesselvoltaire-arrives-uk-build-largest-wind-farm-world
14. ‘Vattenfall inaugurates its largest onshore wind farm in the UK – South Kyle’, Vattenfall, (14 June 2023), https://group.vattenfall.com/press-and-media/newsroom/2023/ vattenfall-inaugurates-its-largest-onshore-wind-farm-in-the-uk--south-kyle
15. ‘EDF Renewables UK to explore potential for 400MW renewables project in Scottish Borders’, EDF Renewables, (21 June 2023), www.edf-re.uk/news-and-views/edfrenewables-uk-to-explore-potential-for-400mw-renewables-project-in-scottish-borders/
16. ‘Energy use in Sweden’, Swedish Institute, https://sweden.se/climate/sustainability/ energy-use-in-sweden
17. ‘Sweden: Making up lost ground on offshore wind’, WindEurope, (12 August 2022), https://windeurope.org/newsroom/news/sweden-making-up-lost-ground-on-offshorewind/
18. ‘Swedish Government grants permit for offshore wind farm Kriegers Flak’, Vattenfall (19 May 2022), https://group.vattenfall.com/press-and-media/pressreleases/2022/ swedish-government-grants-permit-for-offshore-wind-farm-kriegers-flak
19. ‘Simply Blue Group Unveils Multi GigaWatt (GW) of Offshore Floating Wind Projects in Sweden’, Simply Blue Group, (18 May 2022), https://simplybluegroup.com/news/simplyblue-group-unveils-multi-gigawatt-gw-of-offshore-floating-wind-projects-in-sweden/
20. ‘Sweden’, Deep Wind Offshore, https://www.deepwindoffshore.com/sweden
21. ‘Spain’s renewable power generation to top 50% in 2023, surpassing European neighbors on strong wind and solar capacity’, Rystad Energy, (8 June 2023), https://www.rystadenergy.com/news/spain-s-renewable-power-generation-to-top-50in-2023-surpassing-european-neighbor
22. ‘Wind Energy in Spain’, Spanish Wind Energy Association, https://aeeolica.org/en/ about-wind-energy/wind-energy-in-spain/
23. ‘RWE expands solar business in Spain with new large-scale solar farm in operation’, RWE, (26 June 2023), www.rwe.com/en/press/rwe-renewables-europe-australia/202306-26-rwe-expands-solar-business-in-spain-with-new-large-scale-solar-plant-inoperation/
24. ‘BayWa r.e. % NORD/LB complete financing of 54 MWp solar farm’, BayWa r.e (30 May 2023), www.baywa-re.com/en/news/details/baywa-re-nord-lb-completefinancing-of-54-mwp-solar-farm
25. ‘Spain: TotalEnergies Obtains Favorable Environmental Impact Assessment for 3 GW of Solar Projects’, TotalEnergies, (22 May 2023), https://totalenergies.com/media/ news/press-releases/spain-totalenergies-obtains-favorable-environmental-impactassessment-3
Deployment of solar photovoltaic (PV) projects across the globe have continued, despite challenges in recent years, and most likely the current financial year might witness the 1000 GW landmark of global solar PV installation. It has been observed that many of these existing solar asset owners are facing generation loss of their assets and lower revenue than expected. This has become a concern for the developers, investors, independent power producers, and the distribution companies. Some asset owners are more active than others to address these challenges to overcome under-performance and search for realistic solutions to boost revenue.
To avoid the loss of revenue from the exiting assets, a data-driven decision-making process is crucial. Additionally, the reactive nature of operational and maintenance (O&M) response keeps the asset owners behind the expected generation curve. That is why a modernisation of asset management with integrated data analytics and proactive O&M is key for the asset owners to be ahead of the curve. It is also important to take a holistic view when it comes to modernisation of asset management. It is critical to undertake a custom-made pragmatic approach of digitisation on a project to discover the true potential of the assets.
Photovoltaic asset performance management
GreenEnco has developed a systematic optimisation approach (PV asset performance
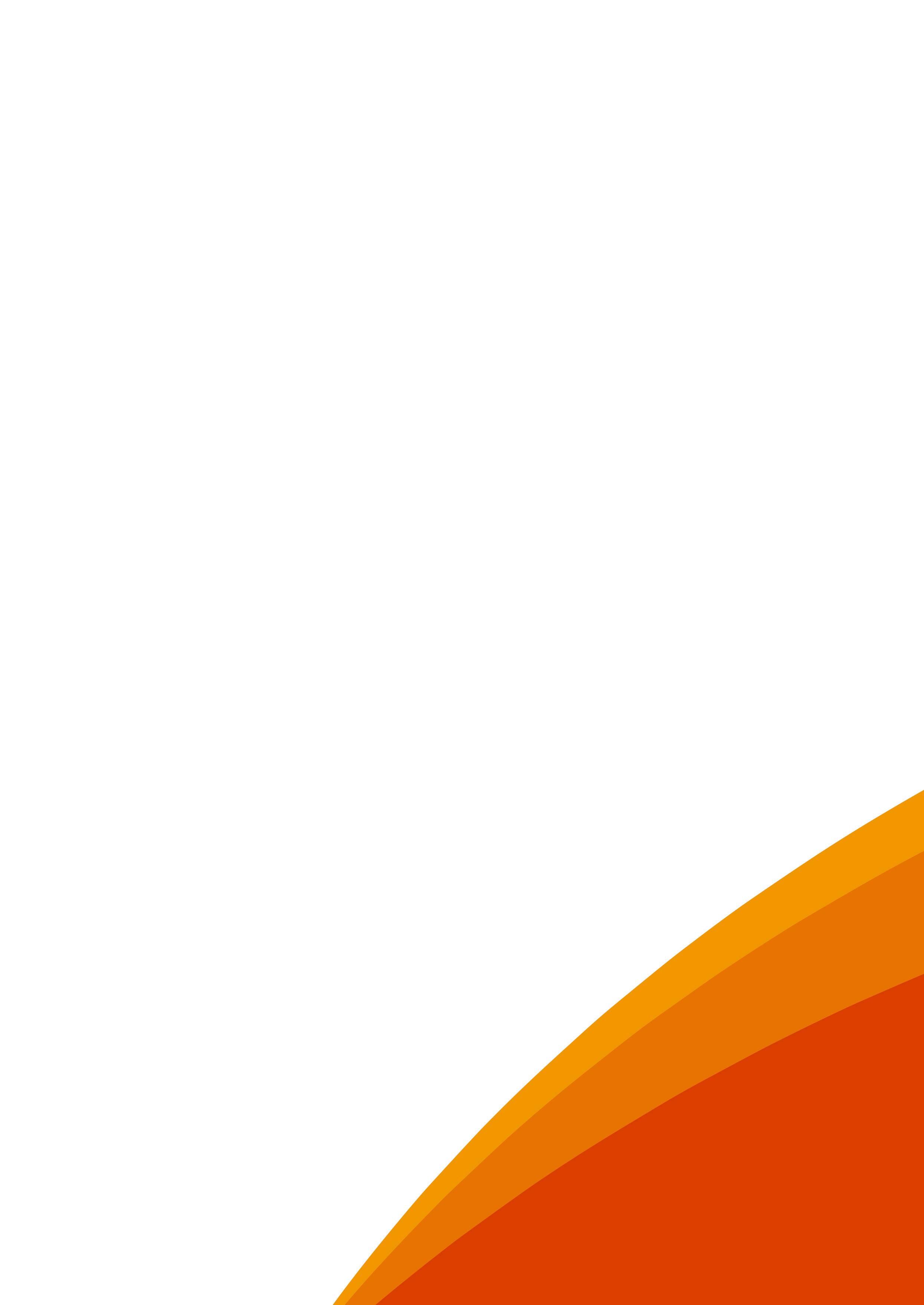
management [PVAPM]) for the operational solar assets to address generation loss and provides solutions to maximise the revenue. GreenEnco’s in-house PVAPM development has been successfully demonstrated to different portfolios of solar assets in Asia and Europe with an average 8% generation gain. PVAPM is not just a software, but it is a strategy which combines ‘high tech’ (i.e. artificial intelligence [AI] and machine learning [ML] algorithms) and ‘high touch’ (solar domain intelligence), for asset owners to unfold the true value of their assets.
PVAPM algorithms have been designed and developed by the GreenEnco team based on decades of experience in academic and industrial domains in the field of solar PV. These algorithms have been validated in peer reviewed academic journals and real-world industrial applications. PVAPM is designed and validated to overcome operational challenges for the asset owners, asset managers, independent power producers, developers, and O&M contractors. Implementation of PVAPM can easily be made with an existing monitoring system SCADA with any PV module, inverter, and module mounting structure technologies. PVAPM is flexible to integrate with an existing system, which reduces decision-making time for the users.
It requires operational data from an existing monitoring system (or SCADA), which includes electrical data, meteorological data, and system configurations. Separate algorithms for each individual component are designed to optimise the overall plant performance. PVAPM then follows three different levels of analysis, as presented in Figure 1.
Dr Jyoti Roy, GreenEnco, UK, discusses how optimisation of solar assets is essential to increasing confidence of green investment in order to reach net zero targets.
PVAPM provides a full cycle optimisation strategy to identify the root cause of an issue using a fault tree analysis, followed by a custom-made improvement solution for each issue at the component level. Prescriptive analytics focuses primarily on the lower capital-intensive scopes to help maximise the financial return for an asset owner. If any measure indicates towards high capital investment, then a cost benefit analysis is provided to help the commercial decision-making process.
Some key interventions, such as thermal inspection of modules and other components, current-voltage (IV) curve test, electroluminescence (EL) test of module, etc., are commonly conducted by the asset owners and asset managers. Sometimes, these interventions are performed independently without comparing each other and without identifying correlations between them. PVAPM is a tool which establishes a correlation of on-site interventions and desktop data analysis, which is a holistic asset optimisation approach.
PVAPM also incorporates maintenance-related data and other on-site services against long-term energy production metrics. Advanced software and information
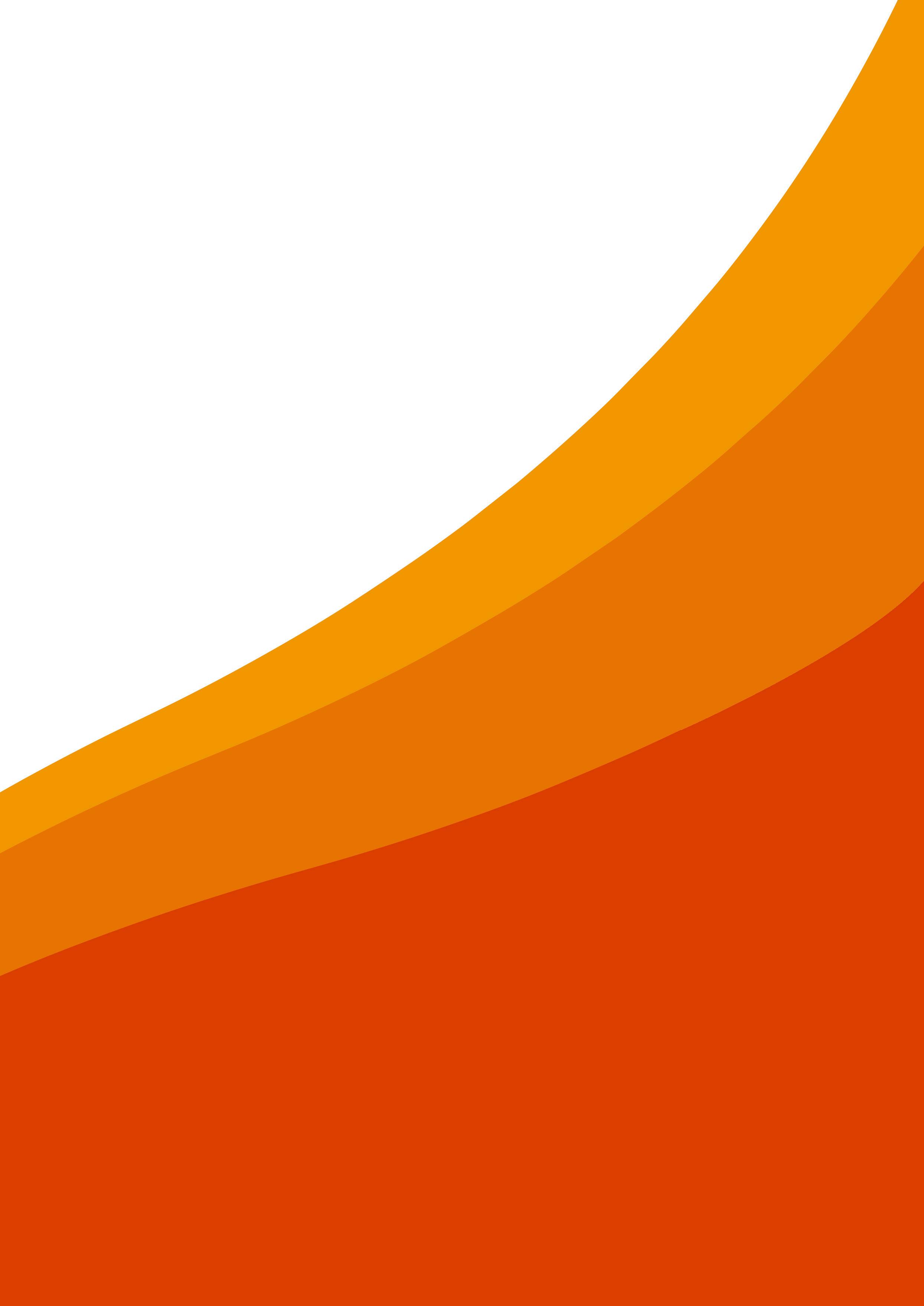
ead ofthecurve
visualisation systems reveal sensitive data deviations that can help detecting performance issues and pinpoint potential problems, which are often difficult to identify by physical inspections. The ultimate aim of PVAPM is addressing a proactive maintenance strategy and generation gain for asset-owners.
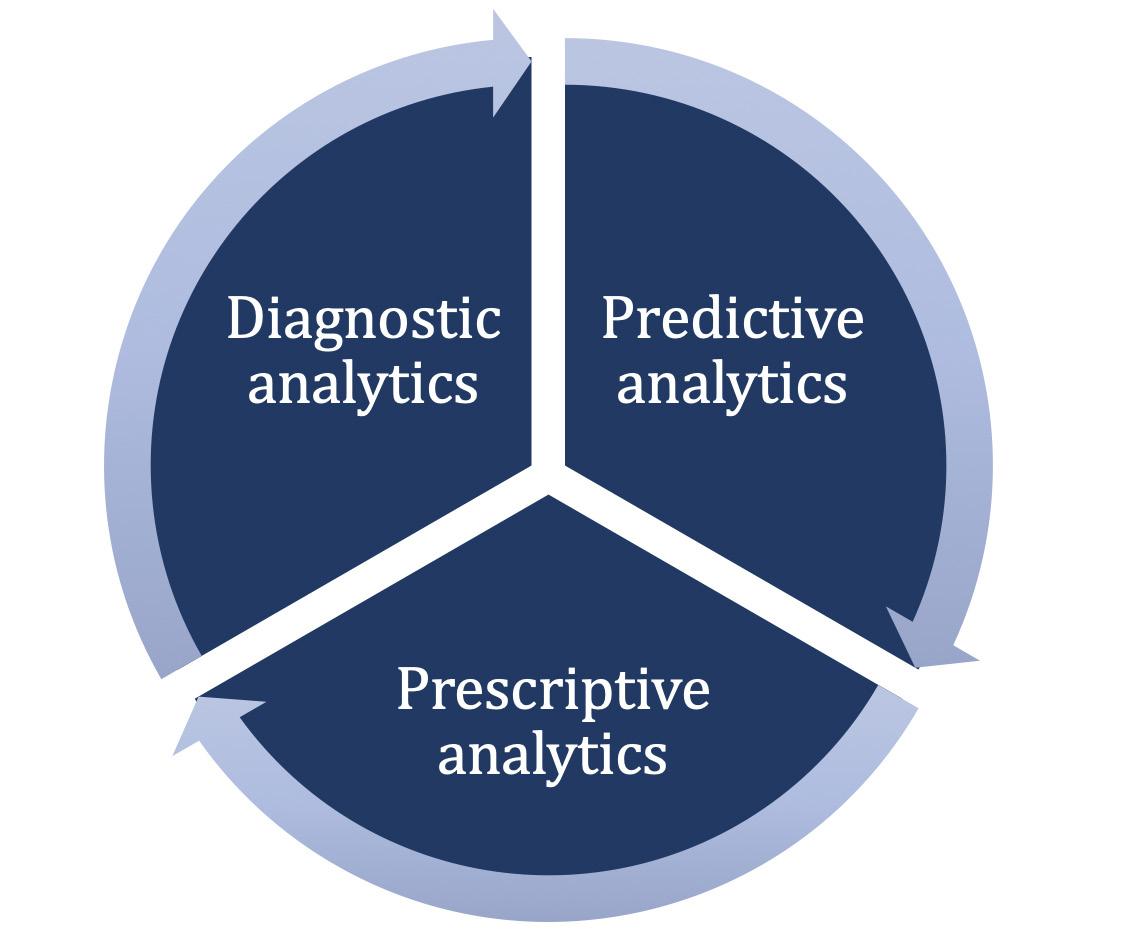
Asset owners in Europe and Asia are benefiting from PVAPM systematic strategies, and some of the selective case studies are presented in Figure 3. These cover crystalline and thin film module technologies, string and central inverters for ground mounted and rooftop systems.
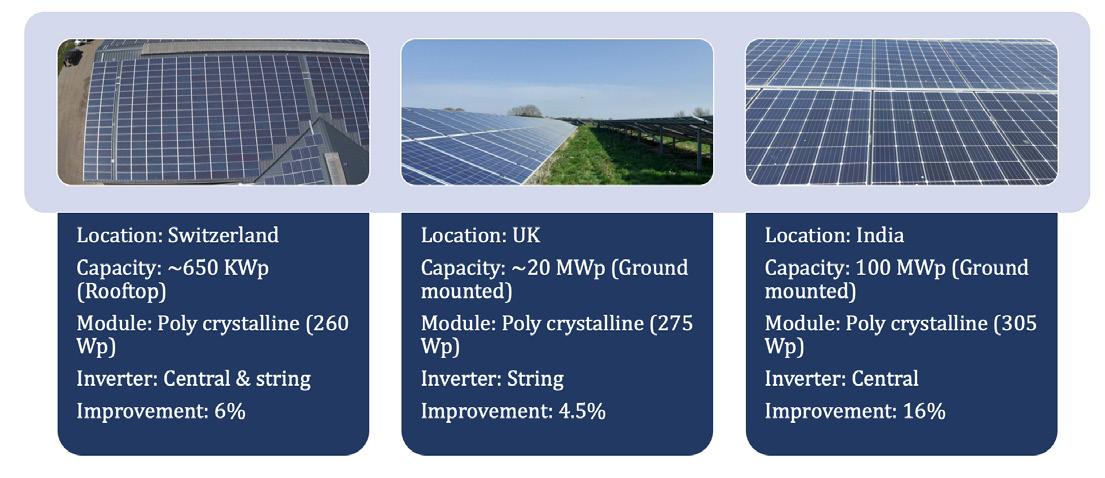
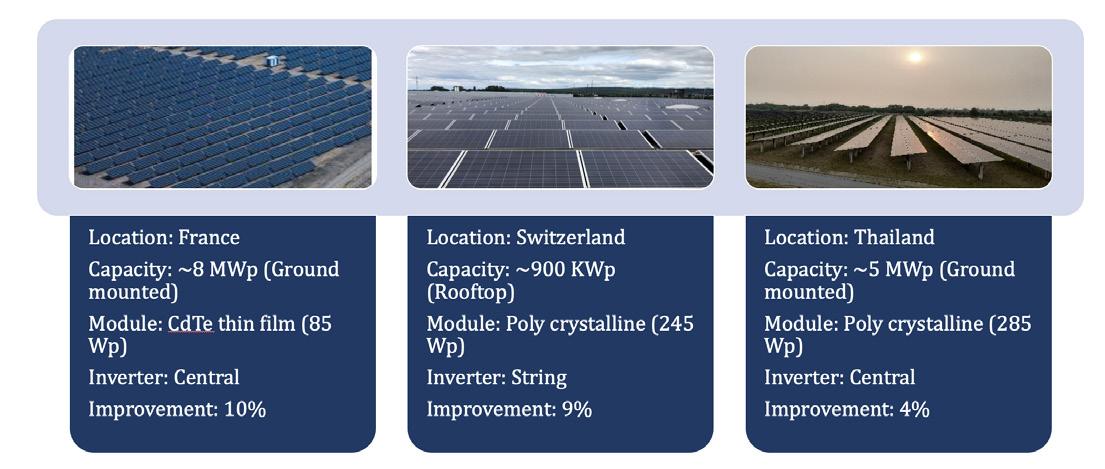
There are three main contributing factors for the loss of generation of an operational solar project (Figure 2).
Asset modernisation
There is an increasing demand of asset modernisation which has been noticed both in Europe and Asia and this is due to the ageing and distressed assets in the European and Asian market. This modernisation is becoming essential and necessary for asset owners to compensate loss of generations, and revamping or repowering the existing solar plants is becoming a general trend that has been observed in recent years.
For any revamping or repowering project, it is essential to maintain the existing compliances, such as technical, commercial, contractual, and interface with existing infrastructures. That is why a holistic approach is key to evaluating the following factors before reaching to a conclusion and decision-making for revamping or repowering:
> The mechanical infrastructure of the existing module mounting system.
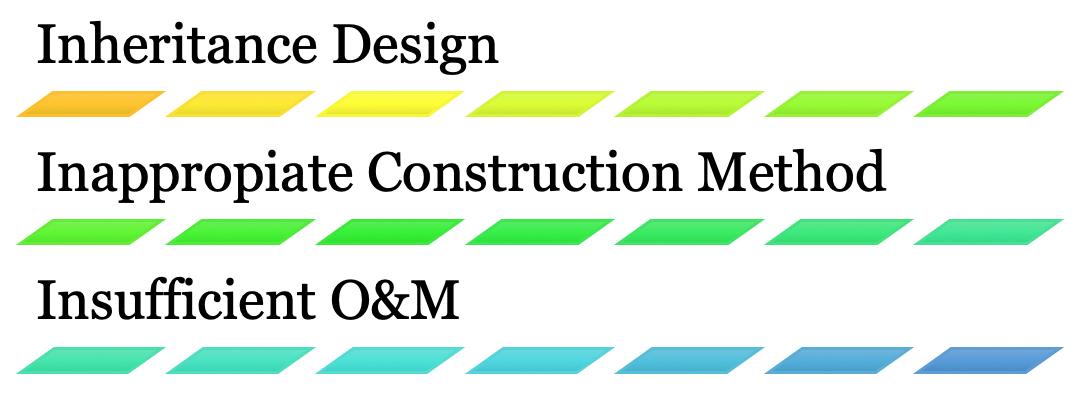
> Existing string configuration and the maximum system voltage level.
> Conditions of the existing modules, DC string cables, DC string combiner box.
> Condition of the inverter and transformer.
> MPPT voltage range of the existing inverter.
> Matching specifications of existing LV and HV equipment of the system.
> Minimise the change interface and onsite work.
> Inverter grid code compliance.
To assess the above factors, a detailed data analysis of the plant performance has proven to be the right approach from technical and commercial standpoints. PVAPM can conduct such assessment to pinpoint whether repowering of a plant is necessary, otherwise pragmatic solutions can be implemented to uplift the generation of a system.
Conclusion
The additional generation is not only bringing direct financial benefit but also creating climate impact by additional carbon dioxide offset. GreenEnco’s PVAPM is applicable to increase the energy efficiency of an existing solar system of any capacity and type (rooftop, commercial, industrial, and utility scale). Increased energy generation from an existing solar system contributes more green electricity to the overall energy mix, resulting in further decarbonisation. Moreover, it increases the value of the asset, which ultimately boosts investor’s confidence in green investment. Additional green investment will create more employment opportunities to help a circular economy and take a crucial step towards net zero targets.
Figure 2 . PVAPM identified reasons for loss of generation. Figure 1 . Photovoltaic asset performance management (PVAPM) steps.With a proven track record in enterprise asset digitization across the infrastructure landscape, vHive offers wind farm stakeholders to strategically digitize their assets, improve operational efficiency and minimize output degradation.
Its autonomous data acquisition and innovative Digital Twin platform offer a significant advantage, helping companies secure their position at the forefront of progress, and pioneering a new era of wind farms profitability.
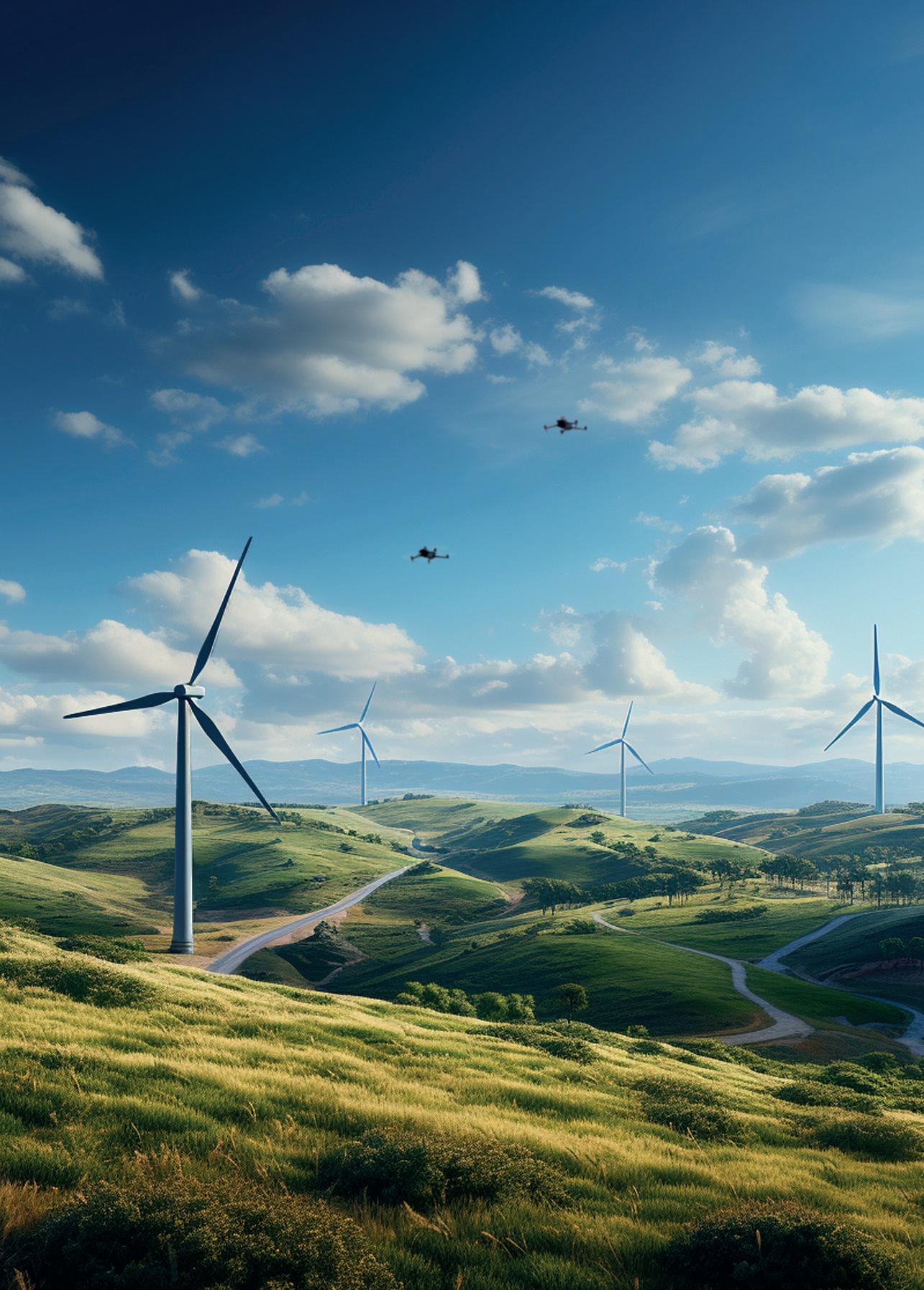
Piotr and Mikołaj
Menlo Electric, offer into how to meet targets 2025’ scenario.
o achieve the ambitious targets set in the ‘Net Zero by 2025’ scenario, a significant increase in renewable energy is needed and there are currently reasons to be hopeful about renewable sources. Existing sources are not sufficient to cover energy consumption and will be even less sufficient with the assumption of electrified transport and heating. Renewable energy is increasingly being built, but more installed capacity is not the only gating factor for the electrical grid.
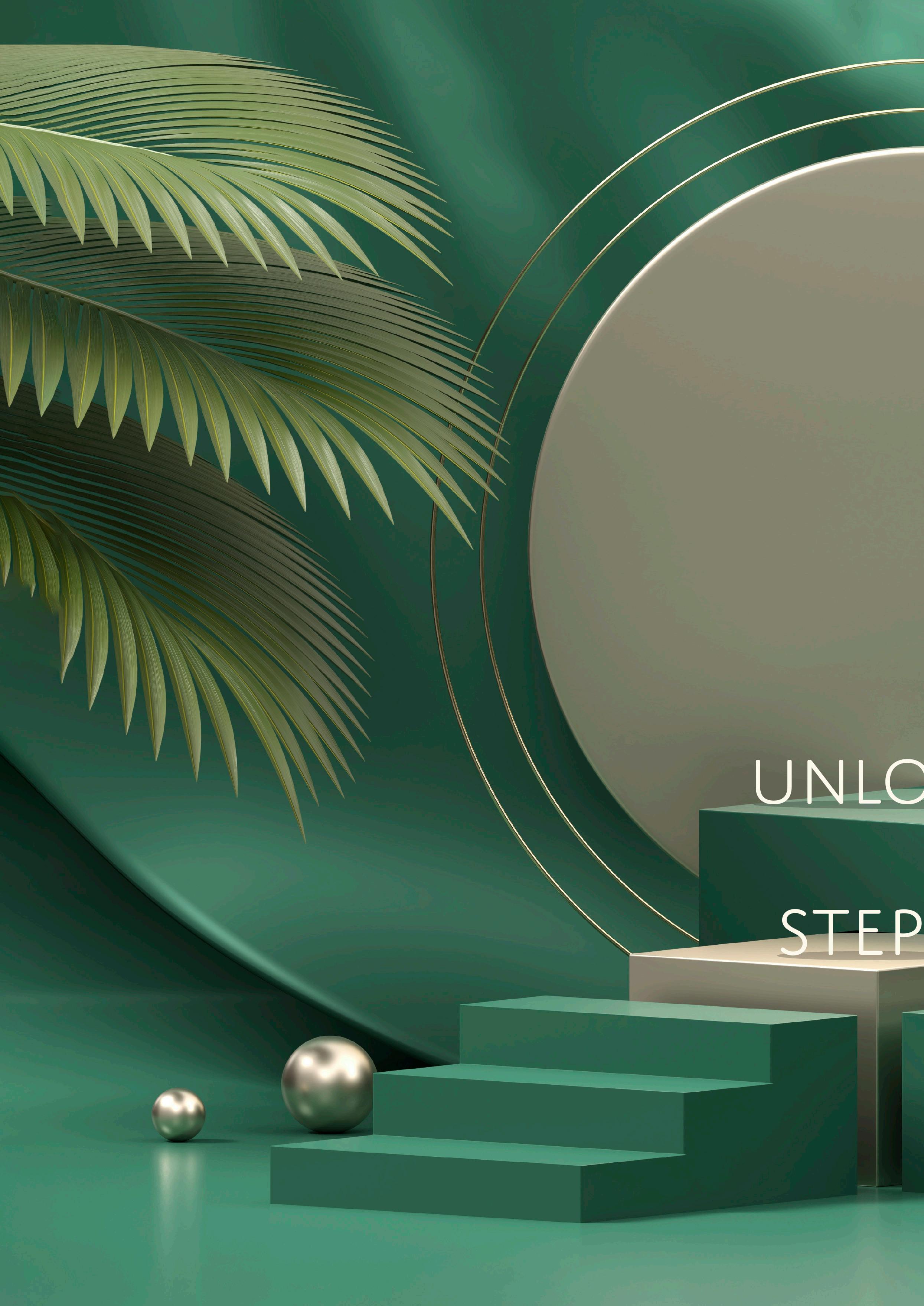
A brief insight into facts
Power plants with the largest installed capacity in Europe in 2022 were wind turbines (19 GW) and photovoltaic (PV) installations (41 GW). The renewable energy supplied to the grid is very weather-dependent, and the world’s energy
Loga
Mikołaj Konieczka, offer a perspective targets for a ‘net-zero by scenario.
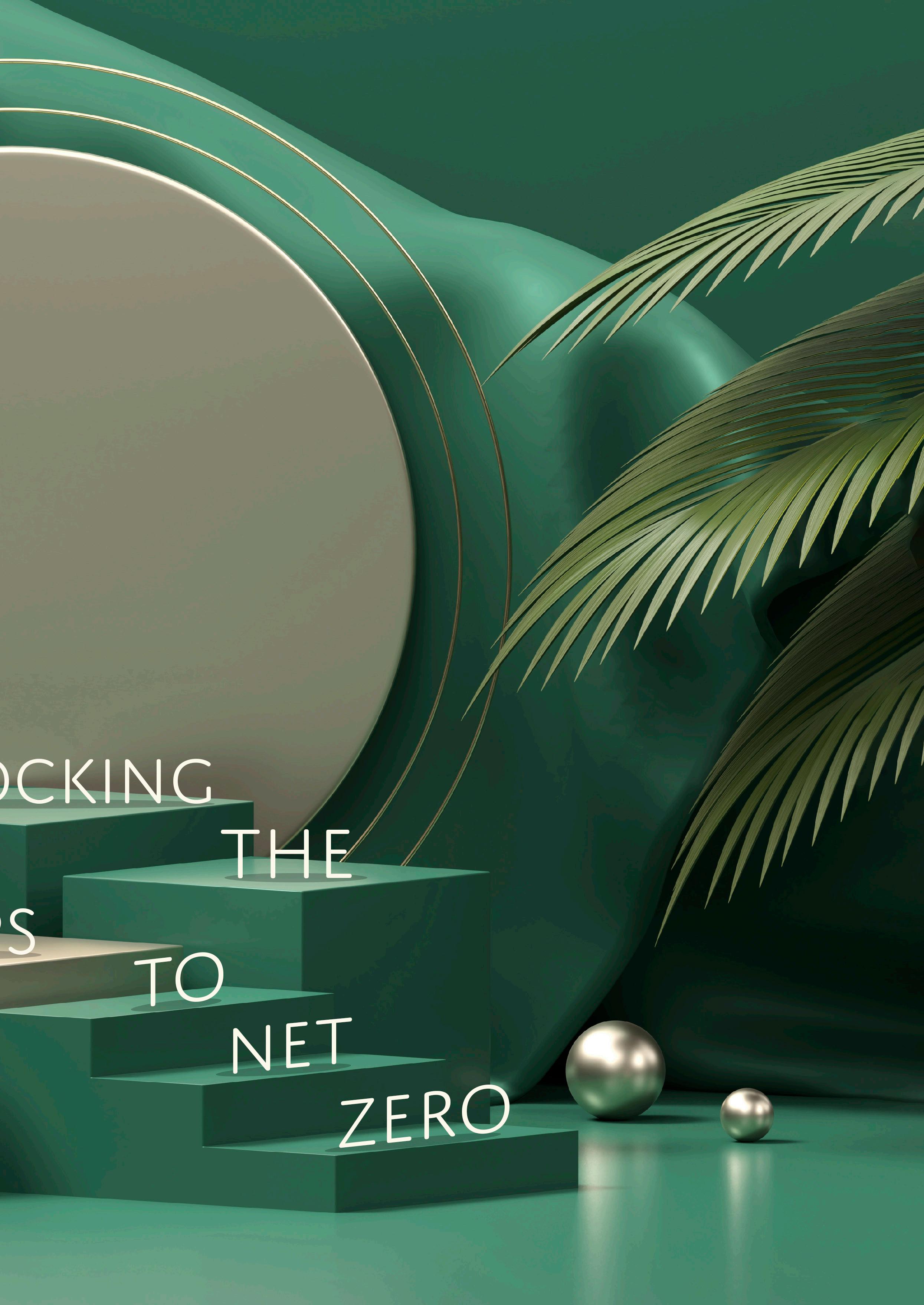
consumption is unfortunately not able to be adjusted to when the sun is shining, or the wind is blowing.
Today, the growth in installed capacity of renewables still pushes out the use of the oldest and least efficient power plants which emit the most carbon dioxide (CO 2 ) in many countries. However, it is important to note that situations where the generation from emission-free energy sources will exceed the full capacity of demand at any given time already occur, and will become more frequent in some countries. Fortunately, electricity production can be reduced, but this means that potential production opportunities are lost because possible production and consumption do not match. On 23 April 2023 in Poland, the state of threat to the security of the electricity supply was announced by the system operator. This forced the reduction of generation from renewable energy sources due to the system being too
inflexible to switch off the emission generation units in the short term and the inability to store energy.
Storing energy: a basic human need?
In order to avoid losing the surplus renewable energy generated and be able to use it when demand increases, energy needs to be stored for the long and short term. Electricity demand can be predicted using historical data, and generation predicted based on
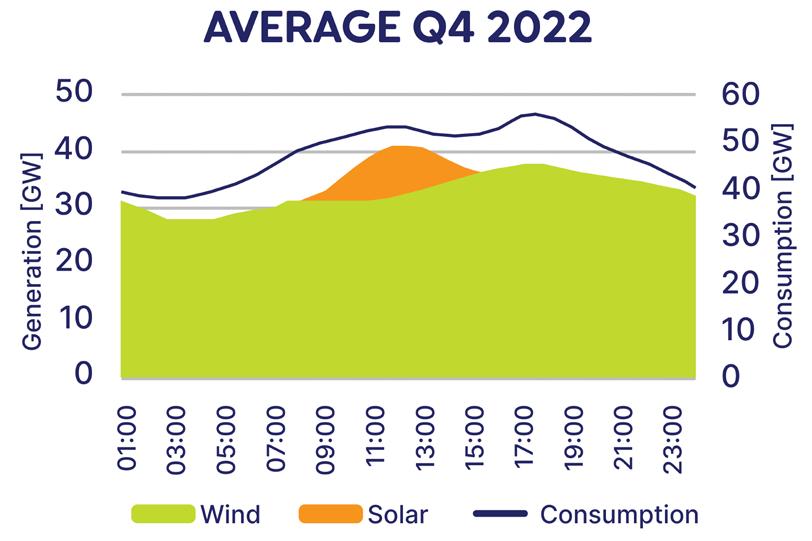
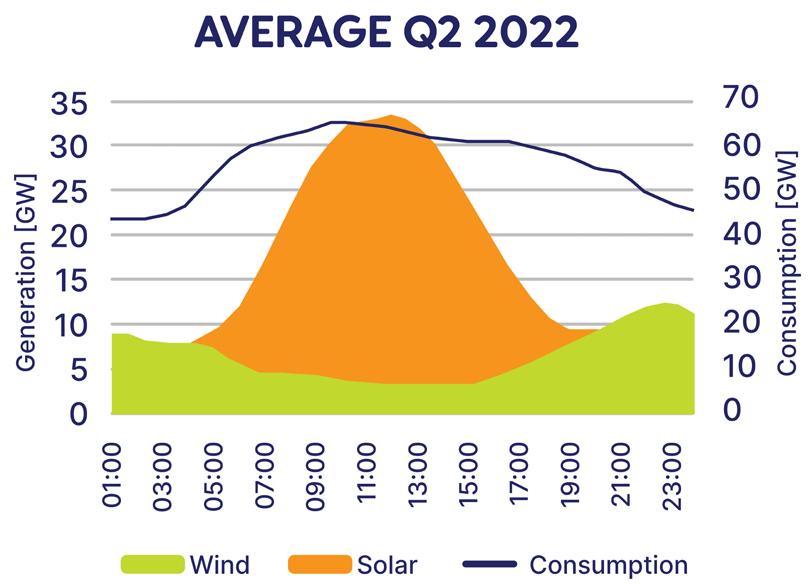
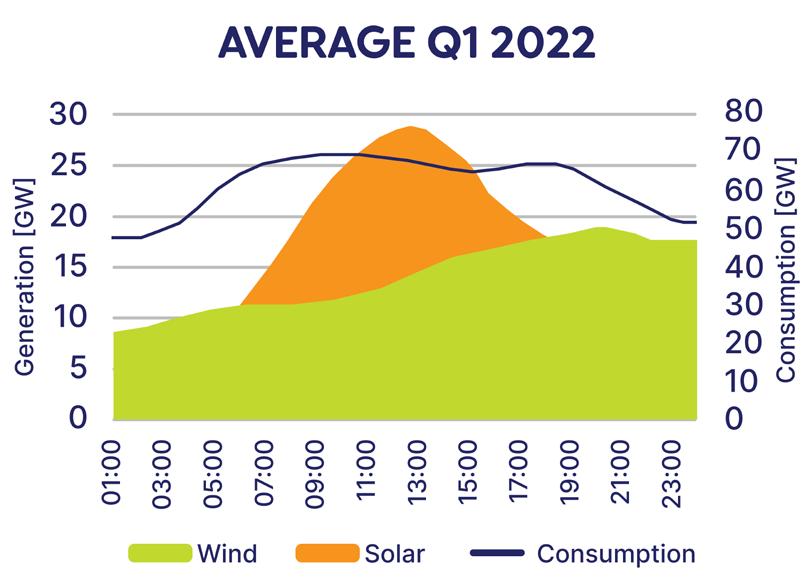
meteorological forecasts. There are many ways of storing energy, the choice depends on various factors –geographical location, needs, state of the grid.
Differences in energy demand are well reflected in the market price of energy at specific hours. At the time of the energy crisis in 2022, all records were broken for both energy prices and the price difference between specific hours on the same day. On 22 August 2022 in Poland, the highest energy price was more than five times the lowest in the same day. A large scale short-term (24 hr) energy storage would help reduce the maximum hourly price of electricity in times of peak energy demand from the times of day with the highest generation.
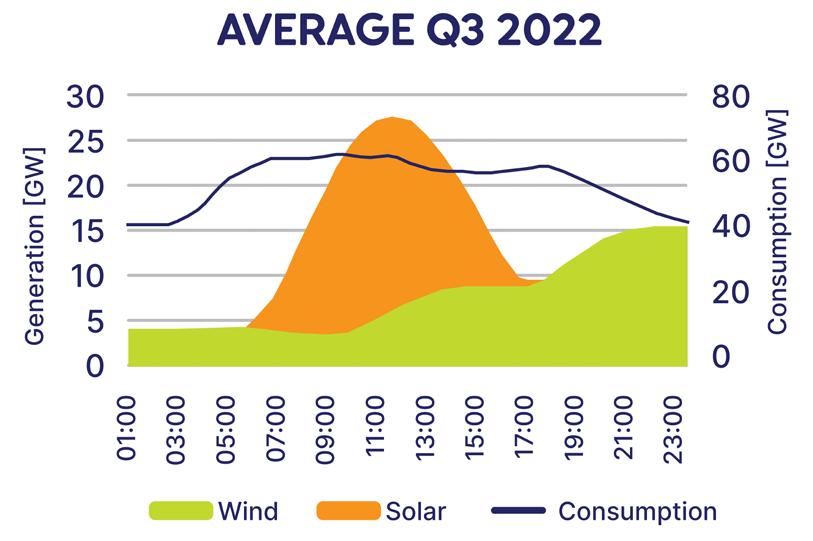
The fact is that the vast majority of energy storage is ensured by pumped storage power plants, which have been in operation for decades. The first pumped storage power plants were built in Italy and Switzerland in the 1890s. The system works quite simply and has therefore been in use for a long time; it consists of a turbopump system and two reservoirs, one of which is located at a greater height than the other. The excess energy generated powers the pumping of water from the lower reservoir to the upper reservoir, and the potential energy is stored. At times of increased demand, water from the upper reservoir is released into the lower reservoir, driving a turbine that generates electricity. This is a cheap and
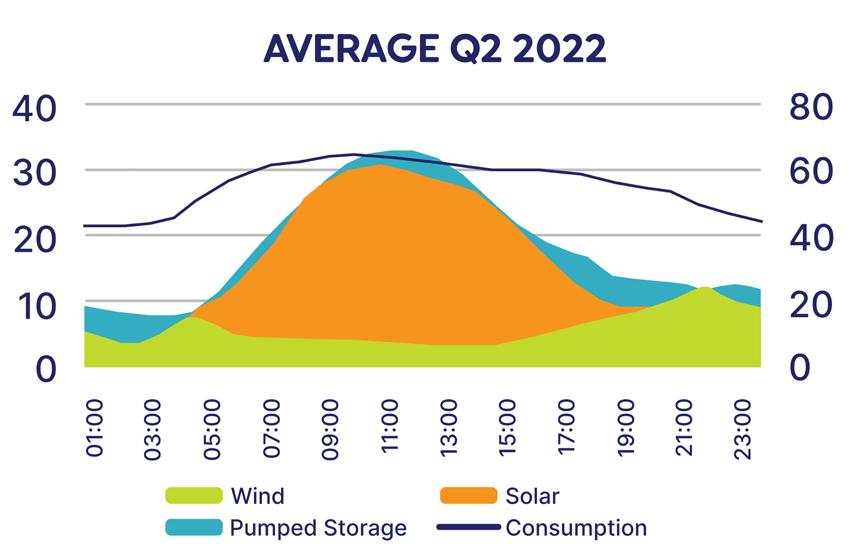
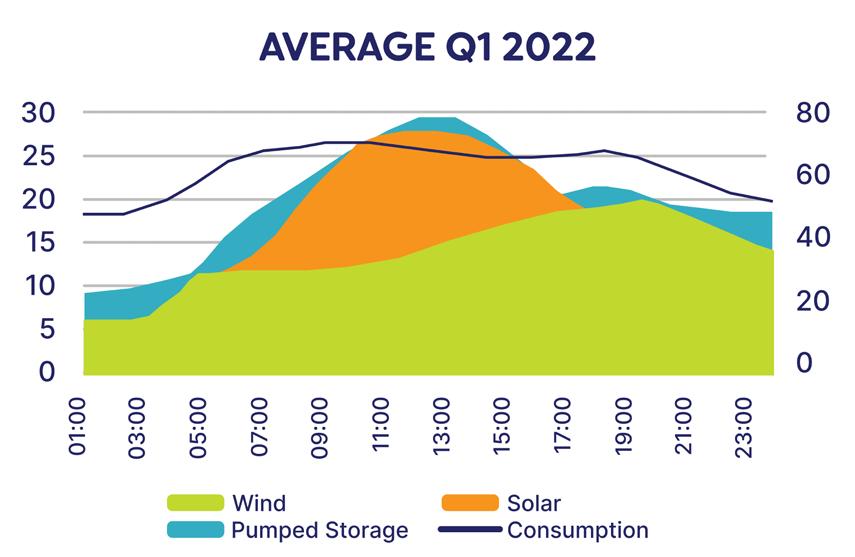
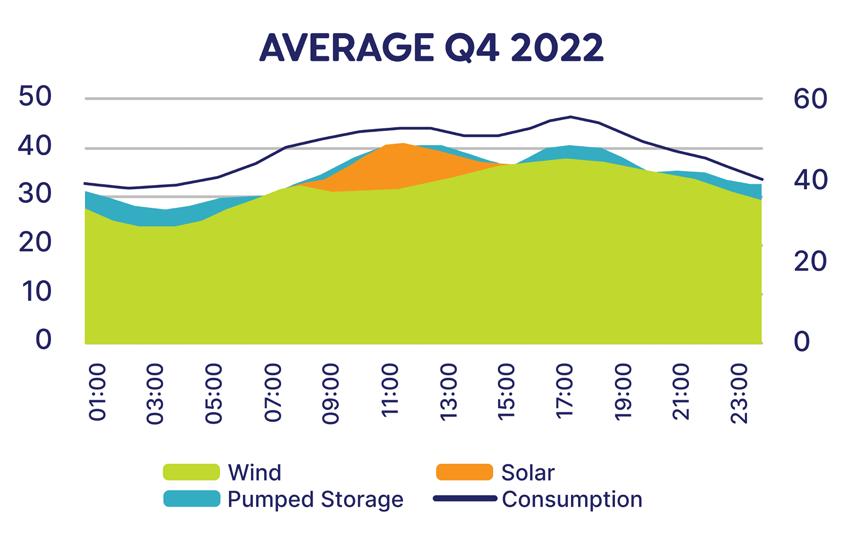
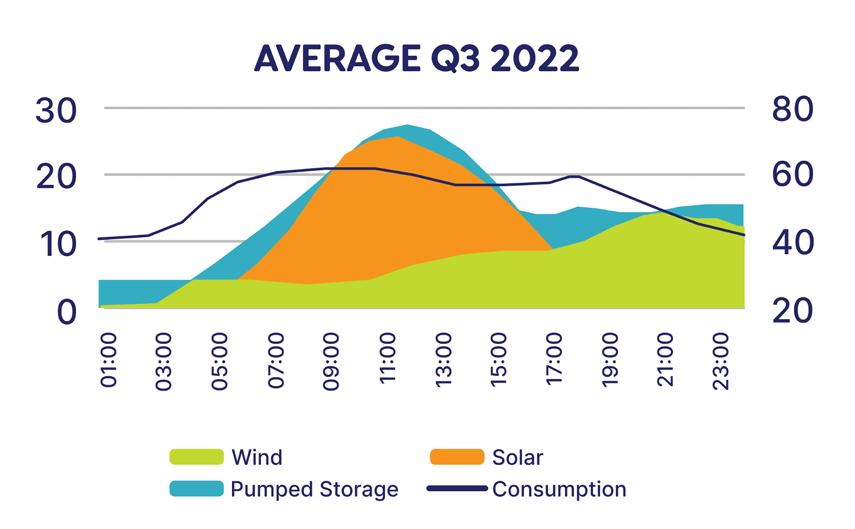
uncomplicated system, with the efficiency of such a plant estimated at 80%. It can also provide long-term storage. However, it requires suitable geographical conditions and a sufficient height difference between the reservoirs, which can limit the use of this system.
In this way, pumped storage power plants contribute to the stabilisation of the power system reducing production peaks.
One of the largest hydropower projects is under construction in northern Portugal. Tâmega will have a capacity of 1158 MW and 40 GWh, equivalent to an average daily electricity demand of 11 million people. The power plant complex, together with integrated wind turbines, is due to be commissioned in 2024.
The vast majority of energy storage capacity consists of pumped storage power plants, but last year saw a significant increase in the number of battery storage installations, whose capacity installed increased by 1.9 GW in 2022, representing over 70% increase in capacity compared to 2021.
Real examples to follow
The majority of storage is handled by lithium-ion batteries. The popularity of electrochemical batteries is due to the decreasing price per kWh over the years and developing technologies. They can also provide additional services, such as voltage and frequency control and reactive power compensation, which are important
functions for today’s power grid. An advantage of this technology is high efficiency, scalability, safety, and low environmental demands; therefore, the batteries are easy to implement on a large scale. However, due to the self-discharging process, they can only be used as short-term storage. Scarce raw materials should also be mentioned as they have limited deposits. Their lifetime is also strongly dependent on the ambient temperature and is manifested in a loss of capacity over a longer period of time.
Case study: Europe
The largest single battery storage facility in Europe, the Pillswood project in the UK, was launched in March 2023 and has a capacity of 196 MWh. The biggest advantage of this technology, however, is the simplicity
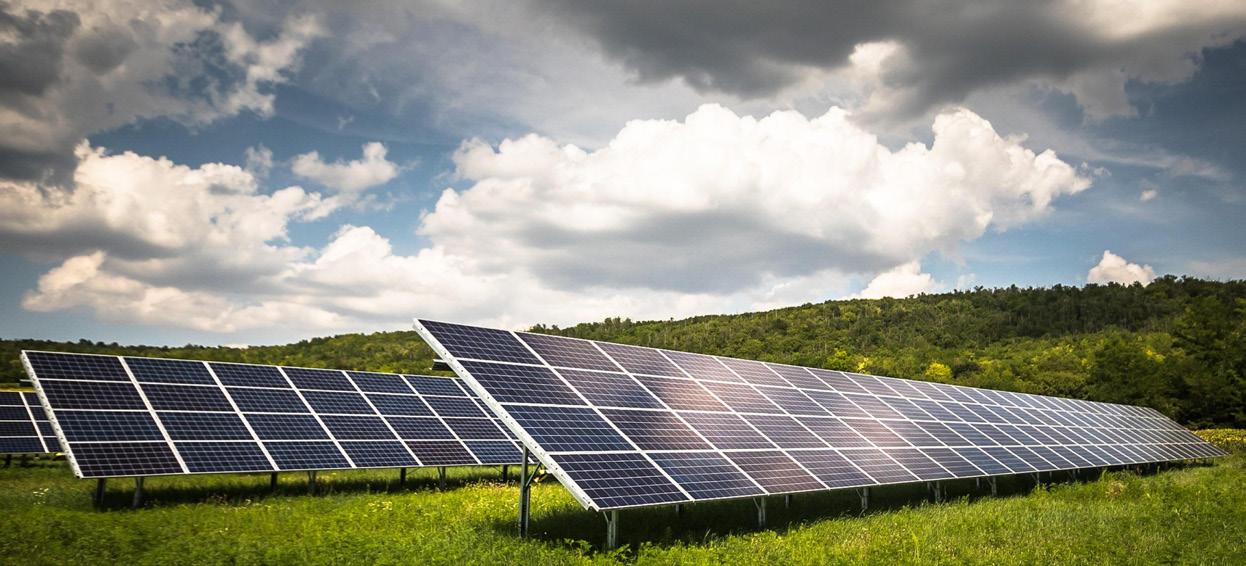
Global Hydrogen Review Online
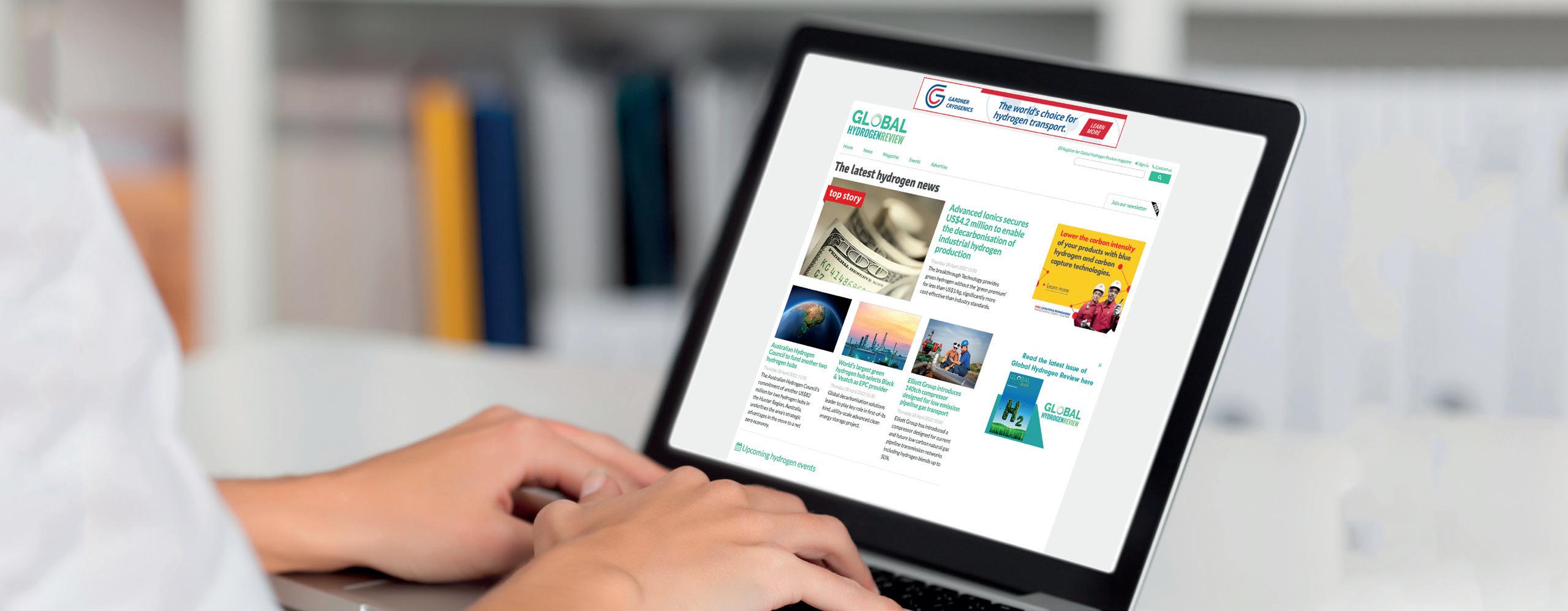
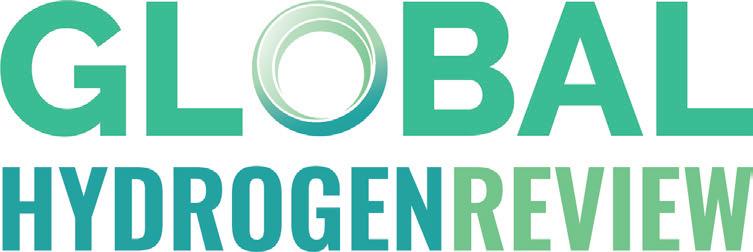
of mass implementation. Using the example of the Polish market, there are 1.2 million prosumers in Poland, representing more than 9 GW of installed capacity. An average output of an installation is 7.5 kW, and if each of them had a hybrid inverter and a capacity of 10 kWh, together they would form a large, distributed energy storage with an output of 9 GW and a capacity of 1.2 GWh.
Thanks to the relatively small size of lithium-ion battery-based storage, almost any prosumer can be transformed not only into an energy producer, but also into a local energy bank. This addresses the challenge of how renewable energy system (RES) energy production and demand meet. In a wider context, the operator could also use this storage at night to store the energy generated at the time of low global demand to cover the peak energy demand requirements.
Case study: China
Flow batteries are also becoming increasingly popular and may play a significant role in the future power system. In this storage system, the electrochemical components that combine in the battery are collected in two separate tanks divided by a membrane. They are only 70% efficient, but they are much cheaper than lithium-ion batteries, and some of the components used, such as vanadium, do not age, so they can be used for many years with virtually no loss of efficiency or capacity. There is increasing investment in these batteries in China, the largest being Redox Flow Battery Storage, which was launched in May 2022 in Dalian and has a capacity of 100 MW and 400 MWh.
What about other technologies?
However, there are other forms of energy storage that are not yet widespread and do not currently represent a large share of the overall storage market but could significantly contribute to the growth of the sector in terms of time and technology development. This is illustrated by a number of investments and improvements in other technologies that are looking to complement or provide an alternative to the current ones.
Compressed air storage
Compressed air storage has been a well-known technology for many years. The first such facility was built in Huntorf, Germany, in 1978 and is still in operation today. The mechanism is that in times of surplus electricity, a compressor is started, and the compressed air is cooled at the compressor outlet and fed into a salt chamber or other underground storage tanks. The compressed air remains there until the power demand is increased where it is then discharged from the tank, heated, and directed into the turbine of the power generator.
Mines in impermeable rock or underground pipelines will be used as tanks, so this type of storage can be built on the infrastructure of phased-out power plants.
One of the largest projects of this type is the Silver City Energy Storage Centre, to be built in Australia,
which will create a 200 MW energy storage facility with a capacity of 1.6 GWh. It uses the A-CAES technology, an improved version of which allows heat generated from compressed air to be stored in a heat storage tank and used to heat the air before it is fed into a turbine.
Gravity storage
A newer technology that has just been discovering its potential is gravity storage. Vault Energy has patented a solution that resembles towers or big blocks. When production is high, the concrete blocks rise to a height and when energy demand is higher, the blocks descend, producing electricity. This is a rather innovative approach based on the mechanism of a pumped storage power plant where electricity is converted into potential energy. A 25 MW, 100 MWh storage project is currently under construction in Rudong, China.
Heat storage
Another way to ensure that the surplus energy is not wasted is heat storage, where the thermal energy produced from the surplus RES is accumulated in a large storage tank, thermally insulated from the surrounding environment. The heat is then fed into the district heating network and used as useful heat in the evening by end users. A 56 million thermal storage plant is being built in Berlin, Germany, to supply 200 MW of thermal energy to the nearby urban grid. In the meantime, the first sand heat storage with a capacity of 100 kW and 8 MWh has been developed in Finland. It is a seasonal heat storage that can be filled and used during the months when heat demand is highest. This is an interesting solution because it can retain stored energy for up to eight months.
Hydrogen in a main role?
A very important technology to consider is energy storage in the form of hydrogen. When there is a surplus of electricity, hydrogen can be produced in an electrolyser and when demand increases, electricity can be produced in a hydrogen fuel cell. There are still some storage difficulties with hydrogen due to its high pressure, which also affects transport. However, hydrogen can not only provide us with electricity, but can also be used as a fuel in heavy industry and air transport due to its high energy density. Hydrogen investments are being made globally. The largest project announced is to produce 3.6 million t of green hydrogen in 2030 through 95 GW of PV installations and 67 GW of electrolysers in an integrated system stretching from Spain through France to Germany.
Conclusion
There are many ways of storing energy, and with the transition to a carbon-free economy, we need to invest more and more in energy storage so that people can continue to use electricity without interruption. Which technologies will find the greatest uptake will depend both on the energy needs of potential sites and the speed of development of the technologies.
srt - 250 sl placer tractor
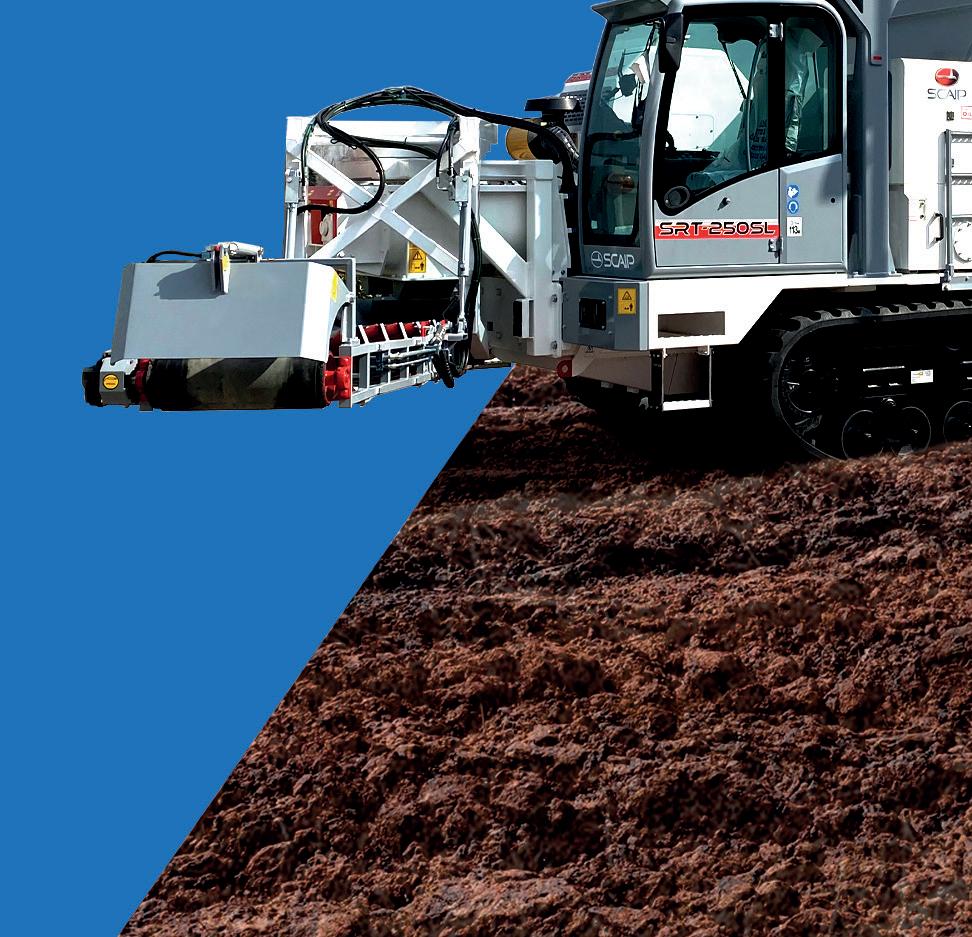
INNOVATIVE AND CUSTOMIZED SOLUTIONS FOR EVERY CHALLENGE INNOVATIVE AND CUSTOMIZED SOLUTIONS FOR EVERY CHALLENGE since 1958
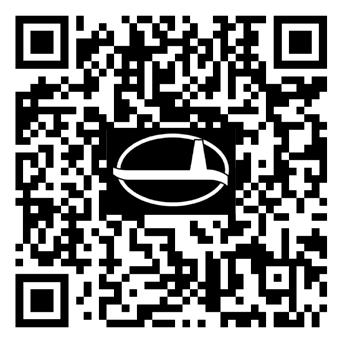
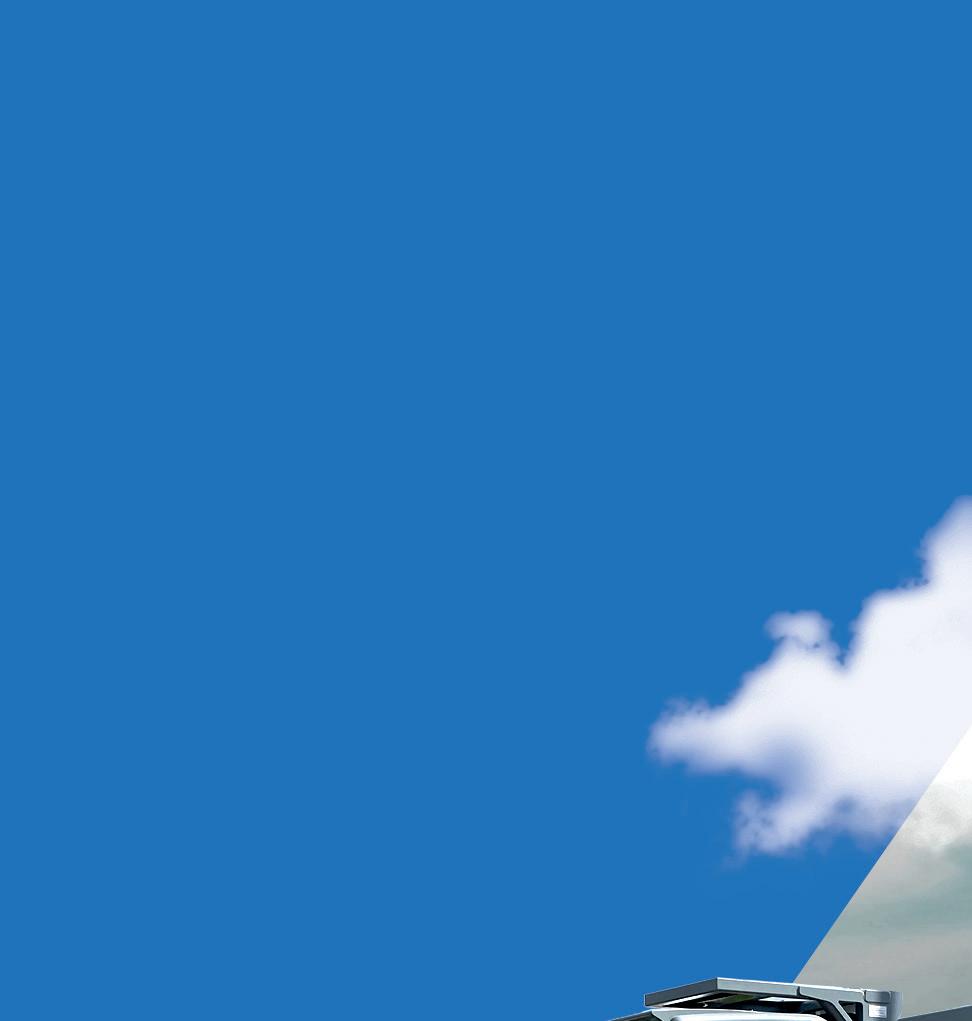
HYDROSTATIC TRANSMISSIONS
max max
produ ction capa city
FOLDABLE CONVEYOR HIGH TRACTION AND STABILITY REMOTE CONTROLLED AVAILABLE
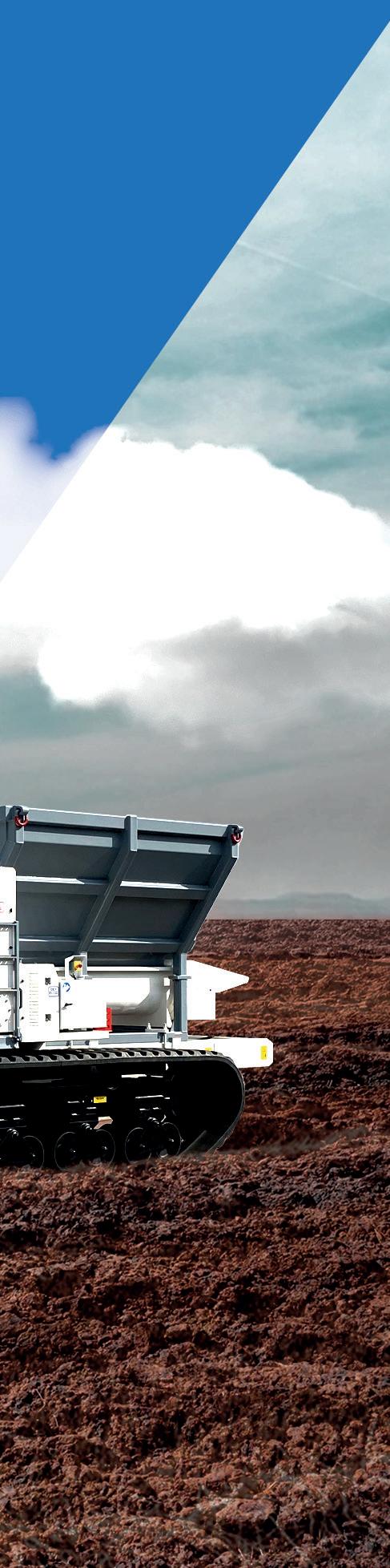
165 yd3/h 9.7 yd 3
Parma, Italy
Sharon Imber, CMO, vHive, focuses on the profound impact of wind turbine farms digitisation and how it is reshaping the entire value chain of wind energy, ushering in a new era of efficiency, sustainability, and profitability.
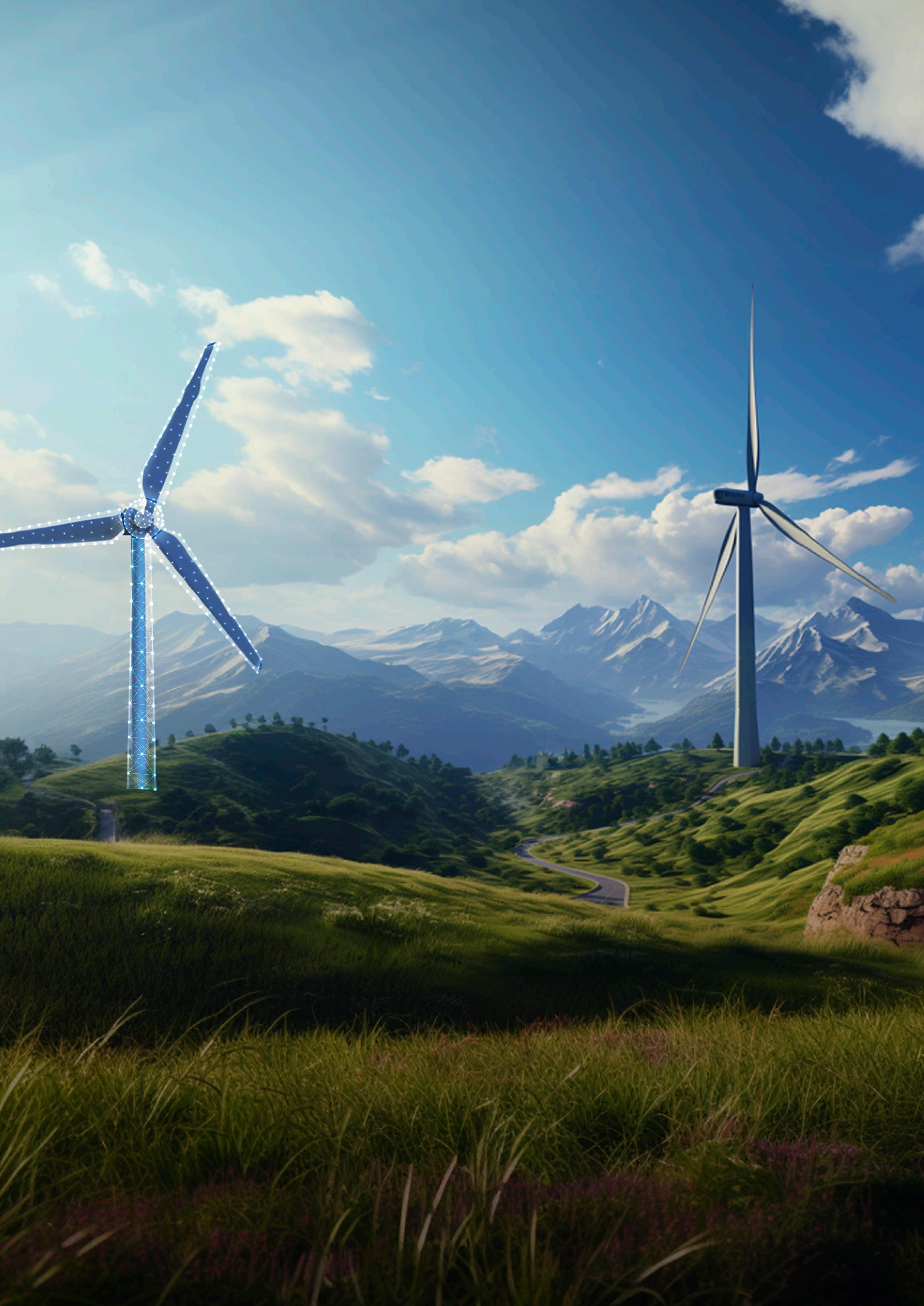
he revolution in wind turbine farm digitisation is no longer a distant vision; it is a reality that is transforming the energy industry as one knows it. Over the years, wind energy has evolved from an experimental alternative to a mainstream source of power, and the increasing adoption of digital technologies in the wind turbine industry has been a key driver of this transformation.
Now, key stakeholders in this sector can already gain the tangible benefits of digitisation, differentiating industry leaders from those who may lag behind. Embracing this technological shift has become a crucial competitive advantage in the pursuit of sustainable energy solutions.
Main failures of traditional methods for turbine inspection
The main disadvantages of using tradition methods to inspect wind turbines are:
> Manual approach: Conventional inspection methods are time-consuming and demand highly skilled personnel to climb the turbines physically.
> Climbing: Climbing the towering wind turbines for inspection poses significant safety risks, as well as resulting in downtime.
> Ground-based zoom photo capture: Using ground-based cameras to capture detailed images of the turbines is limited in its scope and fails to provide a comprehensive view.
What is the big leap? Unlocking the potential of wind turbine digitisation: Paving the way to higher profitability
The wind turbine industry is on the cusp of a revolutionary transformation driven by digitisation, a leap that promises to redefine its future trajectory.
Today’s innovative technology provides a turnkey solution that facilitates scalable and rapid inspections without the need to rotate each blade independently. By harnessing low-cost, off-the-shelf hardware empowered by autonomous multi-drone technology, downtime is significantly reduced. The elimination of the need for professional drone pilots, coupled with enhanced accuracy through artificial intelligence (AI)-based analytics, enables wind farm operators to achieve consistent and comprehensive data gathering for repeatable and comparable analysis.
This combination of autonomous turbine capture technology and AI-driven analytics represents the gospel of today’s wind turbine industry, delivering an array of benefits:
> Revenue optimisation.
> Decreased OPEX.
> Improved productivity.
> Extended lifetime of assets.
> Enhanced safety measures.
The key to realising these advantages lies in creating virtual representations of physical turbines, known as digital twins. Achieving this feat relies on the precise and rapid capture of wind turbines at scale, which significantly reduces downtime and improves overall operational efficiency.
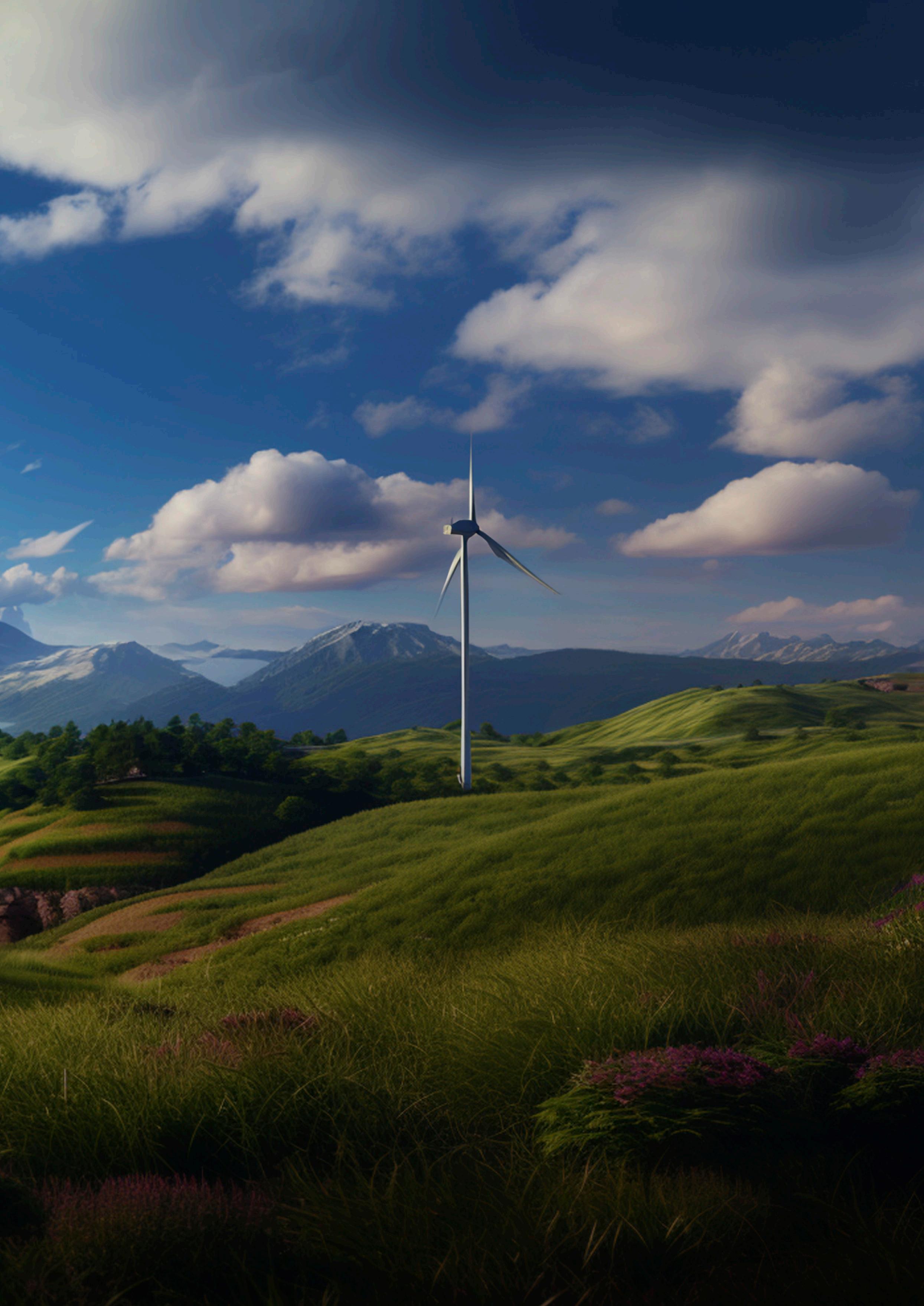
How does wind turbine digitisation bring about these benefits?
The core components of this transformative process include:
> Cost-effectiveness: By leveraging readily available off-the-shelf hardware, wind farm operators can adopt digitisation at a fraction of the cost compared to traditional methods.
> Consistent data: Digital twins ensure uniformity and consistency in data gathering, providing operators
with reliable, non-human dependent insights for decision-making.
> Pilot-independent: Digitisation eliminates the reliance on professional drone pilots, making operations more accessible and cost-effective.
> Speed: Gone are the days of manually rotating blades for inspections. With digitisation, inspections are swift, efficient, and require minimal physical blade rotation.
> Comprehensive Insights: The wealth of data captured through digitisation enables a comprehensive understanding of turbine health and performance.
> Repeatable and comparable: The digitisation process allows for standardised data collection, facilitating repeatable and comparable analyses across turbine surveys over time.
> Multi-drone technology: The use of multiple drones working autonomously reduces downtime and enhances the overall inspection process.
> AI integration: The captured data is harnessed by AI algorithms, enabling the utilisation of cutting-edge analytics and machine learning for valuable insights.
> Flexible operational model: Digitisation knows no bounds; it can be implemented anywhere in the world, utilising existing field operators or through turnkey solutions. Off-the-shelf drones can be deployed, while safety measures ensure that climbing is only necessary for essential maintenance.
> Scalability: The digitisation of wind turbines paves the way for seamless scalability, allowing operators to efficiently expand their operations without compromising on inspection accuracy.
Auto-discovery technology plays a crucial role in this digital revolution. This technology streamlines various aspects of wind turbine management, such as:
> Wind turbine height detection: Drones equipped with auto-discovery technology can accurately detect wind turbine height, aiding in planning and analysis.
> Blade positions/orientation detection: The auto-discovery technology automatically detects the precise positions and orientations of the blades, optimising inspection efficiency.
> Blade capture without rotation: The cutting-edge technology enables the capture of blade data without the need for manual rotation, expediting the process.
Digital twins
At the heart of the digitisation revolution lies the concept of wind turbine digital twins. These virtual representations offer a comprehensive and centralised visualisation platform for all stakeholders.
The key features of wind turbine digital twins include:
> Cloud-based visualisation: A platform that operates on cloud infrastructure, provides easy access to specific data on a web browser, from anywhere, at any time.
> Geo-located high-resolution Imagery: Digital twins incorporate detailed imagery, geographically
located, and offer a holistic view of wind turbines and their surroundings.
> One source of truth: Wind turbine digital twins serve as a single source of truth, fostering seamless collaboration among all stakeholders in the value chain.
> Automated tagging and analytics, fault identification and severity classification: Digital twins are equipped with AI-driven analytics capable of automating the tagging of turbine assets and identifying potential faults, enabling timely interventions for improved turbine performance.
The power of digital twins lies in their ability to detect a myriad of defects, such as blade collar misalignment, erosion, surface cracks, open bond lines, paint peeling off, oil leakage, and more. By harnessing these insights, operators can proactively address issues, prolonging the lifespan and optimising the performance of wind turbine farms.
The annotation and reporting capabilities play a pivotal role in maintenance analysis. Wind turbine inspections become a seamless process, enabling easy annotation of images and comprehensive documentation for field personnel. Generating inspection reports is no longer a burdensome task; instead, it becomes a crucial reference point for prioritising repairs and maintenance efforts.
The value chain of wind turbine digitisation extends beyond mere inspections, encompassing various aspects of wind farm operations:
> Wind turbine and blade inspection: Digital twins empower operators to conduct thorough inspections, identifying potential issues and streamlining maintenance efforts.
> Damage assessment: Timely identification and resolution of faults help prevent costly damages, optimising turbine performance.
> Operational performance optimisation: Real-time data analytics facilitate data-driven decision-making, optimising turbine performance.
> Supporting merger and acquisition (M&A) deals: Offering detailed insights into wind farms’ health, digital twins aid due diligence and enhance decision-making for potential buyers and investors during M&A transactions.
Summary
The wind turbine industry’s digital revolution has arrived, and it is no longer a matter of if, but who, will seize the innovative technologies to gain a competitive edge. With autonomous data acquisition and the transformative digital twin technology, wind farm operators can strategically digitise their assets, enhance operational efficiency, and safeguard their profits. Embracing the digital transformation is the defining factor that will determine industry leaders in the wind turbine sector. Those who adopt these innovative technologies will secure their position at the forefront of progress, shaping successful business models that will pioneer a new era of profitability.
RE+ RENEWING WHAT’S POSSIBLE
SEPTEMBER 11 - 14, 2023
LAS VEGAS, NV
DISCOVER THE LATEST GLOBAL MARKET OPPORTUNITIES
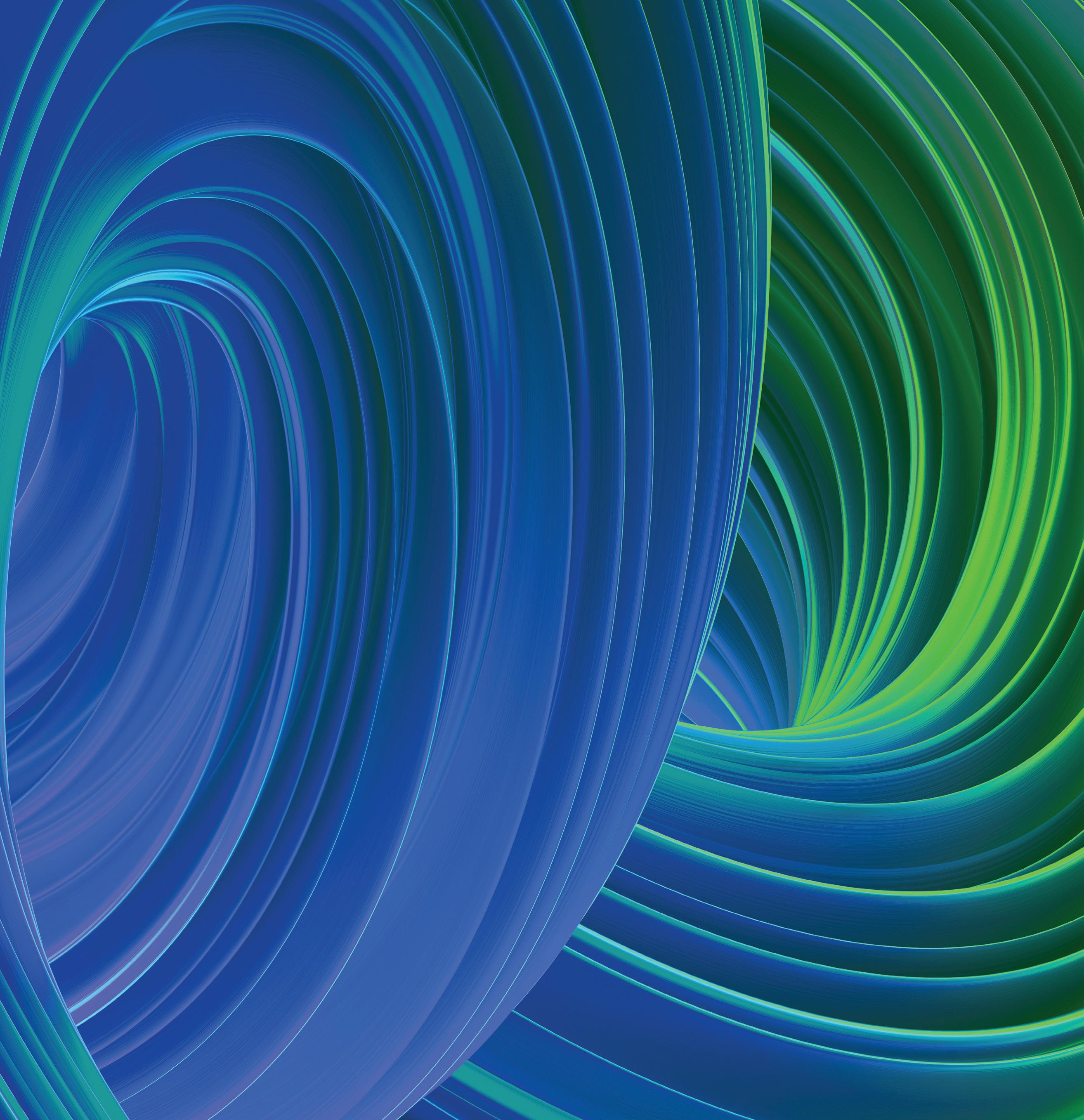
Is your company looking to reach the international clean energy market? RE+ 2023 offers many opportunities for attendees to engage with international leaders, hear about opportunities and challenges, and learn how to enter global markets such as Africa, Europe, Canada, Mexico, the Caribbean, and more!
Learn more and register today!
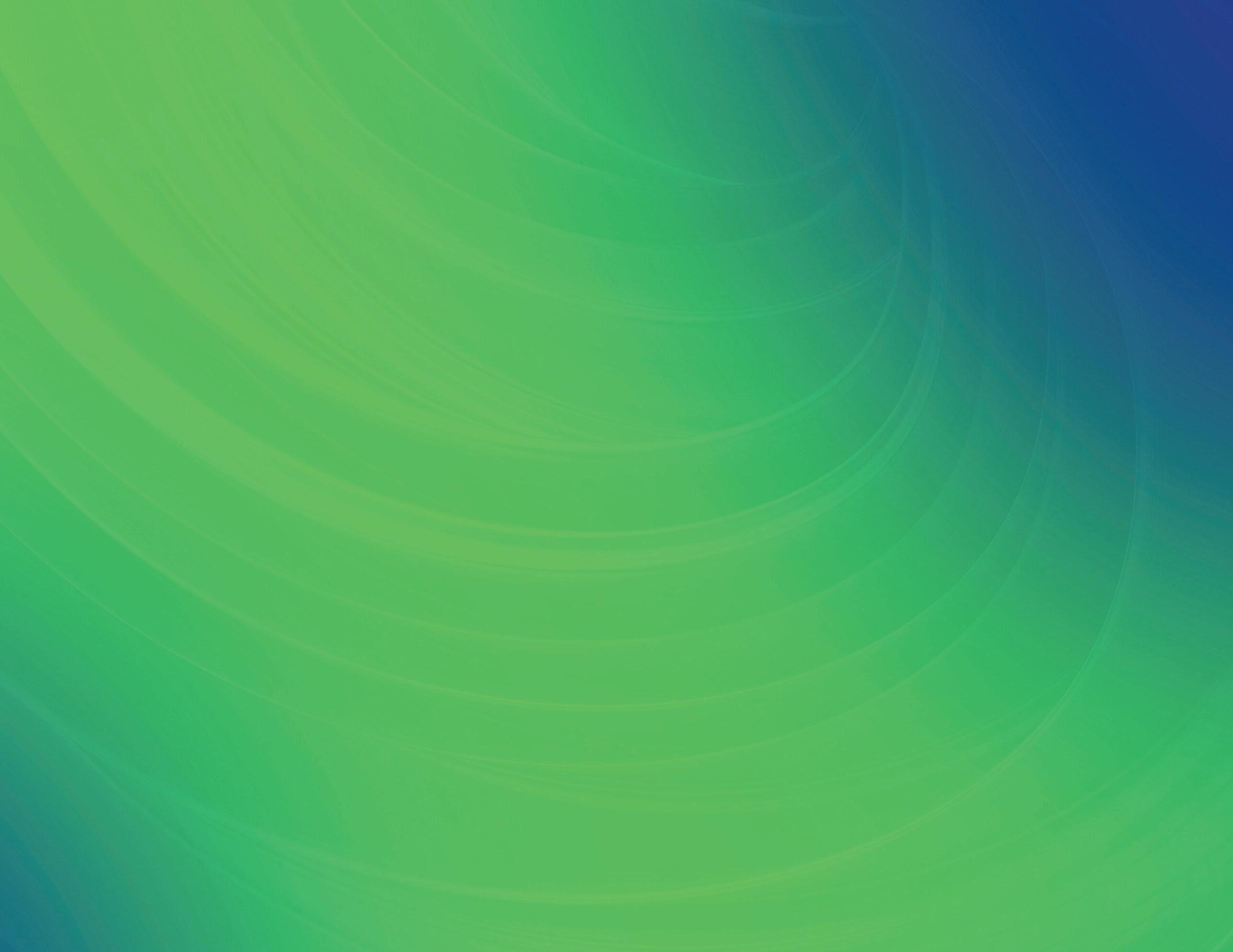
As the world drives to meet ambitious sustainability targets, the global energy storage market continues to grow rapidly.
Globally, it is estimated that 387 GW/1143 GWh of energy storage capacity will be added from 2022 to 20301 – that is more than Japan’s entire 2020 power generation capacity. The US and China are still leading the way, but Europe is set for a significant ramp-up in capacity as a result of the current energy crisis.
However, with more energy being stored – and more batteries being used to higher capacities – there are significant fire risks, and many customers are seeking reassurance on fire safety as the market continues to expand.2
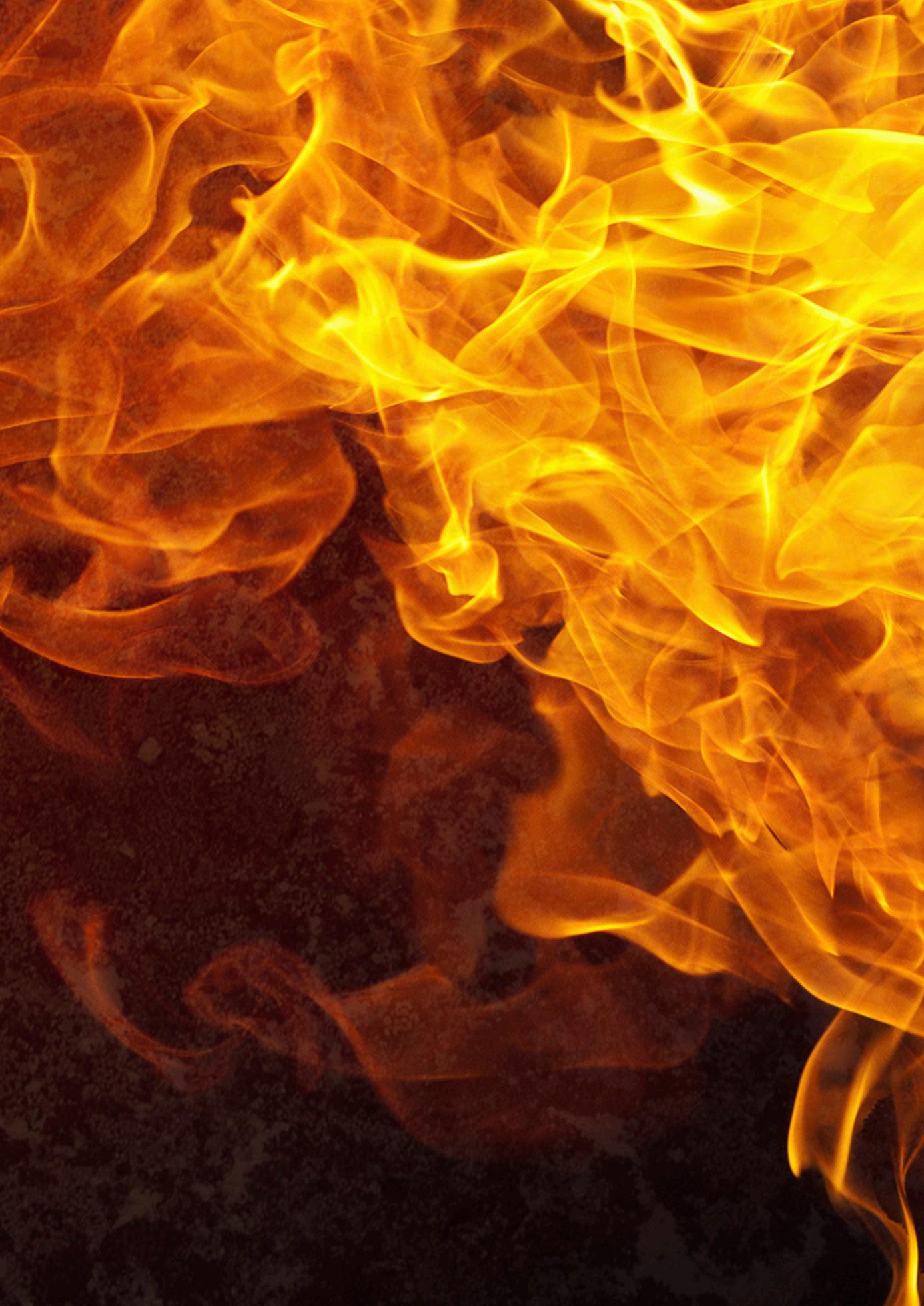
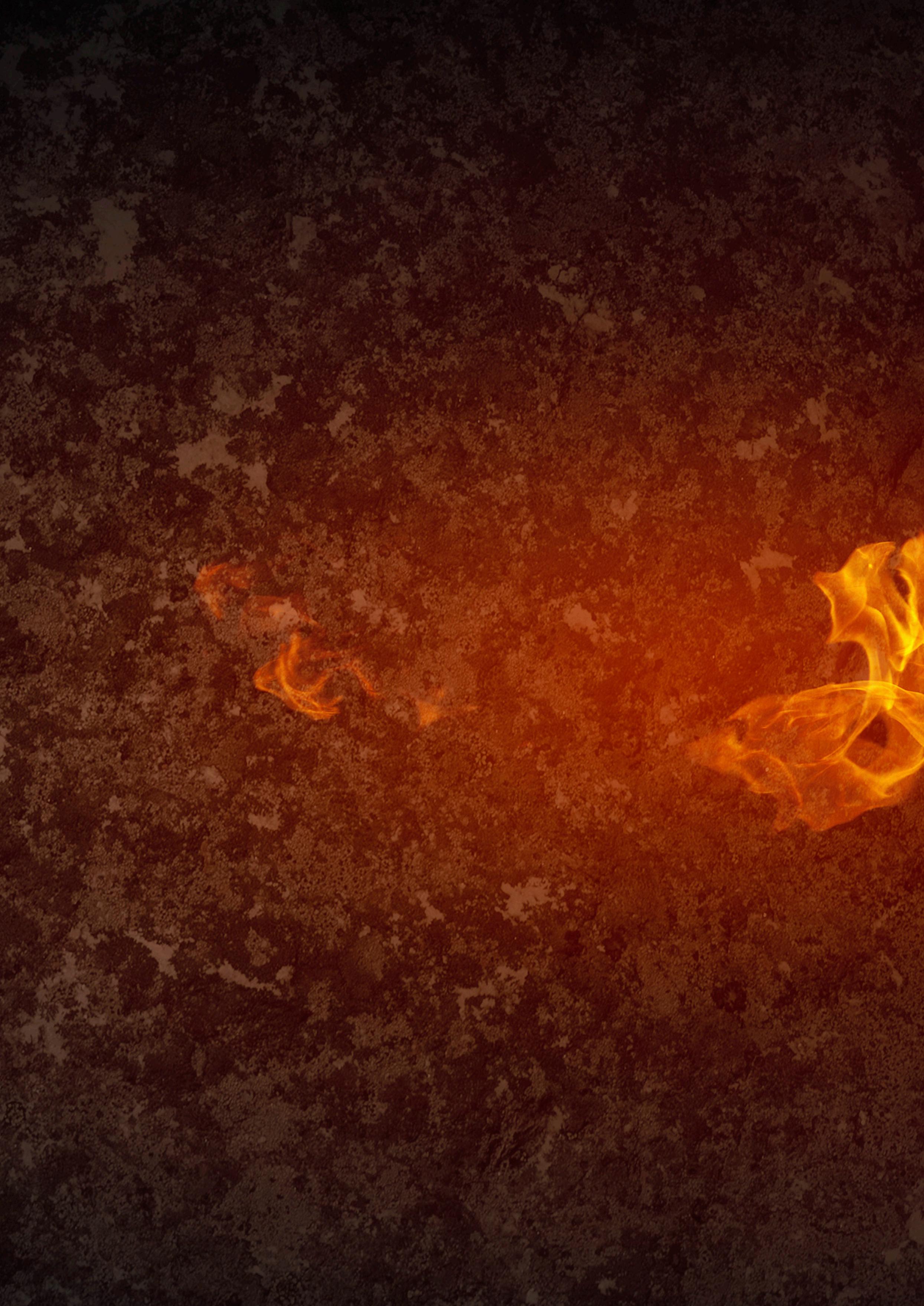
A changing energy landscape
With a growing volume of conversations around carbon emissions ignited in the wake of extensive discussions at COP and other environmental conferences, governments around the world are continuing to push ambitious sustainability agendas.
A key aspect of these agendas is focused on the transition to a future of net zero emissions by 2050. To achieve this, it is estimated that the annual clean energy investment worldwide will need to more than triple (to approximately US$4 trillion) before 2030.3 Inevitably, this will put immense pressure on energy storage around the world, and, as a result, there has been unprecedented increasing demand to boost the capacity for energy storage globally.
With more people and organisations looking for sustainable energy sources, the global reliance on electricity is skyrocketing.4 This ‘electrification’ of vehicles, machinery, and other equipment powering everyday business operations and domestic homes, when sourced from renewables, is undoubtedly having a positive impact on global carbon emissions. However, it is also seeing an increase in the number of batteries – predominately lithium-ion (li-ion) batteries – needed to store and distribute this energy. That comes with its own fire safety risks – which are becoming evident through recent battery fires at energy storage
systems around the world.5 As more batteries are introduced, the frequency of these events will only increase too.
Unpicking the risks
The primary risk presented by an increase in li-ion batteries to boost energy storage capacity is thermal runaway.
Thermal runaway is an extremely dangerous process, which occurs when a battery’s cells malfunction. This can happen for a number of reasons, such as physical damage to the battery, overheating, overcharging, mechanical failure, or overvoltage, for example. In thermal runaway, the battery will experience rapid temperature increases, which in turn will cause it to release additional energy, which further increases temperatures to potentially dangerous levels.
If these temperature spikes are not controlled quickly, using effective fire detection and suppression methods, thermal runaway will progress – where it is likely to cause fires, which will spread across multiple battery cells and to other nearby batteries, toxic gas emissions, and potentially also large explosions. Those toxic gas emissions can include carbon monoxide, methane, and also hydrogen fluoride, which if it reacts with water or moisture in the air creates an acid presence, creating additional risks in the surrounding area.
If batteries are stored in close proximity to one another, as is often the case in energy storage facilities due to space and capacity constraints, this can lead to a much bigger issue, as risks will spread from one battery to the next, amplifying the safety consequences.
A distinct lack of regulation
Disappointingly, there is a distinct lack of regulations governing the safety of energy storage facilities. As the global push for
Kristoffer Eldin, Dafo Energy Storage risks associated with energy storage, control them effectively.
Storage Protection, Sweden, examines the various storage, namely fire, and the measures needed to
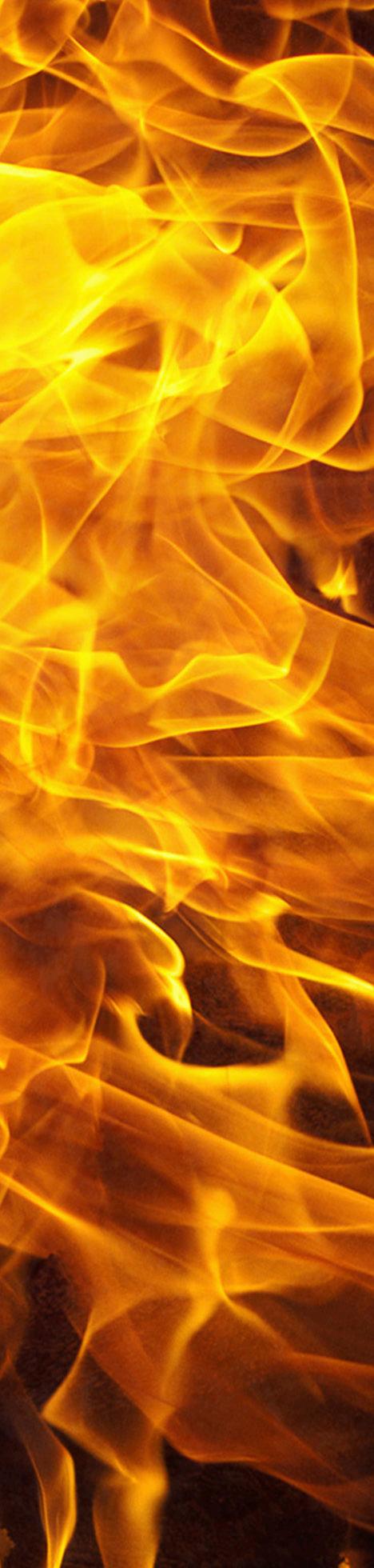
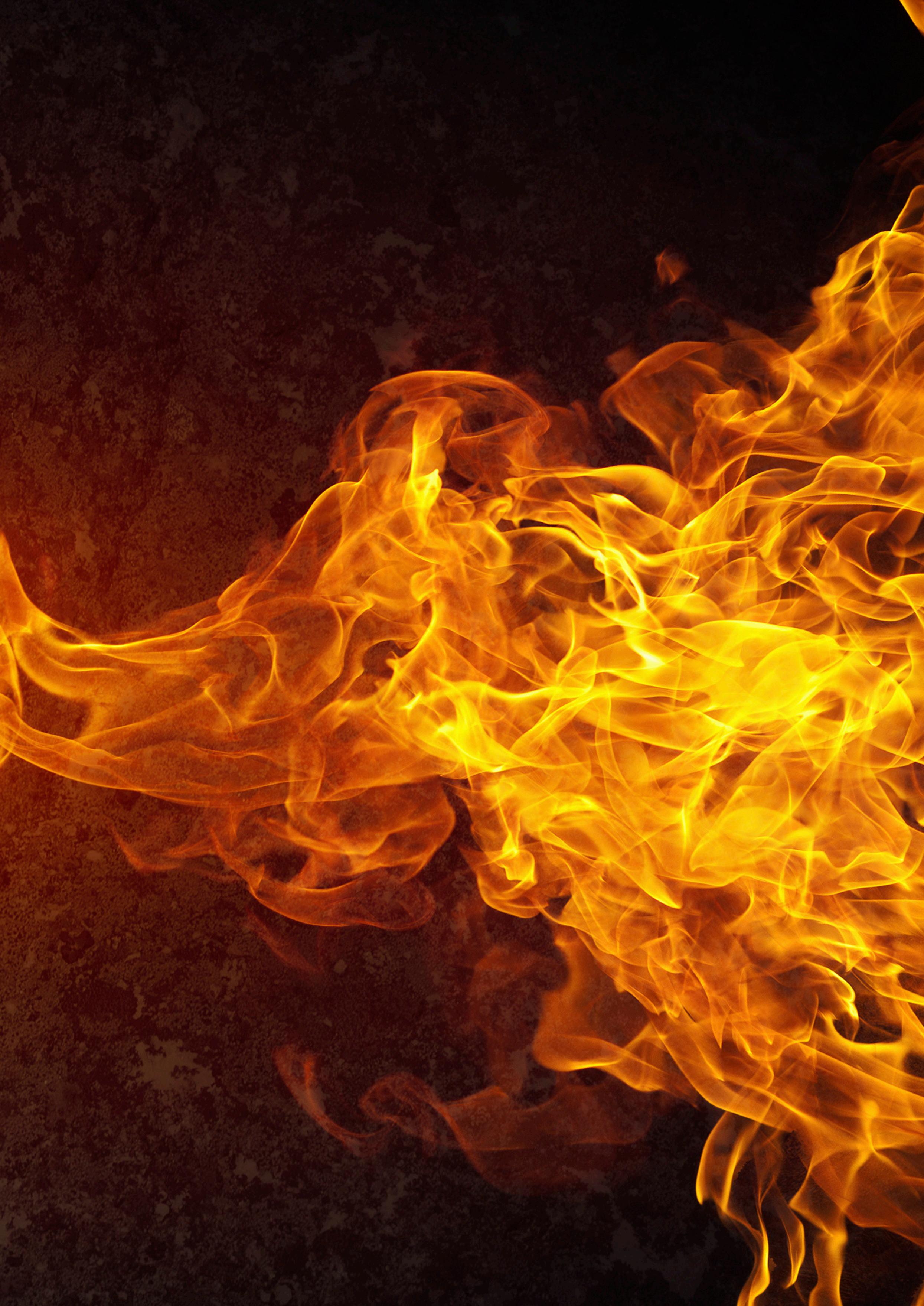
electrification is still in its relatively early stages, ‘how best to ensure safety’ is still in early discussions for many insurers and government bodies and this is not something that has been shared widely across the market yet.
There are some advisory American standards, which some manufacturers and suppliers can choose to follow, such as UL 9540; however, these are not mandatory and are not replicated across the globe and into Europe, for instance. Without legal standards enforcing best practice safety measures, it too often will fall down to price, and battery storage facilities will ask – ‘what is the cheapest solution?’ The short-term answer is to opt for a lower-quality fire detection system that is not fit for purpose – or even to avoid selecting a system altogether. In the longer-term, however, there is a distinct trade off in price – as the cost of the risk once realised will often far outweigh the cost of selecting the right system from the outset.
In the absence of mandatory safety guidance, leaving critical safety decisions down to price at the hands of the organisations is incredibly dangerous.
Out with the old, in with the new…or not?
It is also not just new technology batteries causing the risk. With more electricity being used for almost every application, there is also a growing presence of old, scrap batteries in waste streams, which also need to be stored carefully, as they are subject to the same risks as newer batteries – on an arguably greater scale, due to the harsh environment created by the waste processing stream.
There is also an increasing presence of scrap batteries in manufacturing processes. For example, when car manufacturers have issues with batteries during development stages, these can be replaced in the final model (after the batteries have been manufactured on a large scale) to prevent faulty products being issued to consumers. In these situations, the former batteries are often discarded. However, they will need to be stored somewhere safely before being repurposed for use in alternative parts or models. Again, the same safety risks apply in these circumstances, which means they need to be considered within the facilities fire suppression system.
Overcoming risks to enable a safer, greener future
The first thing to note is that traditional fire detection and suppression systems will often not effectively eliminate risks for li-ion batteries, as, in thermal runaway a battery has the ability to produce its own source of oxygen, which propagates any flames from within, self-sustaining a fire. This makes suppression using traditional measures particularly challenging, as it needs to fight the fire from within the battery to control it effectively. There are other solutions being trialled, such as aerosols or powders, however, while these may take out initial flames, it will not halt the entire process or eliminate the safety risk.
Research has found that to extinguish these types of fires effectively, suppression systems need to apply huge quantities of water for extended periods of time – and this is just for a singular battery. For a whole unit of batteries storing energy for later use, the amount of water that would be required is amplified substantially – which is in direct contrast with the environmental initiatives that led to the need for an increase in energy storage capacity.
As a result, there is a need for a new, unique detection system. Following extensive research and testing into electric battery fires, Research Institutes of Sweden (RISE) and Dafo Vehicle Fire Protection uncovered that an early fire warning system, supported by targeted spot cooling ahead of thermal runaway is often the most effective way to prevent this from taking place within a li-ion battery, reducing the likelihood of fire and explosion.
The key is to detect a potential battery failure in its earliest possible stages, when the battery vents gases, before taking immediate action to cool. This will ultimately stop, or delay, a potentially dangerous situation from progressing, preventing the fire from developing further. Dafo have developed a unique system, which comprises of a cooling system built inside the energy rack for highly-efficient cooling.
By cooling the inside of the battery pack as soon as temperatures begin to rise, you’re able to contain the risk –mitigating its consequences across the entire energy storage facility, to mitigate potential damage to the facility and threat to life.
A risk destined to grow
Inevitably, as the world continues to demand more sustainable energy, energy storage facilities will increase and expand existing capacities to keep pace. This will have a knock-on effect on the likelihood of fires, increasing potential risks as a result.
Increasing sustainability is a must for the planet’s future – but doing so safely is critical for protecting people, surrounding assets, and the environment. As battery storage develops, it has to be about detecting risks in the earliest possible stages – with the appropriate, tailored detection solutions – and implementing spot cooling measures immediately.
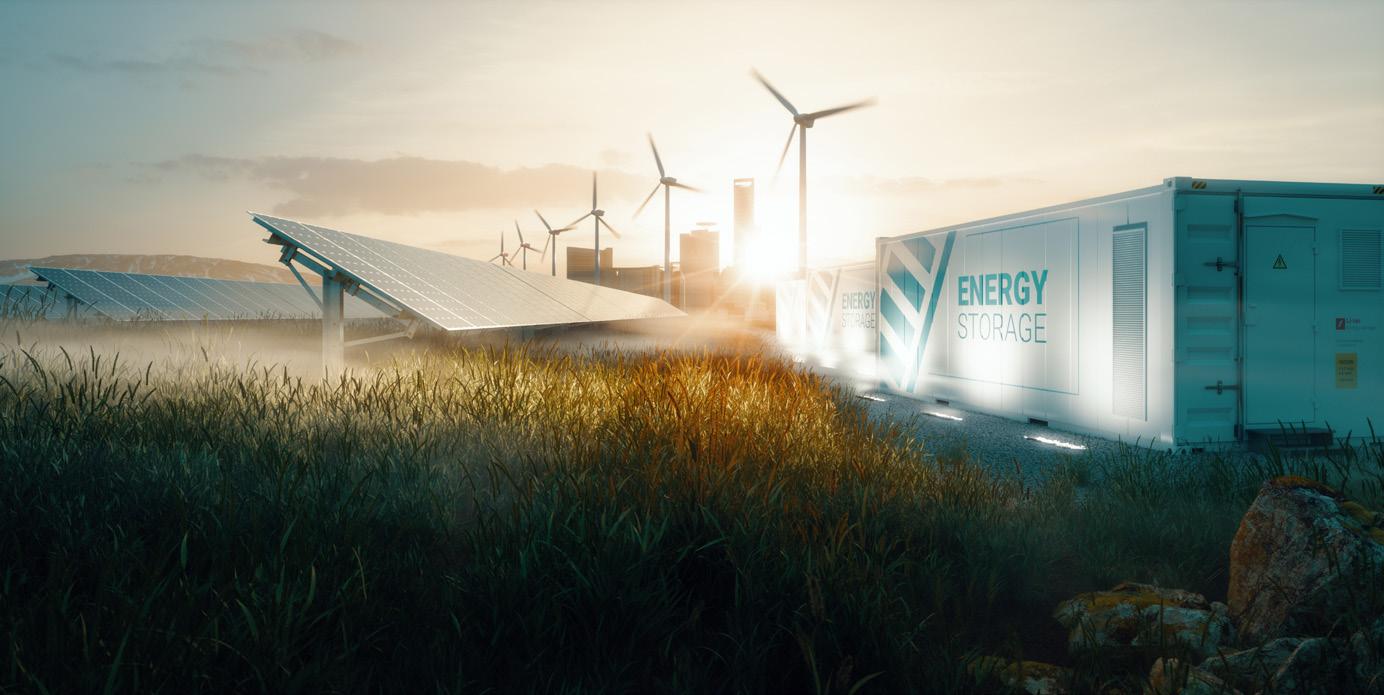
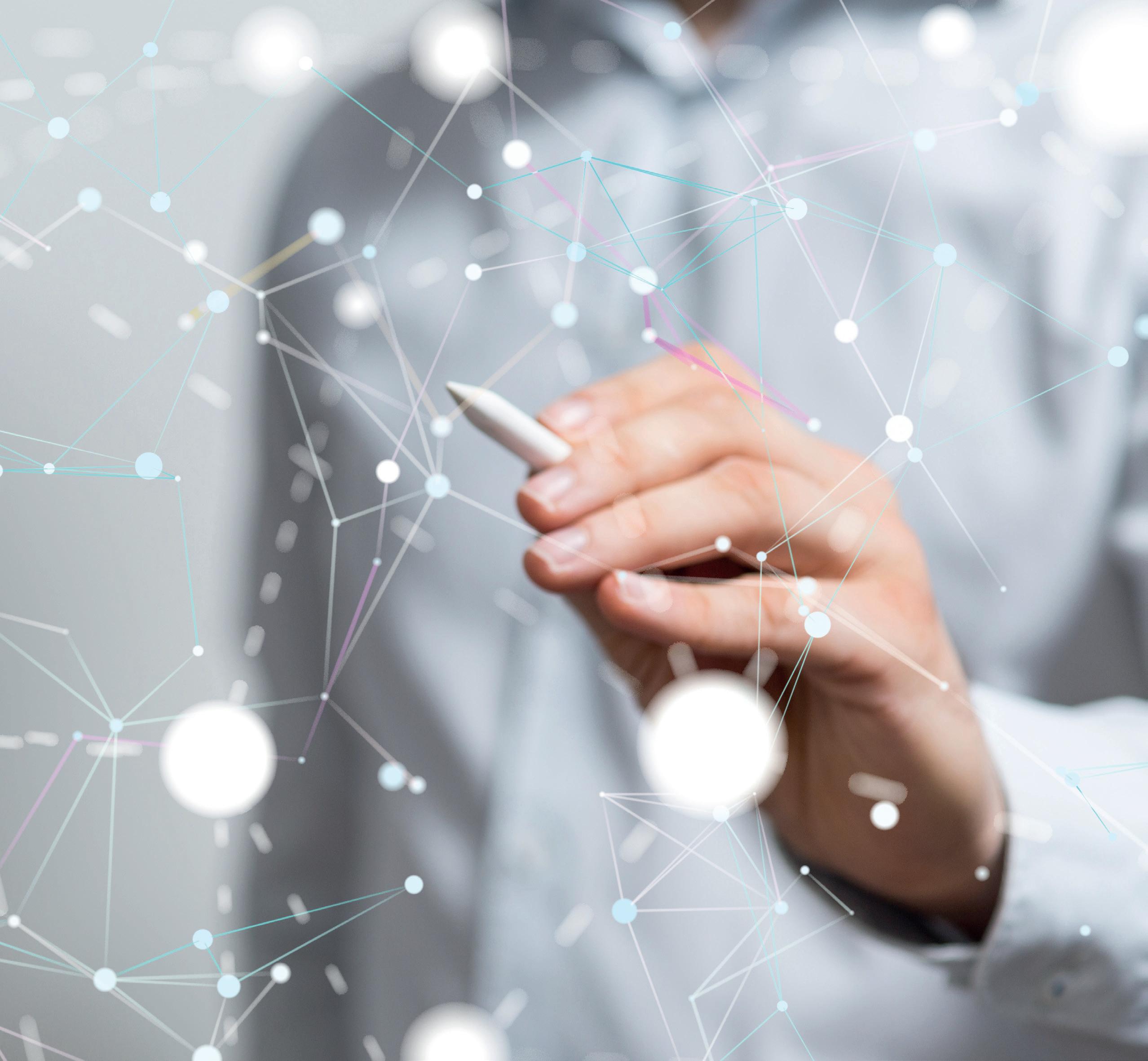
Matt Harper, Invinity, Canada, highlights the importance of battery storage solutions in order to achieve a ‘just transition’ in the energy industry.
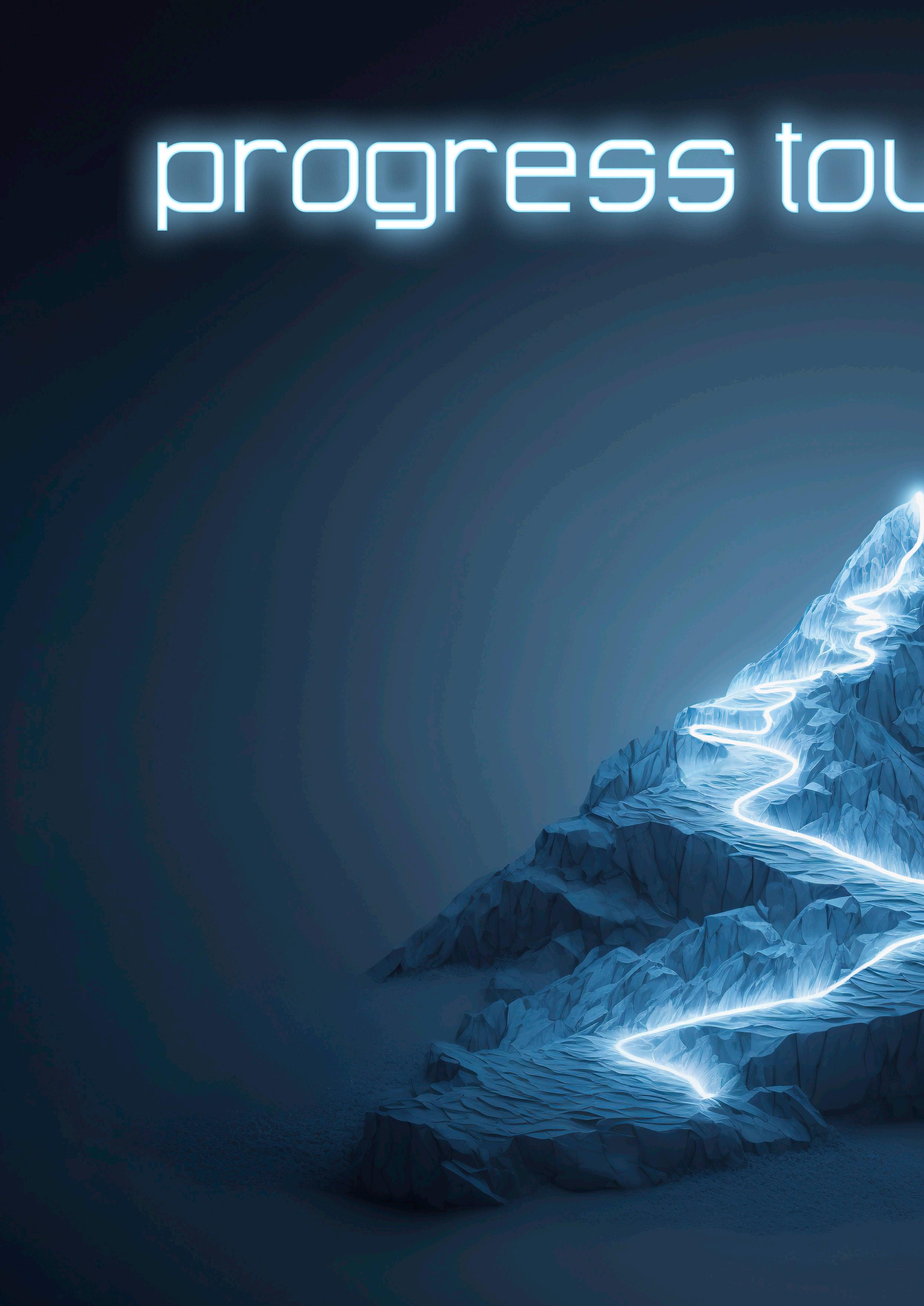
The world is shifting to renewables. The move away from the traditional building blocks of modern society – coal, gas, and oil – and towards clean renewable energy sources is no longer a choice. It is vital to transform the global energy systems and create a sustainable future for the planet.
In 2022, the effects of fossil fuel reliance have become ever more visible, notably the geopolitical challenges and economic instability born by the war in Ukraine and the resulting impact on global energy prices. Now more than ever, the combination of rapidly falling costs and increasing support for societal and ecological benefits are driving the rapid adoption of renewable energy generation, making such sources look ever more like the dominant form of energy as the world moves towards net zero.
The US and Europe are leading this transition, using major infrastructure bills to deliver a strong push towards renewables. Subsequently, other countries are taking
note as they also look to diversify their energy sources in the short-term and set themselves on the path for fully decarbonised economies in the long-term. Global solar and wind deployments, increasingly accompanied by grid scale energy storage, are at a record high as countries look to lock in long-term energy security and tackle record high prices, all while achieving decarbonisation goals.
All of this has led to the deployment of renewables and energy storage faster and at a greater scale than has ever been seen before. This is great news for the world’s economies, the planet, and net zero ambitions. But this must not be a case of ‘progress at any cost’. The global transition to renewables presents an opportunity to fundamentally reshape the world to be more equitable, bringing the social, environmental, and economic benefits of clean energy to all, with no one left behind.
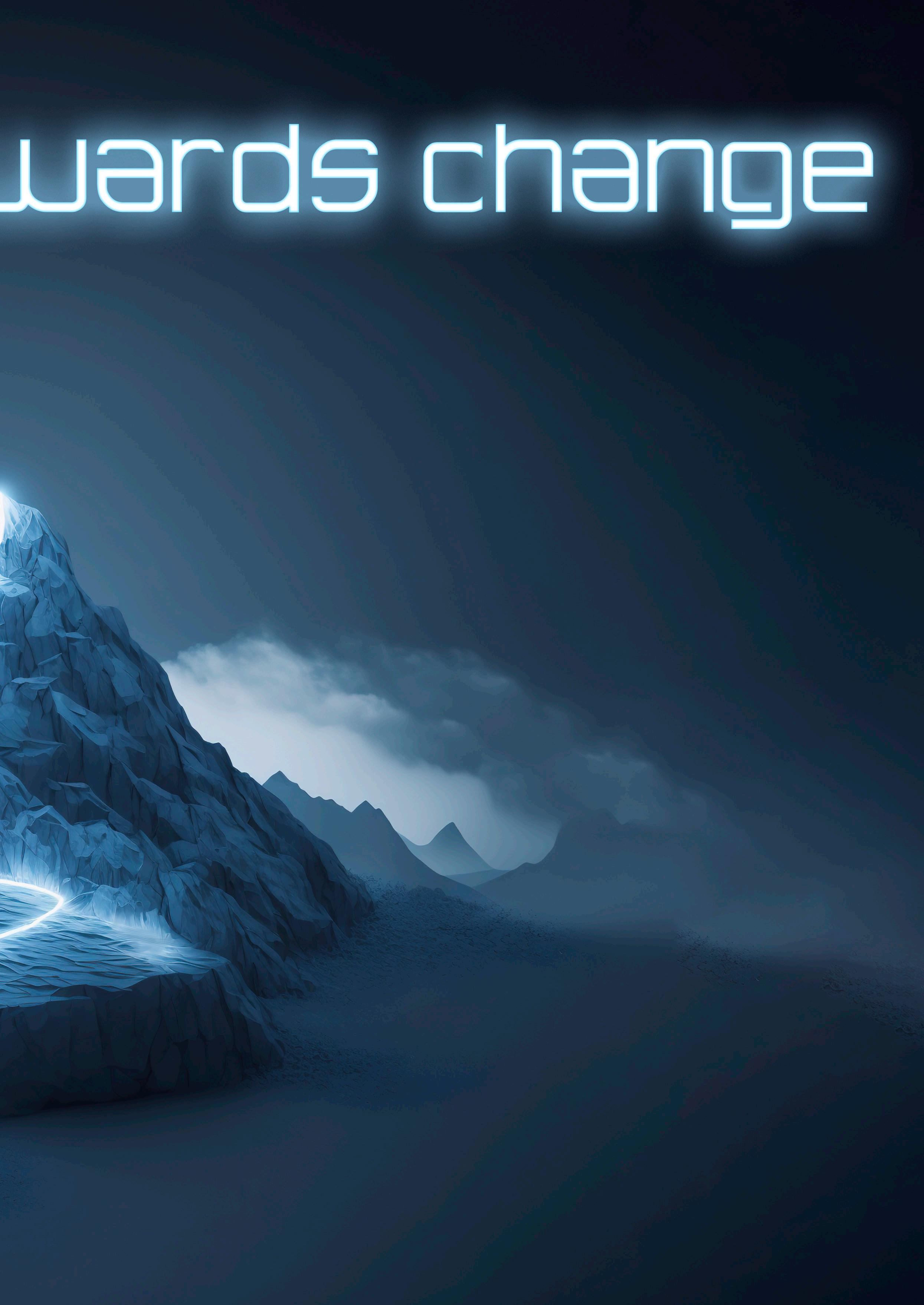
Why is a ‘just transition’ in energy needed?
A ‘just transition’ is the move to an energy system that tackles pressing environmental and societal challenges to benefit everyone, including current and future generations of energy workers. Clean energy is vital to reduce the effects of climate change, pollution, and to help restore biodiversity. Although fossil fuels have driven tremendous economic and technological development, they have also failed many with marginalised communities and low-income countries less able to adapt to extreme weather events, decreased air quality, and environmental pollution.
By contrast, a low carbon energy system driven by renewable energy and supported by grid scale batteries will lead to lower emissions of greenhouse gases (GHGs) and to cleaner air and water. Renewables also produce some of the cheapest electricity available today – making them both an environmentally and economically attractive solution. But to achieve this outcome, governments and organisations must work closely to align policy decisions and investment strategy to power decarbonisation.
If this is done right, this will be the precipice of a transformative period for the global economy, to create a world which is more equitable, climate-resilient, and fair. The clean energy transition will stimulate economic growth, reskill generations of workers, and provide unprecedented energy sovereignty for nations both rich and poor.
However, for countries that are heavily reliant on fossil fuels as the backbone of their economies, the social component of this inevitable transition must be anticipated and supported. Africa’s two largest economies, Nigeria and South Africa, primarily rely on oil and coal, respectively. Moving away from fossil fuels needs to be paired with the creation of new jobs in clean energy and the training to ensure workers have the skills to thrive in this new ‘green economy’.
Ensuring climate resilience
It is increasingly obvious that climate change is fundamentally reshaping the world. Across the globe, severe weather events are increasing in both intensity and frequency. This means communities and countries must fundamentally rethink their approaches to climate resilience. In California, the US, for example, severe weather events regularly force the state’s electric utilities to switch off whole sections of the grid to decrease the risk of wildfires, which in recent years have ravaged the state. Earlier this year, the California power grid had to labour to keep up through snowfall not seen in a generation. In a bid for greater resilience, policymakers are turning to energy storage in the form of both lithium-ion batteries and alternative technologies such as vanadium flow batteries (VFBs) to play a vital stabilising role in the state’s energy future.
A prime example of how batteries provide resiliency is taking shape at a fire station operated by the Soboba Band of Luiseño Indians in Southern California, a region that is increasingly affected by extreme weather events. Their on-site solar panels are coupled with a VFB system, recently supplied by Invinity, that will ensure the station can operate 24/7 by
storing surplus solar energy and releasing it on demand, day or night.
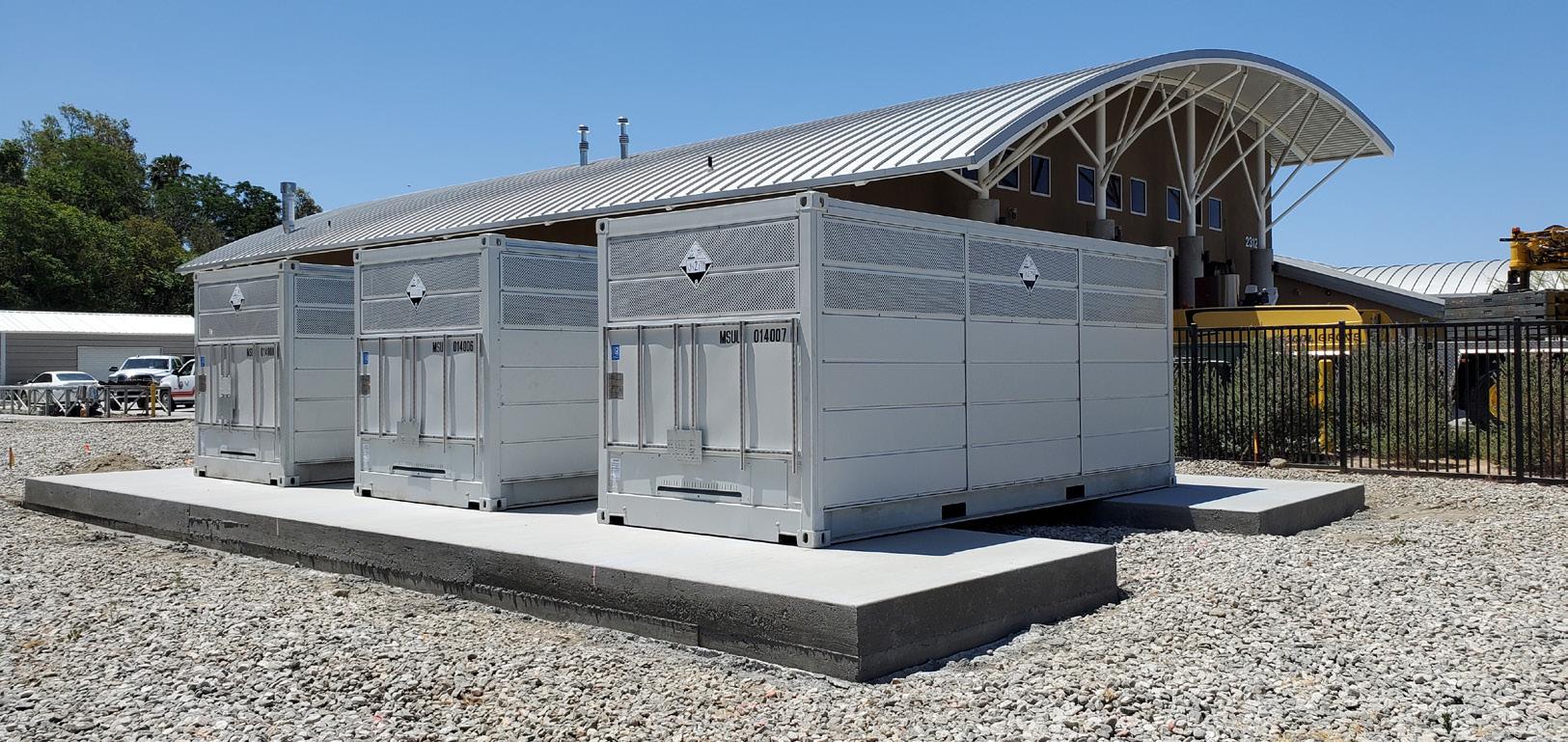
Long duration energy storage (LDES) technologies such as VFBs reduce the impact of wind and solar generation’s intermittent nature by soaking up excess power and discharging it back into the grid for sustained periods when renewable generation is low or demand on the grid is high. VFBs also have a service life exceeding 20 years even in heavy-duty daily usage, delivering better project economics for energy-shifting applications than more common lithium-ion batteries.
The fire station is a vital part of the Soboba community. Tribal residents live in a district identified by the California Public Utilities Commission as a ‘Tier 3 – Extreme’ threat area and have experienced multiple outages linked to wildfires over the last two years. During wildfires, the Soboba fire station serves as the incident command centre and emergency shelter as well as the point of distribution for food, equipment, and supplies – making uninterrupted power vital for community resiliency.
The number of blackouts across California is expected to increase as pre-emptive public safety power shutoff events become more common, so the Soboba Band is planning on expanding services at the fire station site by building a medical centre and community building, along with additional battery backup and microgrid systems for those facilities.
Tribal job trainees will be incorporated as much as possible into the project to increase job readiness within the community. GRID Alternatives Inland Empire, an affiliate of GRID, will be working to strengthen community wide emergency plans and help Soboba Tribal members make the best use of the facility during outages and emergencies.
This project is a perfect example of how renewable energy and storage can enable a just transition to clean energy.
Policy support to unlock energy storage
Recent concerns around energy security have gone a long way in accelerating the deployment of renewable energy, with the US, EU, and China taking the lead. According to the International Energy Agency Renewables Report 2022, the world is going to add as much renewable energy in the next five years as in the previous 20, building on a year where for the first time ever over US$1 trillion was invested in renewable energy infrastructure. But to get more from renewables, it is necessary to get more from the energy storage that is deployed alongside it. Utility scale LDES infrastructure needs to
operate flexibly and reliably, providing both high-throughput and longer-duration capabilities to counteract the intermittency issue.
The US Inflation Reduction Act (IRA) is the biggest federal investment into tackling climate change to date, investing US$369 billion into the energy and climate change sector. This is a massive opportunity to accelerate vital energy storage projects. Previously, batteries were required to be linked to solar photovoltaic (PV) projects to qualify for investment tax credits (ITC). Now that batteries alone are eligible for ITCs, energy storage can be deployed to sites where they will deliver the greatest impact.
Alongside its REPowerEU plan to accelerate the deployment of renewables, the EU has introduced its own version of the US IRA, the Green Deal Industrial Plan for the Net Zero age.1 The plan includes a new EU regulatory framework for batteries to ensure a competitive and resilient regional value chain. This is particularly important as the battery industry alone estimates it will need 800 000 additional workers by 2025, underlining the need for key skills to support this roll-out. Wood Mackenzie forecasts that capacity will need to expand 20-fold by 2031 to meet these demands,2 with both REPowerEU and the Green Deal Industrial Plan helping to create the market conditions necessary to meet this rise in demand.
Moving beyond lithium to proven energy storage alternatives
Energy storage systems are the key to enabling a fully renewable-powered future. To date, the world’s energy markets have been tailored to suit the capabilities of lithium-ion batteries, a technology developed for mobility and electric vehicle applications but also capable of providing some services for the electric grid. Lithium batteries were primarily deployed on the grid to correct infrequent deviations and fill short-term supply gaps (i.e. up to 30 mins.); as such, they have played a role in proving how batteries can be an important part of the energy system.
But the rapid growth in the electric vehicle market for which lithium batteries were originally developed is putting enormous pressure on battery supply chains, thereby reducing the availability of lithium batteries for grid storage. Additionally, grid-connected battery storage systems are being used harder than in EV applications, shortening their expected lifespan and increasing potential fire risks. Critically, the difficulty in recycling lithium batteries means they are projected to account for 2 million t of hazardous waste by 2030.3 This is not a sustainable long-term solution. The battery sector needs to look to alternative and innovative technologies to meet the growing demand for delivering renewable energy when people’s homes, businesses, and industry need it. It is time to capitalise on the momentum built by lithium batteries and embrace more sustainable battery storage solutions.
To shift to an economy incorporating ‘just transition’ principles, it is important to look beyond lithium as the ‘one-size-fits-all’ option, accept its limitations, and look to a more diverse group of battery technologies to store and deliver clean power on demand for all.
A multi-technology transition
VFBs are already recognised as the foremost alternative to lithium-ion for stationary energy storage applications. VFBs have gained significant interest around the world for their unmatched capacity to store and supply electricity on an industrial scale. They are a form of LDES capable of providing from two to more than 10 hours of energy on demand. One significant advantage of VFBs is their primary ingredient: vanadium, a globally abundant element that is more common than lithium, copper, nickel, or cobalt in the Earth’s crust.
VFBs are much more durable, present no fire risk even in extreme environments, and exhibit no degradation with heavy daily use. They also respond extremely quickly to the demand for power, allowing them to provide the same grid support services as lithium-ion batteries but doing so for 25 years or longer. A VFB’s electrolyte is also fully recyclable, an attribute that allies well with the ecological and economic demands of the ‘just transition’.
Invinity has 65 MWh of VFBs already deployed or contracted for delivery at more than 70 sites around the world, with the installed capacity of the company’s batteries set to more than double in 2023.
The time for change is now
With the increasing deployment of renewable and energy storage technologies globally, the world is making important progress towards a ‘just transition’ to renewable energy. Significant policy interventions that support the creation of green jobs and sustainable investments are shining examples of the increased ambition and momentum towards decarbonisation.
The role that energy storage has to play in that transition is becoming increasingly clear, with 10 countries having now established targets for storage alongside renewables. These policies need to be replicated and strengthened globally to ensure this positive momentum towards ever-more clean power continues. Developed nations must support less-developed economies with their energy transitions so that rapidly growing economies’ continued reliance on fossil fuels does not undermine global progress. Supporting the deployment of cost-effective and sustainable energy storage solutions is key to enabling the transition and unlocking the green economy, which will provide a new generation of sustainable jobs. The long-term benefits of the sustainable energy transition are clear, and the world must now strive towards the goal of reaching net zero with no one left behind.
References
1. European Commission, ‘Communication from the Commission to the European Parliament, the European Council, the Council, the European Economic and Social Committee and the Committee of the Regions: A Green Deal Industrial Plan for the Net-Zero Age’, (February 2023), https://commission.europa. eu/system/files/2023-02/COM_2023_62_2_EN_ACT_A%20Green%20Deal%20 Industrial%20Plan%20for%20the%20Net-Zero%20Age.pdf
2. ‘Europe grid-scale energy storage outlook 2022’, Wood Mackenzie (23 March 2022), www.woodmac.com/reports/power-markets-europe-gridscale-energy-storage-outlook-2022-150016066/
3. JACOBY, M., ‘It’s time to get serious about recycling lithium-ion batteries’, Chemical & Engineering News, (14 July 2019), https://cen.acs.org/materials/ energy-storage/time-serious-recycling-lithium/97/i28
With the great focus on the green transition, one type of renewable energy can be produced without being dependent on the weather, and that is biomethane. Biomethane can substitute natural gas which is being pumped from the underground. Therefore, biomethane is a key to the green transition and to carbon neutrality. Besides biomethane production, a new movement of power-to-X is rising and boosting the green transition. Nature Energy A/S is a Danish company which promotes biomethane production, and with their upscaling of a power-to-X project over the last four years, they have the green transition in focus.
Biogas production
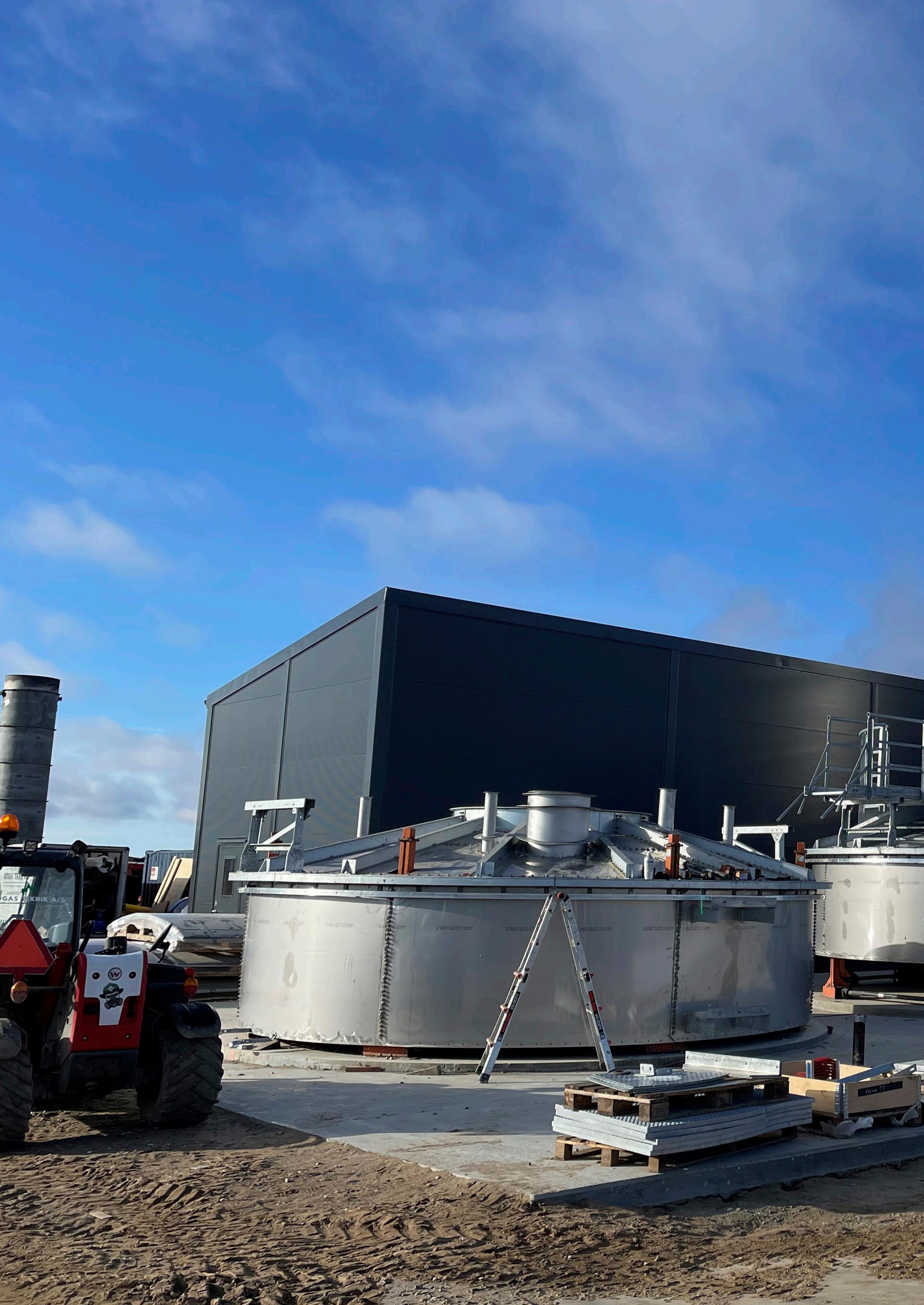
Nature Energy is one of the world’s leading biogas producers, with 14 full scale biogas plants in operation, producing more than 200 million m3 biomethane, which is injected directly into the natural gas grid. The contribution of biomethane into the natural gas grid reached an all-time high in the Danish natural gas grid of >30% in 2022.1 Of the 30%, Nature Energy alone contributed one-third of the biomethane, covering a total of 10% of the Danish gas grid consumption in 2022.
Biogas production is an anaerobic process where organic material is utilised in a fermenter to produce biogas. The breakdown of organic
material will, during four steps, be reduced from long chains of organic material to two of the smallest and simplest organic molecules, methane (CH4) and carbon dioxide (CO2). The organic material varies from farm-based, such as straw, deep litter, and manure, towards industrial waste, such as food waste, potato pulp, etc. The contribution of waste products to energy production, while the degassed biomass (digestate) is given back to the farmers, ties the production and the suppliers together, making it a circular economy (Figure 2).
Besides the full scale gas production, Nature Energy is looking into ways to improve the biogas process and utilise the side streams from biogas production. A major focus for Nature Energy is to improve the utilisation of carbon in the process, and from the biogas production the CO2 is seen as a waste product. Since biogas production is a circular economy, the CO2 is commonly discharged into the atmosphere. At Nature Energy Korskro, the CO2 is not being discharged, but liquefied by compression and low temperature and then utilised in the Danish food sector. The production from this plant alone covers 25 – 30% of the total CO2 usage in the food industry in Denmark, making this an unviable solution for all biogas plants to utilise the CO2
Brian D. Jønson, Nature Energy, Denmark, considers how power-to-X is boosting the green transition, with biogas and biomethane presenting viable alternative fuel solutions.
S c a l E U P
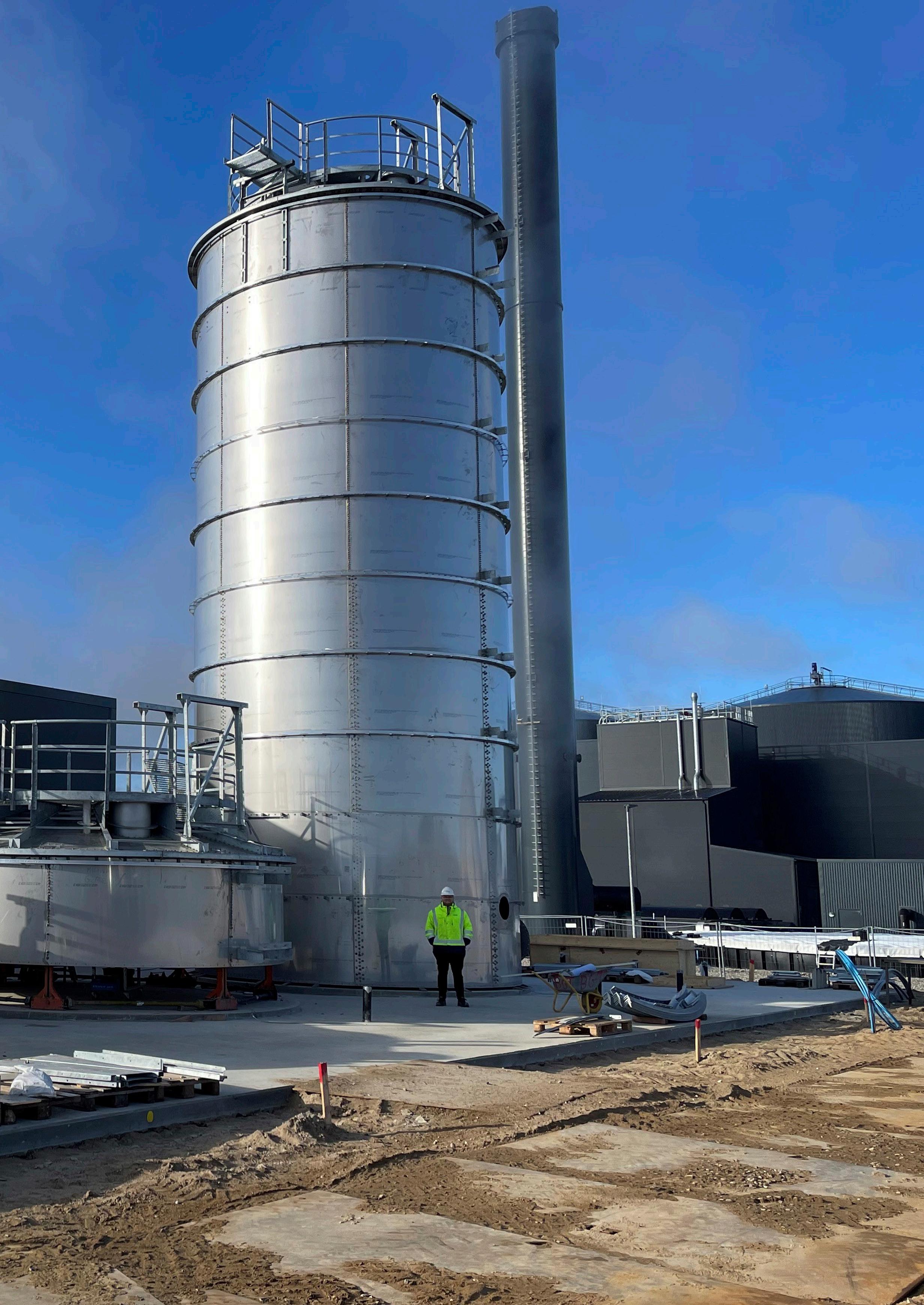
Another way to utilise CO2 is to combine it with hydrogen (H2) to produce a range of chemicals and products from small organic molecules, such as CH4 to larger organic molecules which can substitute jet fuel. The H2 is sourced from renewable energy through an electrolyser unit which will use electricity to split water into H2 and oxygen (O2). This is equal to storing the electricity as H2 and is therefore known as power-to-X, which is a term used for utilising power (electricity) to produce (store in) another product (X).
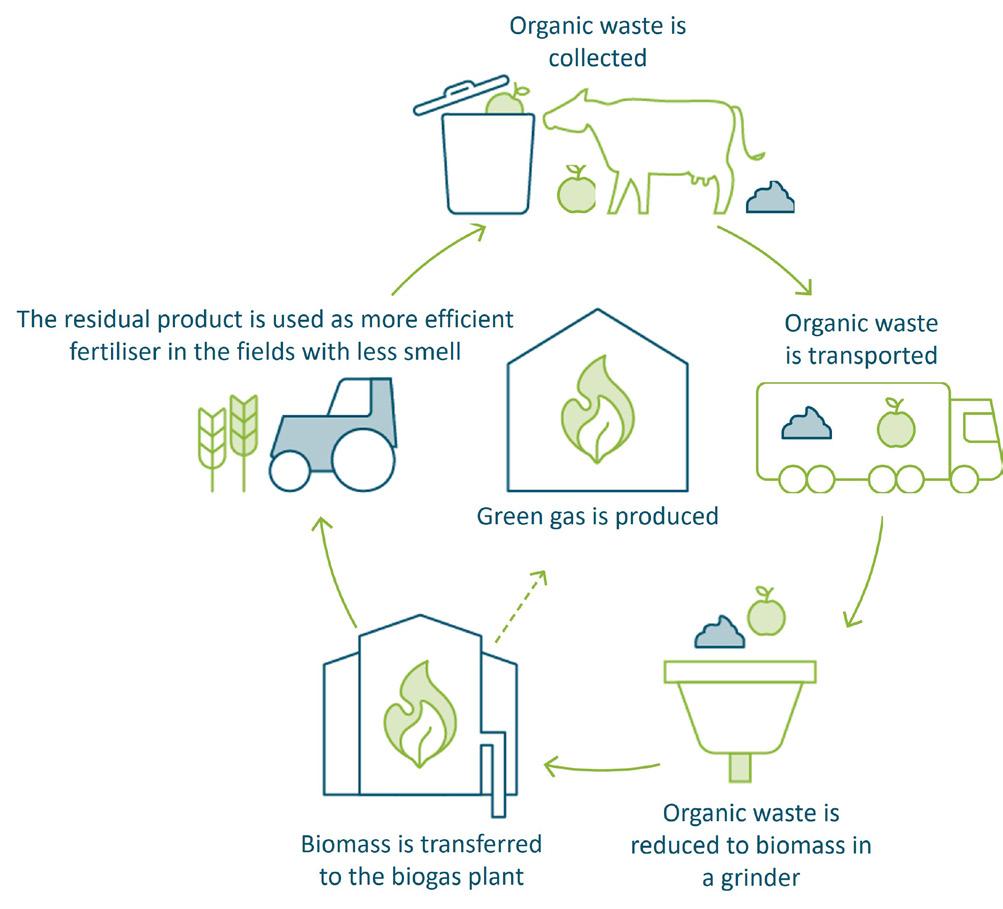
Power-to-X project
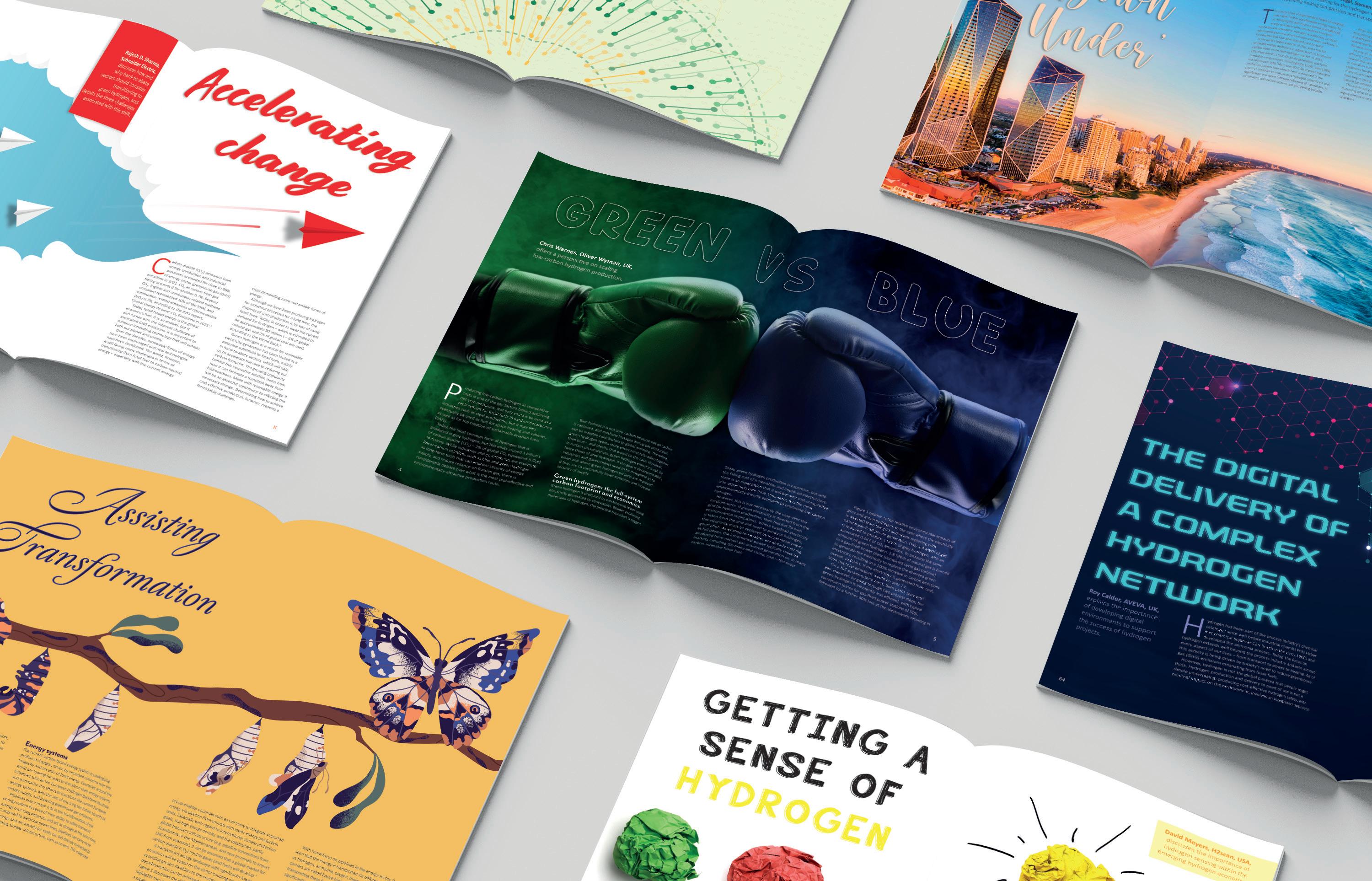
Since the autumn of 2019, Nature Energy has invested resources to research the utilisation of CO2 from the biogas production, by hiring a PhD-student, Brian Dahl Jønson. He would work in a funded project, ‘eFuel – Electrofuel from a bio-trickling filter’, which is an
Energiteknologisk Udviklings – og Demonstrationsprogram (EUDP) project (Project ID 64018-0559). The project was a collaboration between Nature Energy, BiogasClean A/S, University of Southern Denmark, and the Technical University of Denmark. The focus of this project was to demonstrate a biological way to utilise CO2 from a biogas plant. One way to utilise CO2 is to combine it with H2, and either with a chemical or biological catalyst convert it into CH4. This is known as methanation.
The methanation process is a familiar process as it has been used in chemical systems for many years, formally known as the Sabatier Process, where CO 2 and H 2 are converted over, e.g. an iron-based catalyst at elevated temperatures of >300˚C and pressures of >4 bar. The most known industrial scale process of the chemical methanation is from Audi in Werlte, where they have produced e-methane since 2013. 2
The technology used for Nature Energy’s project was a biological trickling filter (BTF) (Figure 3). The technology is known as a three-phase reactor with gas, liquid, and solid. The gas and liquid are of co-current flow, and the solids are a packing material which will maximise the surface area on which biofilm can be formed. The high surface area of biofilm gives this reactor an advantage of better solubility of gases into the biofilm, as more surface area is available. Besides this, the reactor was operated with ambient pressure and temperatures of 55 – 65˚C.
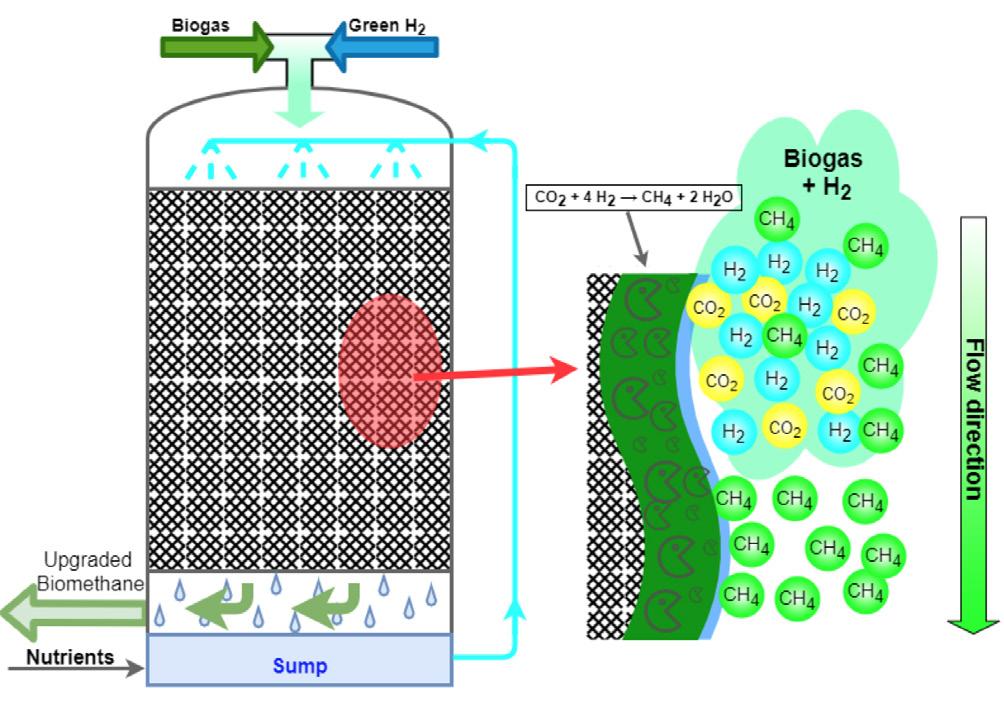
The culture used for the BTF was a mixed culture which originated from Nature Energy full scale biogas digesters. The culture needs hydrogenotrophic methanogens (HM) as these will convert CO 2 and H 2 to CH 4 by their metabolism. Within a biogas digester, these types of methanogens are present and will contribute 25 – 33% of the biomethane produced.
Laboratory scale, 2019 – 2023
The project started out in reactors of 8 l of active volume (Figure 4). These were laboratory scale reactors, and their purpose was to verify the technology using the mixed culture from Nature Energy. These reactors were run in multiple experiments, ranging from steady-state operation to verify the performance towards adaptation towards a fluctuating energy market.
The results from the laboratory scale verified the technology. The 8 l reactors reached stable production at a gas retention time of 45 min., yielding a production capacity of 4.5 m 3 CH 4 m R -3 d -1, which is a measure of the volume of CH 4 (m 3 CH 4) produced per volume of reactor (m R -3) per day (d -1). Besides these results, another experiment showed how effectively the BTF could follow the intermittent energy system. 3 The results showed how the feed of H 2 could be cut off for periods of up to three days, where they could return to the initial performance within hours. These results clearly indicated how this technology has the possibility to work in synergy with the fluctuation of renewable energies in the
Figure 2 Illustration of circular economy, where waste and side-streams work in synergy from biogas production and farmers.energy system. These results were all contributing to the next step in the project, to upscale the technology from 8 l reactors to 1000 l reactors.
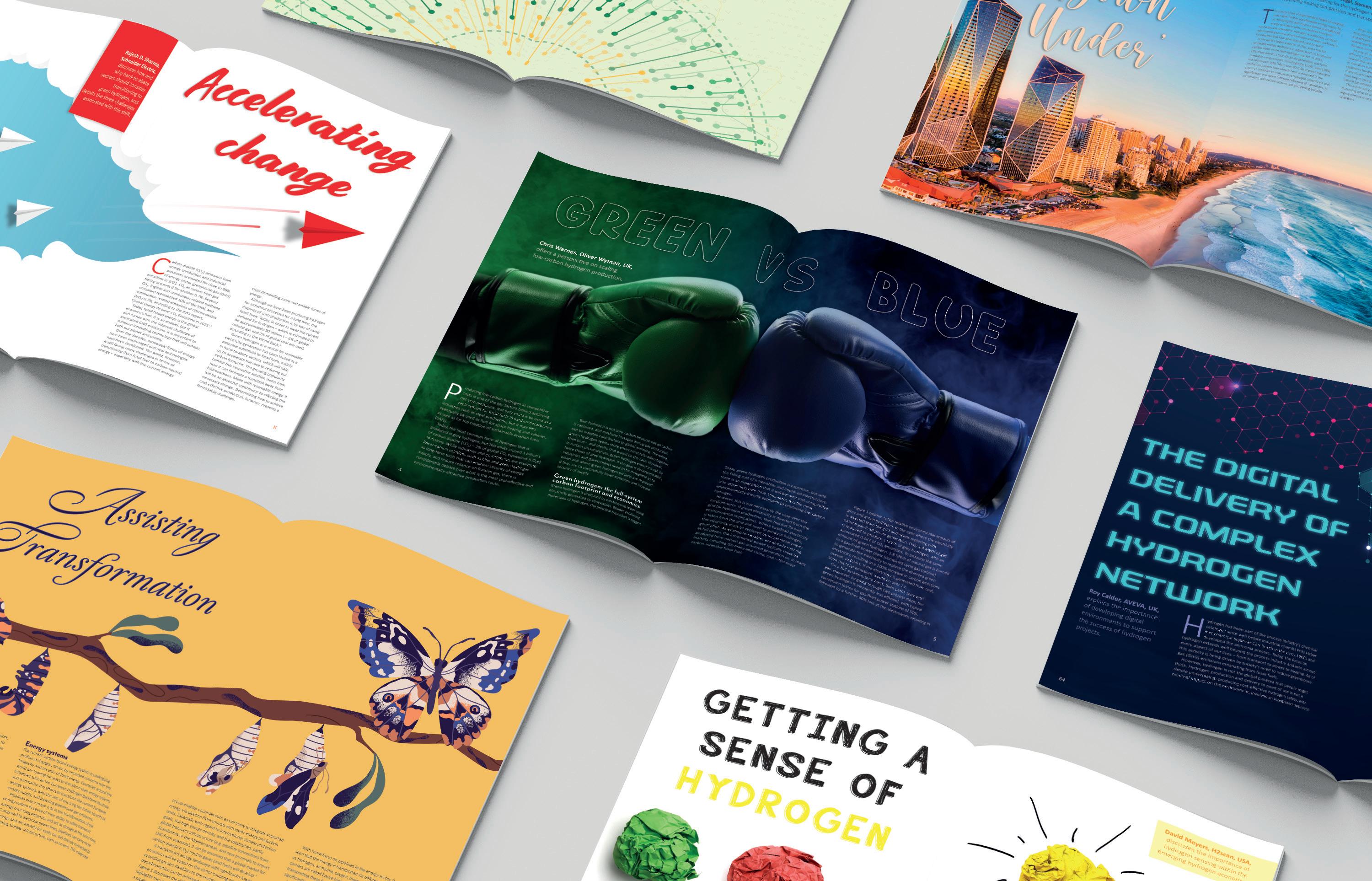
Pilot scale, 2021 – 2023
With the upscaling, new possibilities arrived. The possibility to run on raw biogas along with an electrolyser unit for the H 2 production. For this purpose, two 1000 l reactors were built in a 20 ft container with full automation and control, to give indications of further upscaling and improve the learning from the forthcoming experiments in this scale. The reactors, automation, and control were built by BiogasClean A/S, as part of the EUDP project. It was all fitted into a 20 ft container for convenience and for easy transport. The finished pilot scale is illustrated in Figure 5 and Figure 6.
In the pilot scale it was verified that the technology worked with raw biogas containing 3000 ppm H2S along with other traces. The conversion compared to laboratory scale exceeded the expectations with a PC during steady state operation of 9.4 m3 CH 4 m R -3 d-1. The PC was further increased when the operation was shifted into serial operation, which increased the PC with 12%, reaching 10.6 m3 CH 4 m R -3 d-1. With this operation going on from 2021 to 2023, the technology was verified in upscaled reactors – indicating the potential for this technology in industrial scale. The results from the pilot scale also verified the laboratory findings of flexibility towards an intermittent energy system. All these results, both laboratory and pilot scale, resulted in a further upscaling of the technology in the regime of Nature Energy.
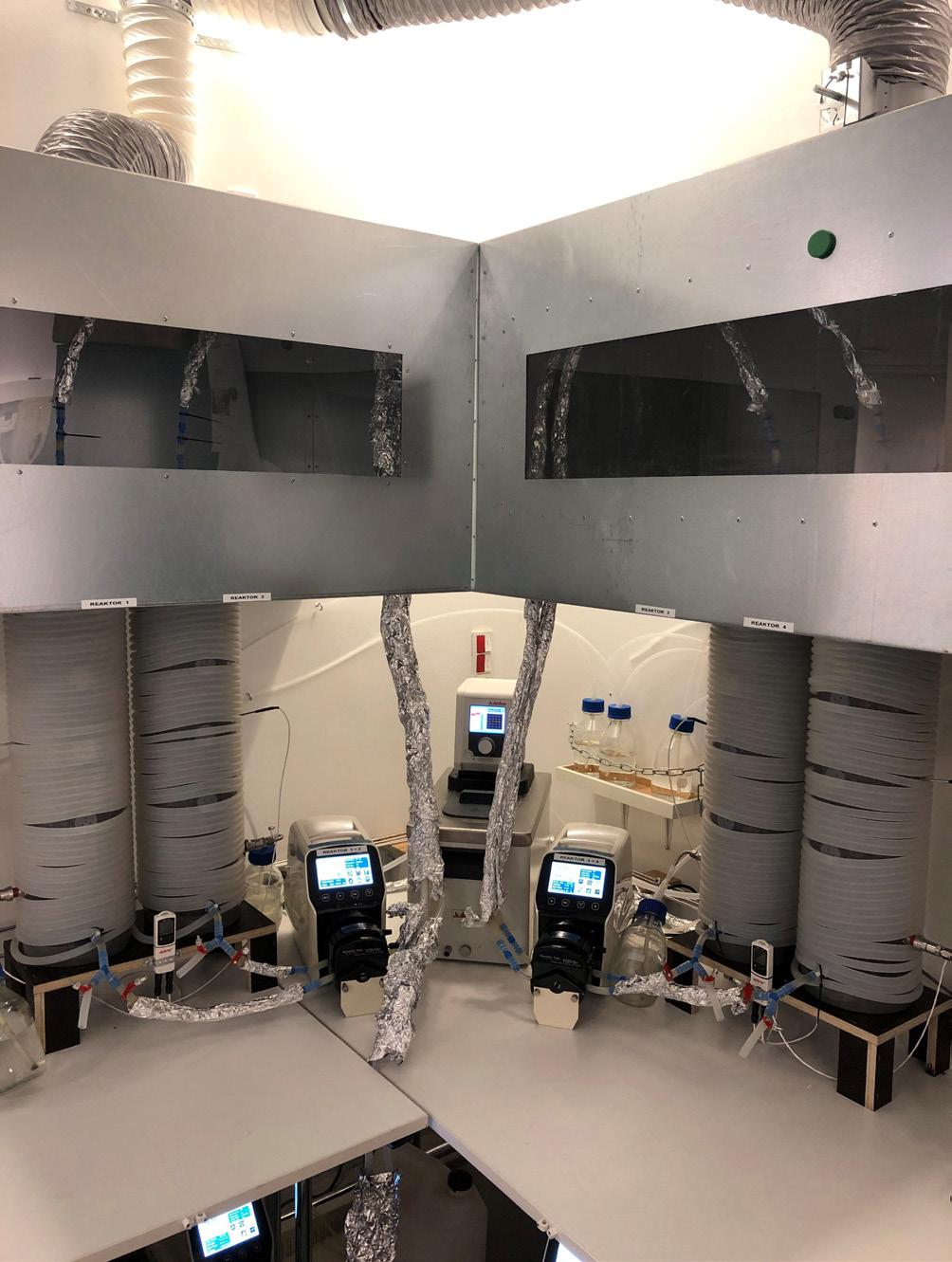
Demonstration scale, 2023 – 2024
The upscaling of the technology was a part of Energy Cluster Denmark’s projects, which was under Erhversfyrtårn Syd, which is a part of a bigger project from
the Danish Board of Business Development, which focuses specifically on the green transition, including power-to-X projects.
In the demonstration scale, the focus was to utilise 25% of the CO 2 from a full scale biogas plant. This equals to 3.3 million m 3 CO 2, or 6500 t of CO 2 which are being utilised yearly. Besides being a part of Erhversfyrtårn Syd projects, Nature Energy is collaborating with Andel, which is a Danish energy company.
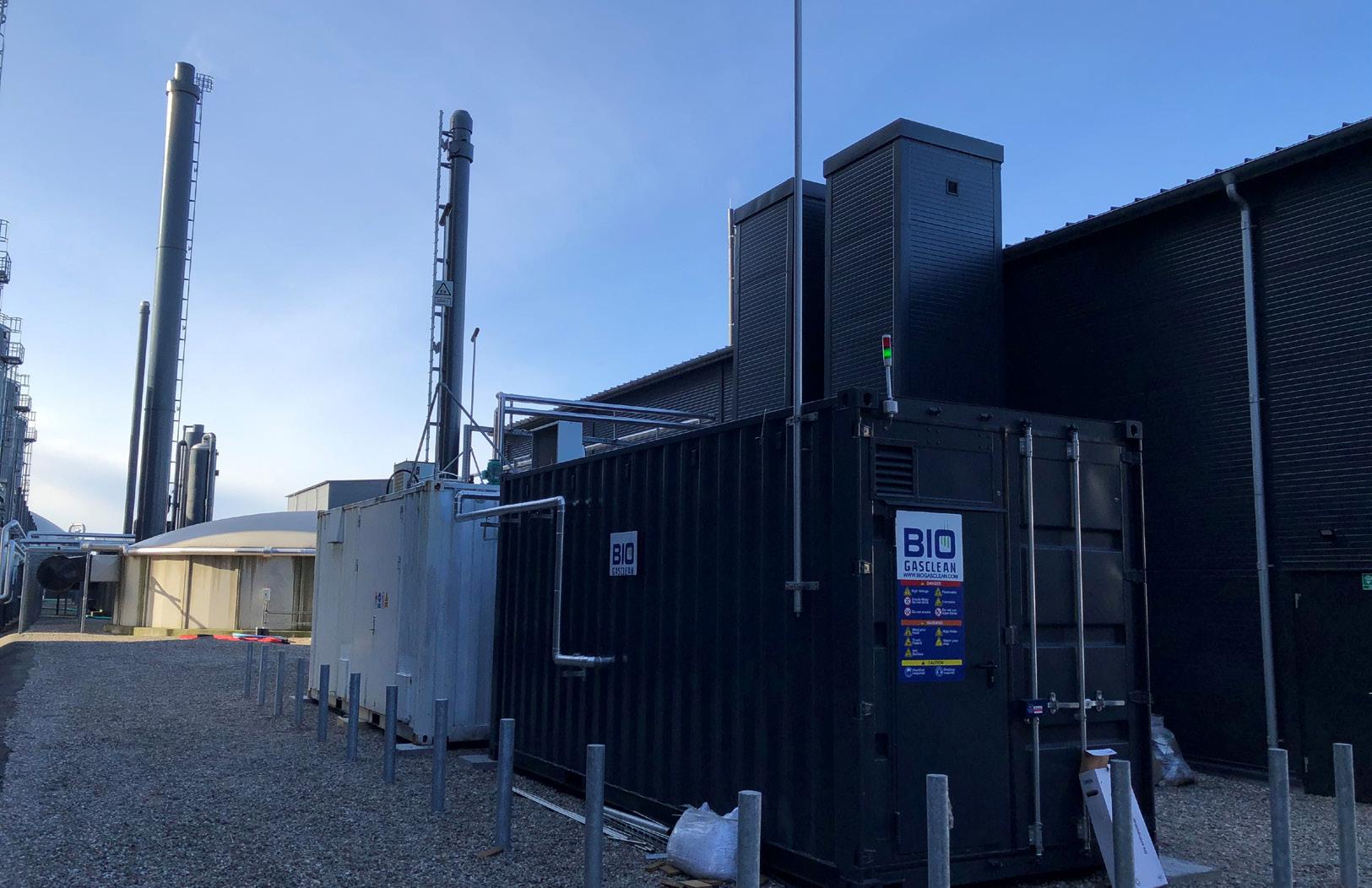
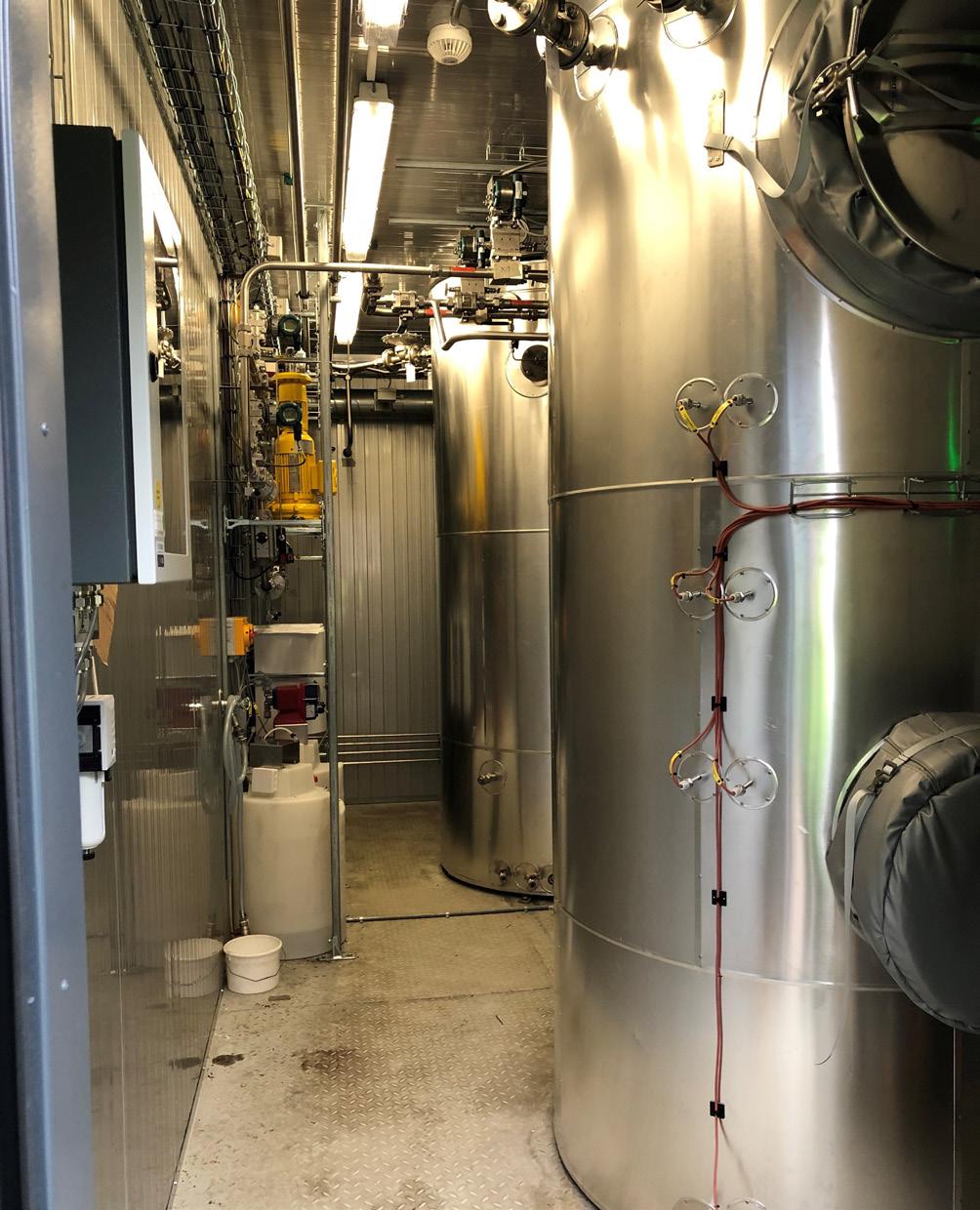
Nature Energy will oversee the process, while Andel will oversee the electricity and electrolysers for H 2 production. The needed electrolyser capacity for the demonstration scale BTFs will be 6 MW. The total capital investment in this project is DKK 100 million (US$14.3 million or €13.3 million).
As of March 2023, the current status on the project is illustrated in Figure 6. The construction of the demonstration plant is on schedule and expected start is late 2Q23. The three biological trickling filters will have an active volume of 300 m 3 each, which is an upscaling factor of 1 million from the first laboratory scales run in 2018 – 2019 by Jønson, or a factor of 37 500 since the 8 l reactors operated at Nature Energy lab in 2019.
The demonstration scale will be the largest of its kind in the world. This huge step towards the green transition, power-to-X, and carbon capture utilisation will hopefully resonate throughout the industry worldwide and start a wave of projects and investments. A lot of investments are calculated, but risk filled, chances have been made towards making this project go from an idea in laboratory scale to an industrial sized technology. These kind of investments and risks are needed to make sure the green transition is accelerated in a way that can follow all needed legislations made from EU and individual countries. Nature Energy expects this project and this technology to show it is possible, and that other industries/companies will follow through.
References
1. ‘Biogas via the gas network, Energinet, https://energinet.dk/gas/biogas/
2. ‘Power-to-gas plant’, Audi, www.audi.com.mt/mt/web/en/models/layer/ technology/g-tron/power-to-gas-plant.html
3. JØNSON, B., MORTENSEN, L., SCHMIDT, J., JEPPESEN, M., and BASTIDAS-OYANEDEL, J-R., ‘Flexibility as the Key to Stability: Optimization of Temperature and Gas Feed during Downtime towards Effective Integration of Biomethanation in an Intermittent Energy System’, MDPI, (11 August 2022), www.mdpi.com/1996-1073/15/16/5827
Figure 6 Pilot scale of the biological trickling filter technology from the EUDP project. Inside of blue container with the two reactors on the right.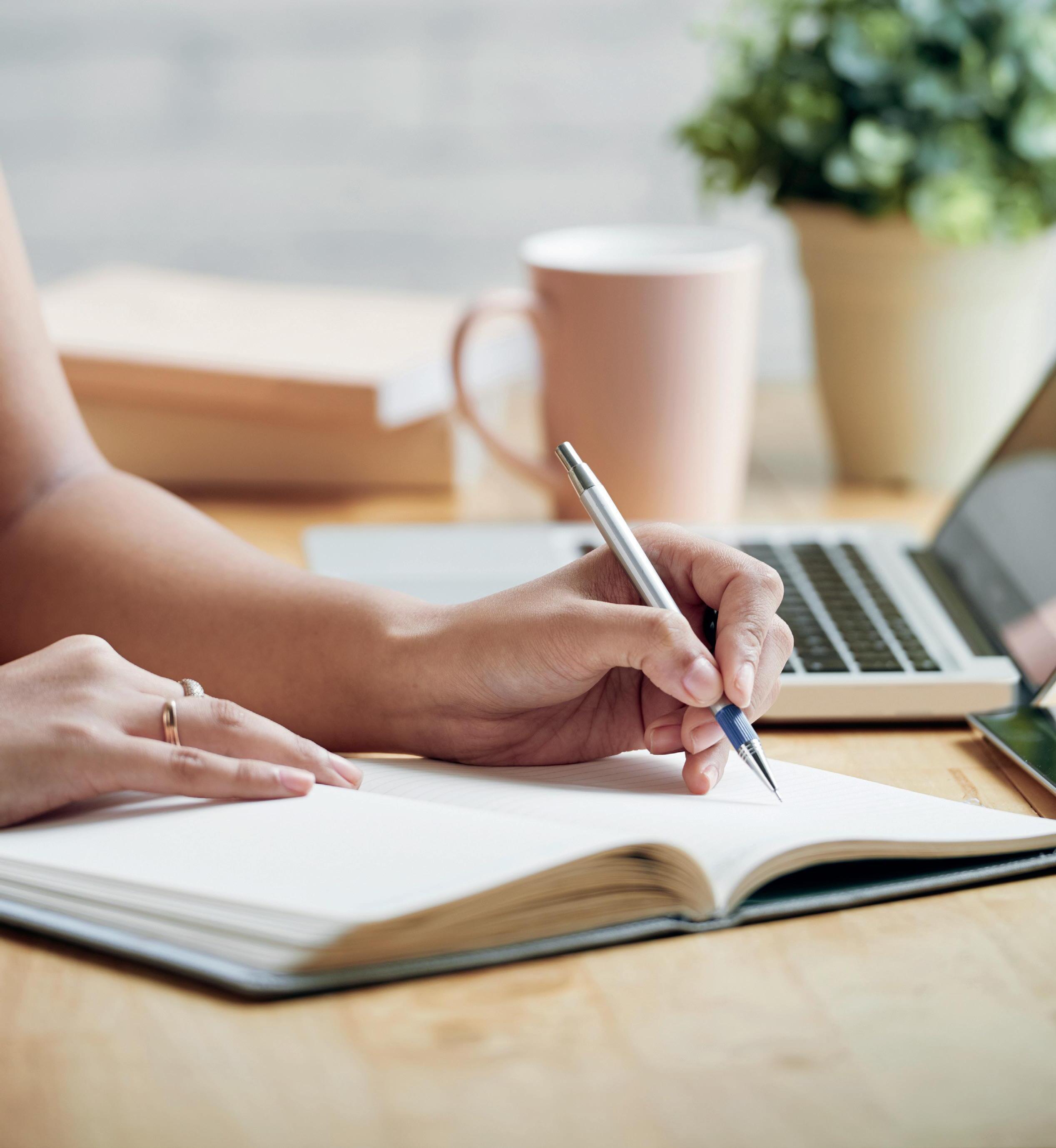
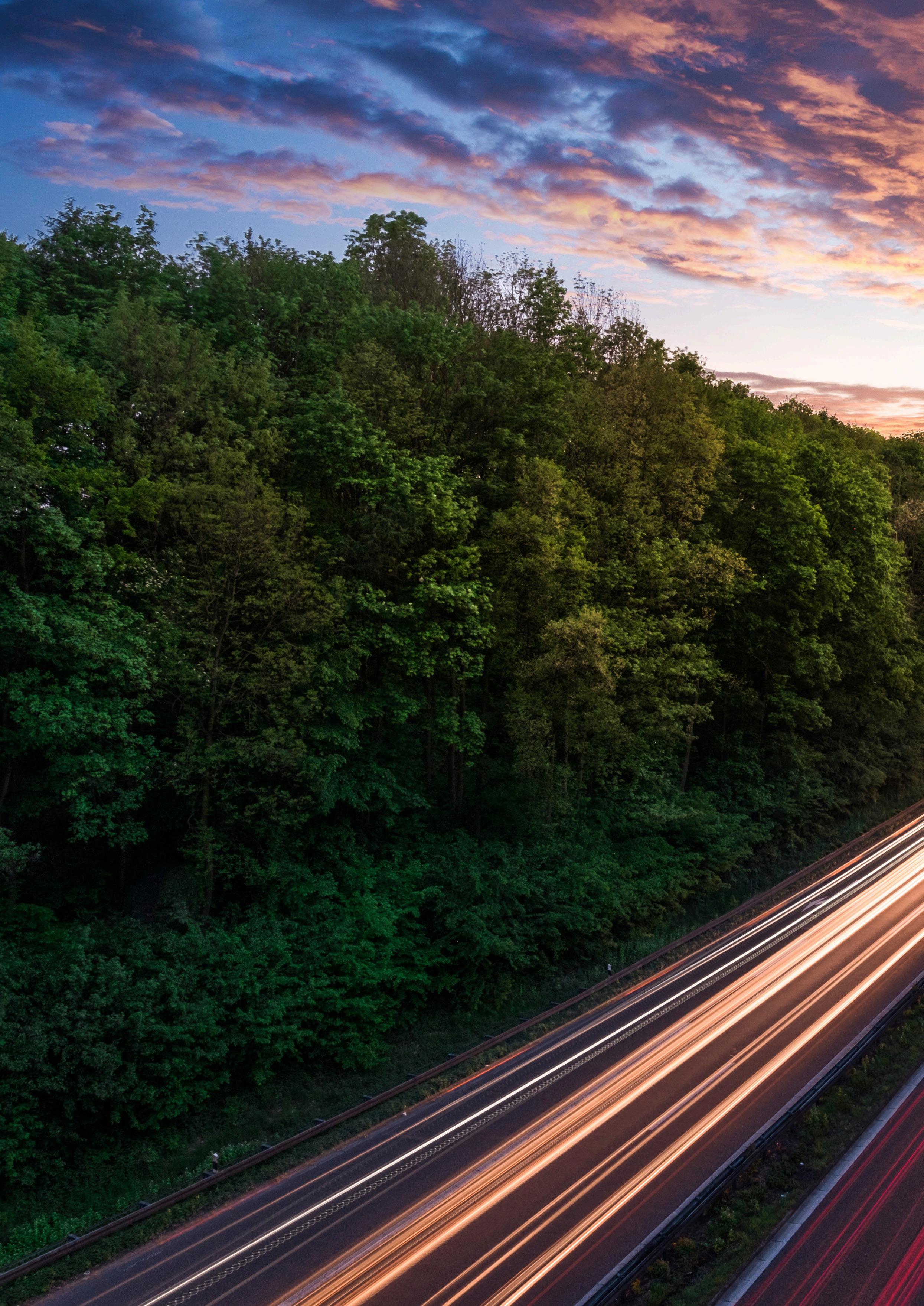
The market for alternative, greener fuels is rapidly picking up pace to support global transport decarbonisation strategies. For these to offer a valuable substitute for their fossil fuel-based counterparts, they need to be economical, readily available, as well as able to meet global demands in terms of quality and quantities. Perhaps more importantly, their production should be as efficient and sustainable as possible to meet net zero targets, requiring companies to adopt state-of-the-art processing solutions.
The transport industry is responsible for a large volume of anthropogenic emissions, accounting for 37% of carbon dioxide (CO2) from end-use sectors, with 7.7 Gt emitted in 2021. This considerable carbon footprint is mostly associated with its continued reliance on fossil fuels, which are used for 91% of the transport sector’s final energy, a value that has largely remained stable since the 1970s, as it has decreased by only 3% since then.1 To meet global carbon net zero emission targets by 2050, the environmental footprint of the fuels used, as well as their demand, should decrease sharply.
Most of today’s forecasts expect liquid fuel demand within the transport sector to peak by 2030 and then remain relatively stable until 2050.2 Various predictive models and studies differ in their estimates of future fuel demands as well as allocations to road passenger, freight, aviation, and shipping. However, they all envisage a considerable decline of traditional oil-based products in favour of alternative, low-carbon fuels, such as electricity, hydrogen-based solutions, as well as biofuels and other products from renewable resources. This is in line with global decarbonisation efforts and transition policies aimed at building 100% renewable energy systems.
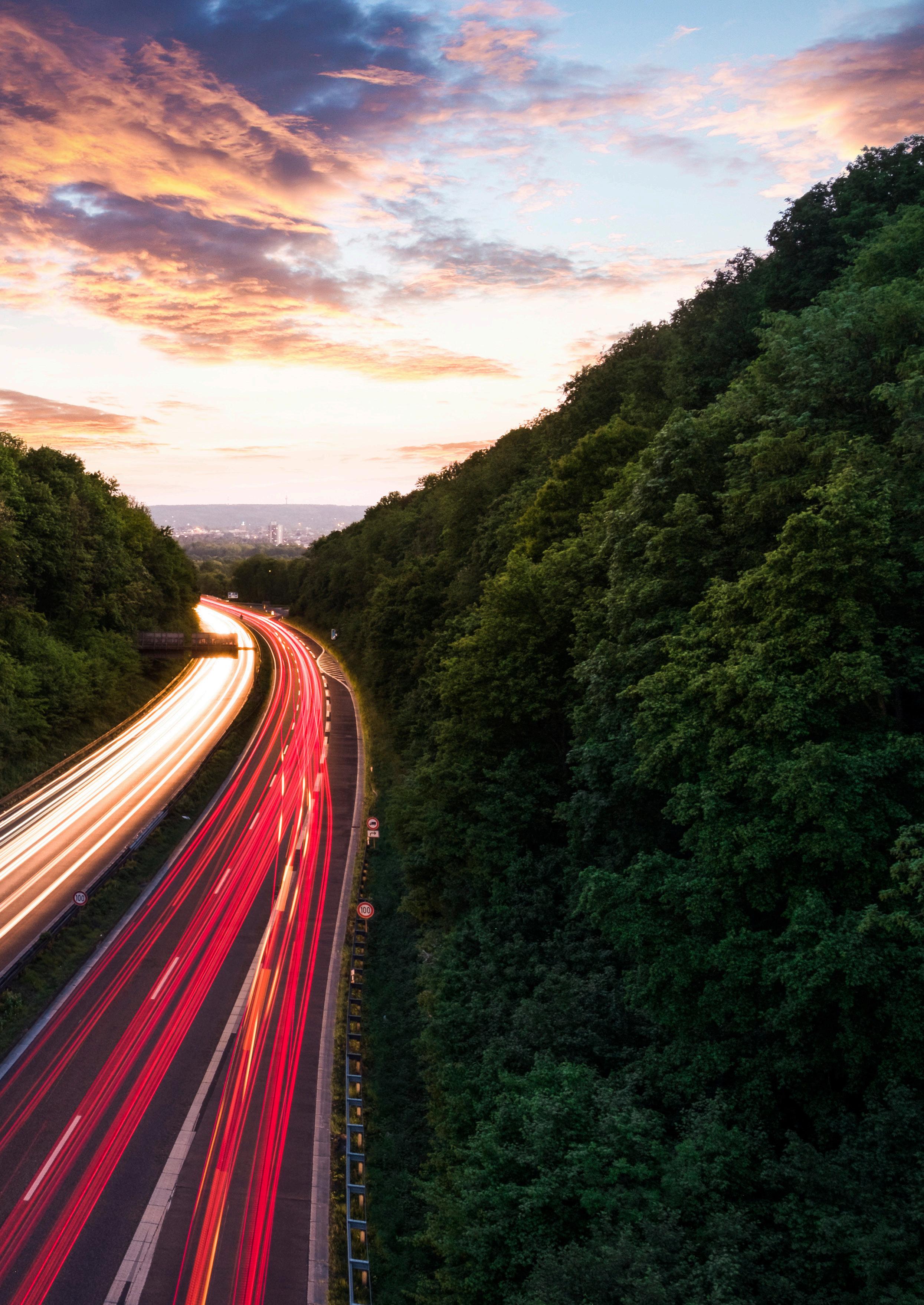
Sander van Donk, Sulzer Chemtech, Switzerland, looks at how renewable resources are key to decarbonising the transport sector and what technologies are best suited to ensure a reliable supply of quality fuels.
Electrification is the predominant trend in the decarbonisation of passenger cars, which currently require 25 million bpd. Nonetheless, this alternative does not represent a feasible option, at least in the short term, to reduce the emissions of haulage, shipping, and aviation. For example, to support planes or vessels, batteries should feature a specific energy that is six to seven times higher than current solutions.3
The role of renewable fuels in decarbonising the transport sector
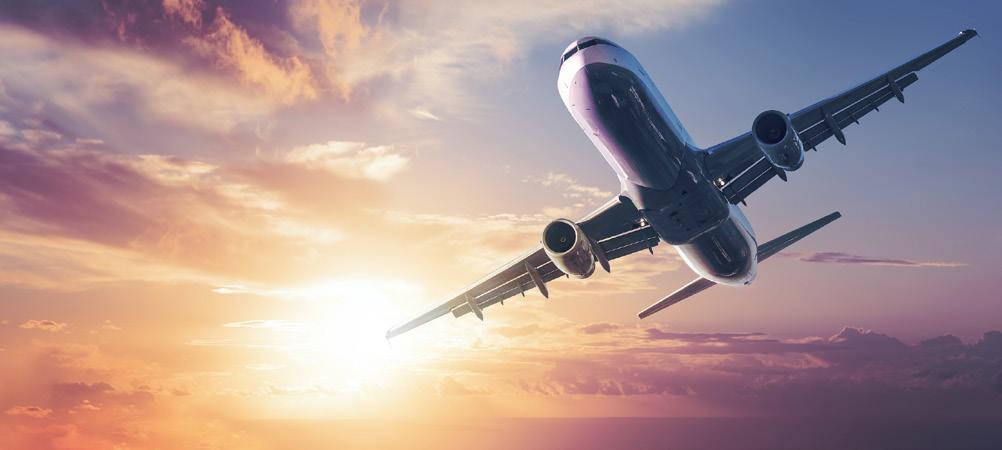
Currently, the only fossil fuel alternatives that are able to support any means of transport on a commercial scale and can compete with the costs of traditional energy sources are renewable liquid fuels. These currently provide approximately 4.2% (4.2 EJ) of the energy consumed by the transport sector worldwide.4 Belonging to the category are a variety of solutions, from bioethanol to renewable diesel (also known as hydrogenated vegetable oil [HVO]), biodiesel (or fatty acid methyl ester [FAME]) and sustainable aviation fuel (SAF).
The production methods and technologies for all these have been evolving over time. The first generation of biofuel production plants have been relying on food sources, namely sugar, starch, or oilseed-crops. These provide homogeneous feedstocks that are relatively simple to convert and purify. However, the resources used are mostly from edible plant materials, putting the production of alternative fuels in direct competition with the food industry.
In effect, these take up land, water, and other resources that could otherwise be devoted to food production while reducing potential supplies for human and animal consumption. In addition, first-generation biofuels could increase the price for the processing of sugars, oils, and other products. To avoid this, different international regulations promoting renewable energy production and use put a cap on alternative fuels from edible crops, such as the 7% limit imposed by the EU’s Renewable Energy Directive (RED).5
Driving innovation in alternative fuel manufacturing
Second- and third-generation processing plants, as well as other innovations in renewable fuel production, are focusing on non-edible plant biomass, waste animal fats, or vegetable oils
to provide more sustainable energy sources for the transport sector. These are believed to be able to address future demands, as it is estimated that nearly 100 EJ of sustainable biomass supplies are available, which could support up to 50 EJ of liquid renewable or biofuel production.6 Even more, new ventures are also expanding beyond purely bio-based or renewable fuel production, exploring how to drive more circular practices, turning non-recyclable, end-of-life plastics into hydrocarbons for energy generation.
While the transition from first-generation biorefining to more eco-conscious processing is highly advisable and promoted worldwide, the new types of feedstocks are typically more heterogeneous and complex, thus they can be more difficult to convert into fuels. Therefore, it is more challenging to balance decarbonising efforts and market requirements, delivering high-quality products while maintaining highly efficient and sustainable processes.
A premier example of the differences in processing requirements when handling alternative materials is offered by HVO/sustainable diesel and SAF, whose production skyrocketed from 80 000 t in 2010 to over 8 million t in 2021. Just like conventional fossil fuel-based feedstocks, these require hydrotreating, but typically contain higher concentrations of olefins and heteroatom contaminants. Therefore, the traditional two-phase, trickle-bed design of conventional refineries may be less adequate to accommodate these differences.
In effect, olefin- and heteroatom-rich materials require 2 – 3 times higher quantities of hydrogen to obtain high octane or cetane numbers. Furthermore, their hydrotreatment involves a highly exothermic process that generates considerable heat, reaching over 200˚C. This, in turn, increases the risk of catalyst coking, over-cracking, as well as deactivation and runaway reactions.
Supporting new processing needs
The issues presented by alternative feedstocks require the use of specific equipment by new and existing hydrocarbon processors. Only by doing this, can they successfully address these aspects and efficiently deliver cost-competitive, quality products. A solution that is designed to support companies in the adoption of bio-based or more circular raw materials for fuel production is BioFlux®, developed by Duke Technologies and licensed globally by Sulzer Chemtech.
This hydrotreating technology optimises HVO/sustainable diesel and SAF manufacturing by featuring processes, units, and components, as well as offering operating conditions that are best-suited to alternative feedstocks. More precisely, it accommodates a considerably higher liquid flux, compared to conventional hydro-processing frameworks, to completely dissolve hydrogen within the liquid feedstock, ultimately reducing hydrogen consumption. In addition, the internals facilitate the homogeneous mixing and even distribution of the feedstock and hydrogen. This capability supports complete catalyst wetting, increases the ability to effectively manage the reaction zone temperature, and reduces the likelihood of by-product formation while maximising product yield.
Even more, as the process uses treated oil to deliver more hydrogen to the reactor and to stabilise
temperature fluctuations, it does not require large volumes of hydrogen recycle gas as a quench fluid. Without any recycle gas, companies can use a cost-effective, less energy-consuming, low-pressure separator rather than a conventional hot or cold high-pressure system.
In addition to olefin saturation and hydrodeoxygenation, processors can also incorporate BioFlux’s isomerisation and selective cracking train to improve the properties of the paraffinic products obtained. Following this step, or directly after hydrotreatment, product recovery occurs through distillation.
BioFlux technology and its components can help users achieve high throughput and recovery rates. More specifically, they are expected to be able to produce one barrel of renewable diesel from each barrel of feedstock. Even more, the quality of the end products is compliant with current international standards, such as No 2 Grade of the US ASTM D975, as well as the European EN590 and EN15940 for standard diesel and paraffinic diesel fuels, respectively. Besides, processors can achieve these grades while maintaining low operational and capital expenses, ultimately reducing the price of alternative fuels. In effect, BioFlux has been recognised as one of the most cost-effective hydrotreating technologies by an independent techno-economic report from NexantECA.7 Finally, the lower hydrogen and energy requirements of the solution can limit the carbon intensity of alternative fuel production, with emissions reduced by 7 – 11% compared to conventional hydrotreating units.
Case study: Supporting winterised renewable diesel production
One of the most important applications of BioFlux is currently being implemented in Map Ta Phut, Rayong, Thailand. There, Sulzer Chemtech is helping Energy Absolute (EA), one of the biggest companies in Thailand for renewable energy supply. The technology will enhance the quality of the renewable diesel produced to meet the regulations on winterised diesel use in cold, wintery conditions in North America and Europe. It will also help EA produce SAF with maximum flexibility in the specific ratios of HVO/SAF end products. Therefore, the project will expand the company’s manufacturing plant, which offers an annual capacity of 43 000 t, and drive competitiveness.
References
1. TETER, J., ‘Transport’, International Energy Agency, (September 2022), www.iea.org/reports/transport
2. ‘World Energy Outlook 2022’, International Energy Agency, (October 2022), www.iea.org/reports/world-energy-outlook-2022
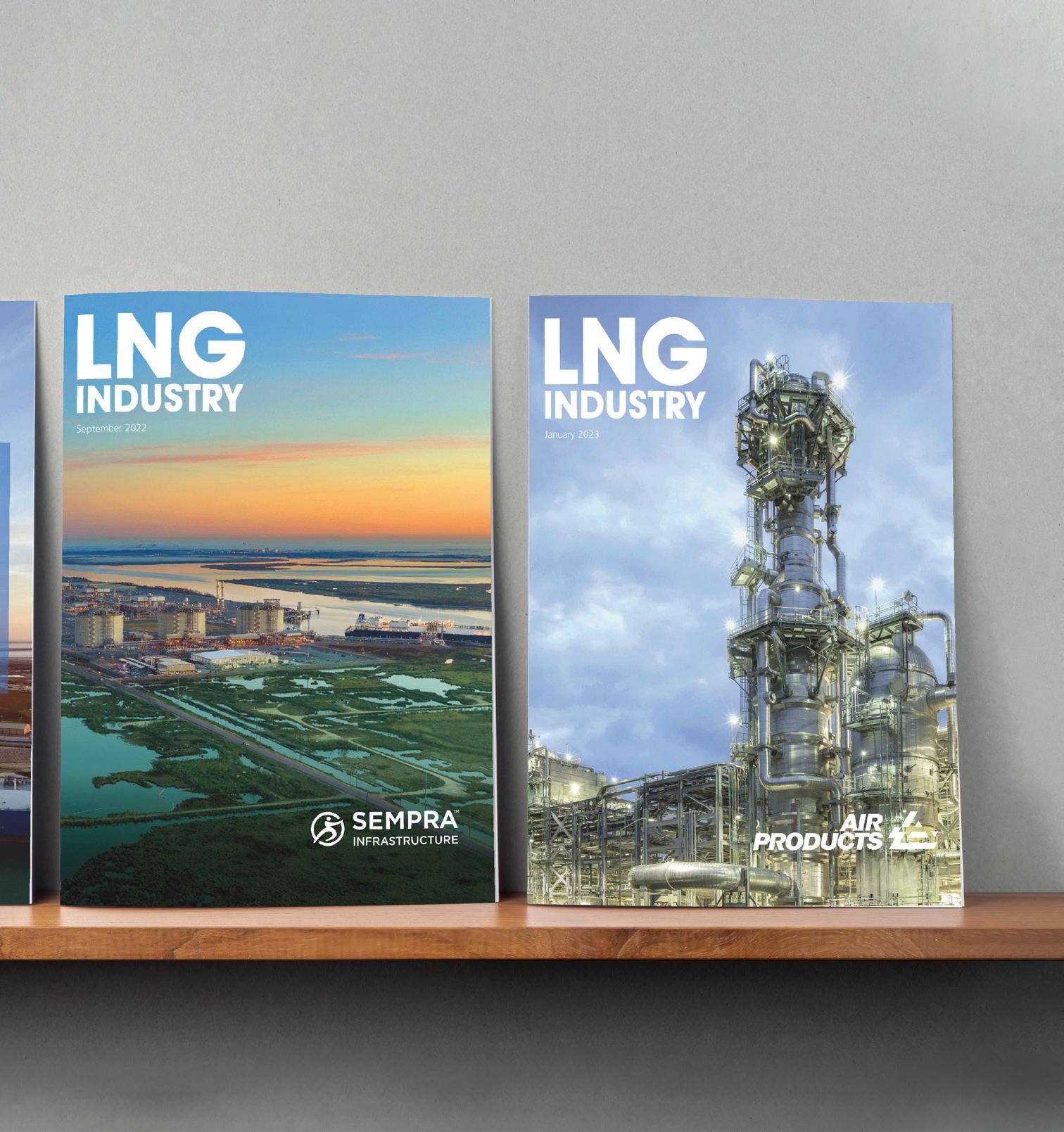
3. GRAY, N., MCDONAGH, S., O’SHEA, R., SMYTH, B., MURPHY, J. D., ‘Decarbonising ships, planes and trucks: An analysis of suitable low-carbon fuels for the maritime, aviation and haulage sectors’, Advances in Applied Energy, Vol. 1, (23 February 2021).
4. DAVIS, S. J., LEWIS, N. S., et al., ‘Net-zero emissions energy systems’, Science (29 June 2018).
5. ‘Directive (EU) 2018/2001 of the European Parliament and of the Council of 11 December 2018 on the promotion of the use of energy from renewable sources (recast) (Text with EEA relevance)’, Official Journal of the European Union, (21 December 2018).
6. ‘Net Zero by 2050’, International Energy Agency, (May 2021), www.iea.org/reports/net-zero-by-2050
7. ‘Biorenewable Insights – Hydrogenated Vegetable Oil (HVO) (2022 Program)’, NexantECA, (November 2022), www.nexanteca.com/reports/biorenewableinsights-hydrogenated-vegetable-oil-hvo-2022-program
eothermal energy has been gaining momentum as the world transitions from fossil fuels to renewables in the battle against climate change. Over the past year, several organisations –including the U.S. Department of Energy (DOE) – have released major reports citing its potential,1,2,3,4 and in January 2023, the DOE announced US$74 million for research toward enhanced geothermal systems.
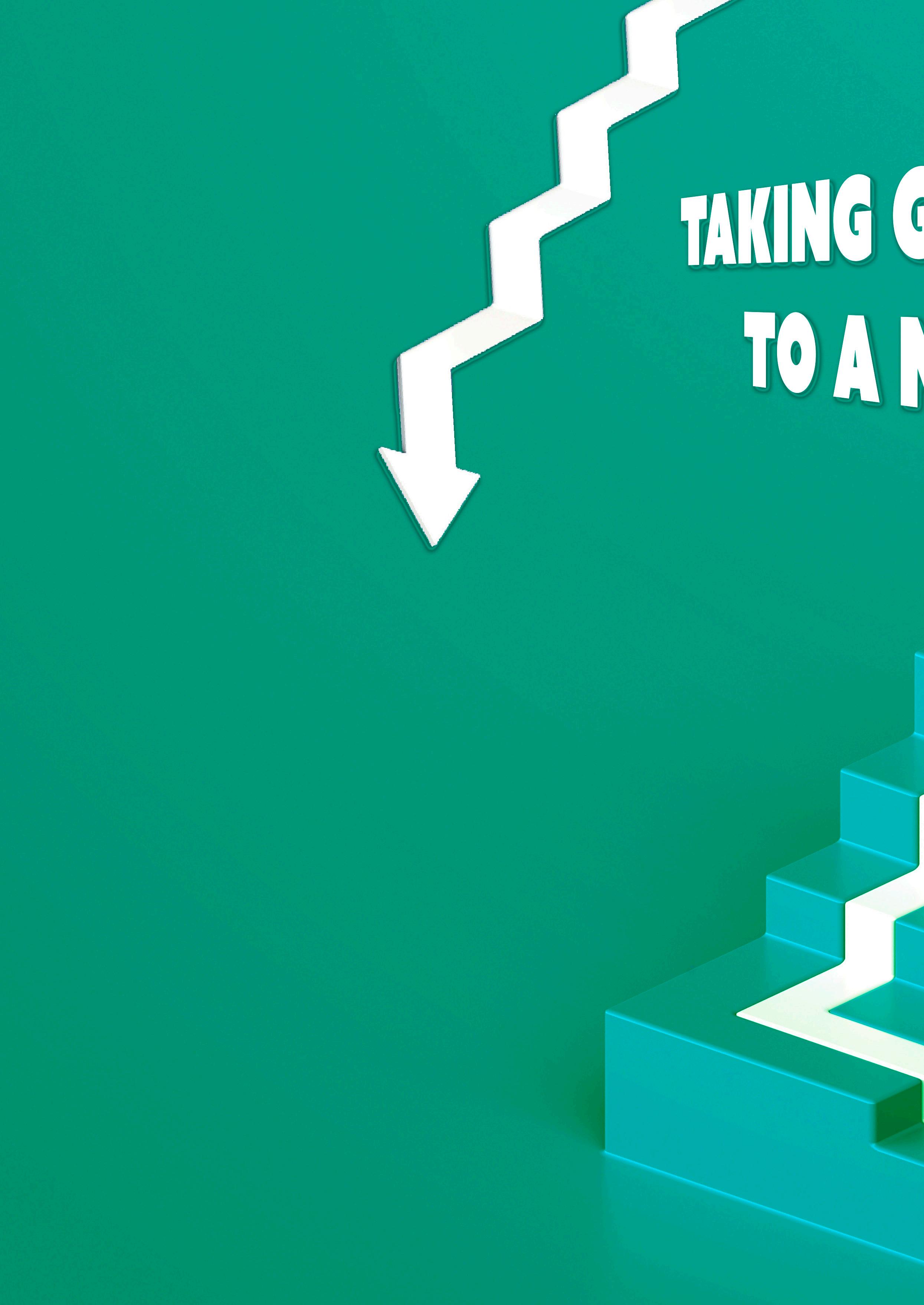
The total energy content of the heat beneath people’s feet exceeds the world’s global annual energy demand by a factor of a billion. That makes geothermal energy the most powerful and abundant renewable clean energy source on Earth, much more so than wind, solar, nuclear, and all fossil fuels combined.
Geothermal has other significant advantages. It can operate 24/7, which makes it a great complement to intermittent sources of power such as solar and wind. It also has the smallest land ‘footprint’ of any other renewable because most of its key components are underground. Similarly, geothermal has a relatively small mineral footprint, minimising the consumption of raw materials that are not evenly distributed across the globe.
Geothermal is also the perfect energy source to take advantage of the largest energy workforce in the world: the oil and gas industry. The professionals in that sector have a skill set that is exactly what is needed for geothermal to scale. Finally, geothermal could be tapped from any location in the world, making all countries energy-independent, erasing many current geopolitical conflicts, and levelling the energy playing field for all.
Toward superhot rock
The problem is that right now the mother lode of geothermal energy cannot be accessed. It is in superhot
rock approximately 5 – 20 km beneath the planet’s surface. There, conditions are so extreme – with temperatures over 374˚C and pressures more than 220 times atmospheric pressure – that water pumped through the rock would transform to the supercritical state, a steam-like phase that most people are not familiar with (familiar phases are liquid water, ice, and the vapour that makes clouds).
A geothermal well flowing supercritical steam at 400˚C can yield 5 – 10 times more power than a typical geothermal well producing hot water at 200˚C. That makes it an extremely efficient energy source, with power densities similar to fossil fuels’ if it could be pumped above ground to turbines that convert it into electricity. The result: a step change increase in the power produced per well, which could make superdeep geothermal cost-competitive with both other renewables and fossil fuels such as natural gas and coal.
Today, those supercritical conditions can only be accessed in places such as Iceland and the Yellowstone area in the western US, where such heat is relatively close to the surface.
Conventional drilling technologies – the mechanical bits and hammers for rock destruction
Matt Houde, Carlos Araque, and Kevin Bonebrake, Quaise Energy, USA, detail how accessing the superhot rock deep beneath the ground could help power the world.
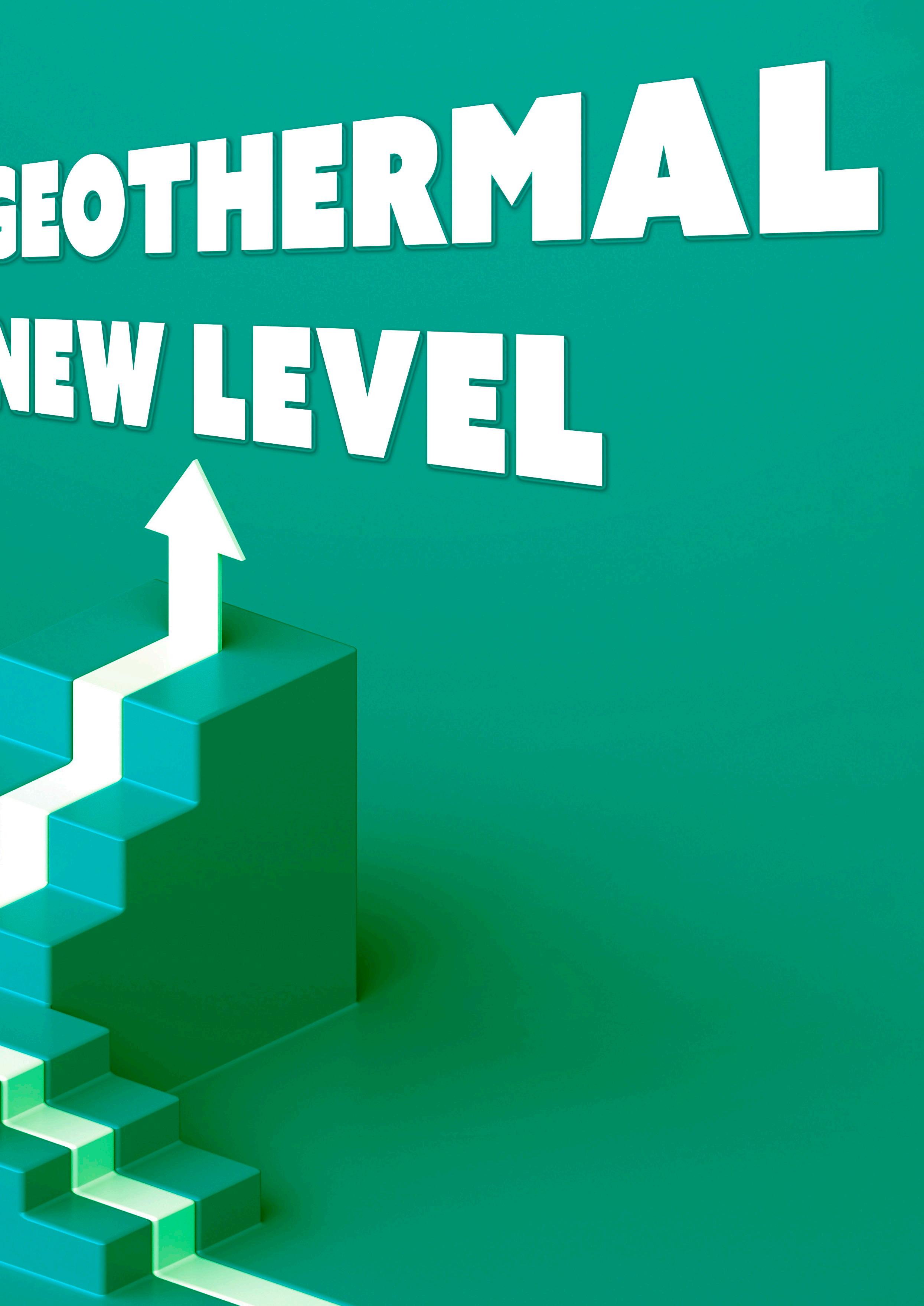
– cannot withstand the formidable conditions more than approximately 3 km down. The rock encountered at those depths is much harder and hotter than the softer sedimentary formations nearer the surface. As a result, conventional drilling typically becomes much slower as it hits these more-abrasive rocks that are also quite hot, and it takes time to replace drill bits that have failed.
The deepest hole that has ever been drilled, the Kola borehole in Russia, went down 12 km, but took 20 years to complete. If geothermal power from superhot rock is
ever to play an important role in the energy transition, thousands, if not millions, of such wells would be necessary, and today that is financially prohibitive.
The ability to drill far down into the Earth is the number one challenge facing geothermal. Without that ability, geothermal cannot realise its full potential. It stays shallow, and it stays low temperature. Cracking that challenge, however, will open up a new frontier, analogous to when the US completed the railroad that stretches across the US continent or when man made it to the Moon.
New technologies
Enter new drilling technologies. Quaise Energy, for example, is working to replace conventional drill bits with millimetre wave energy (related to the microwaves many of us cook with). That energy heats up the rock to such high temperatures –exceeding 3000˚C – that it actually vaporises.
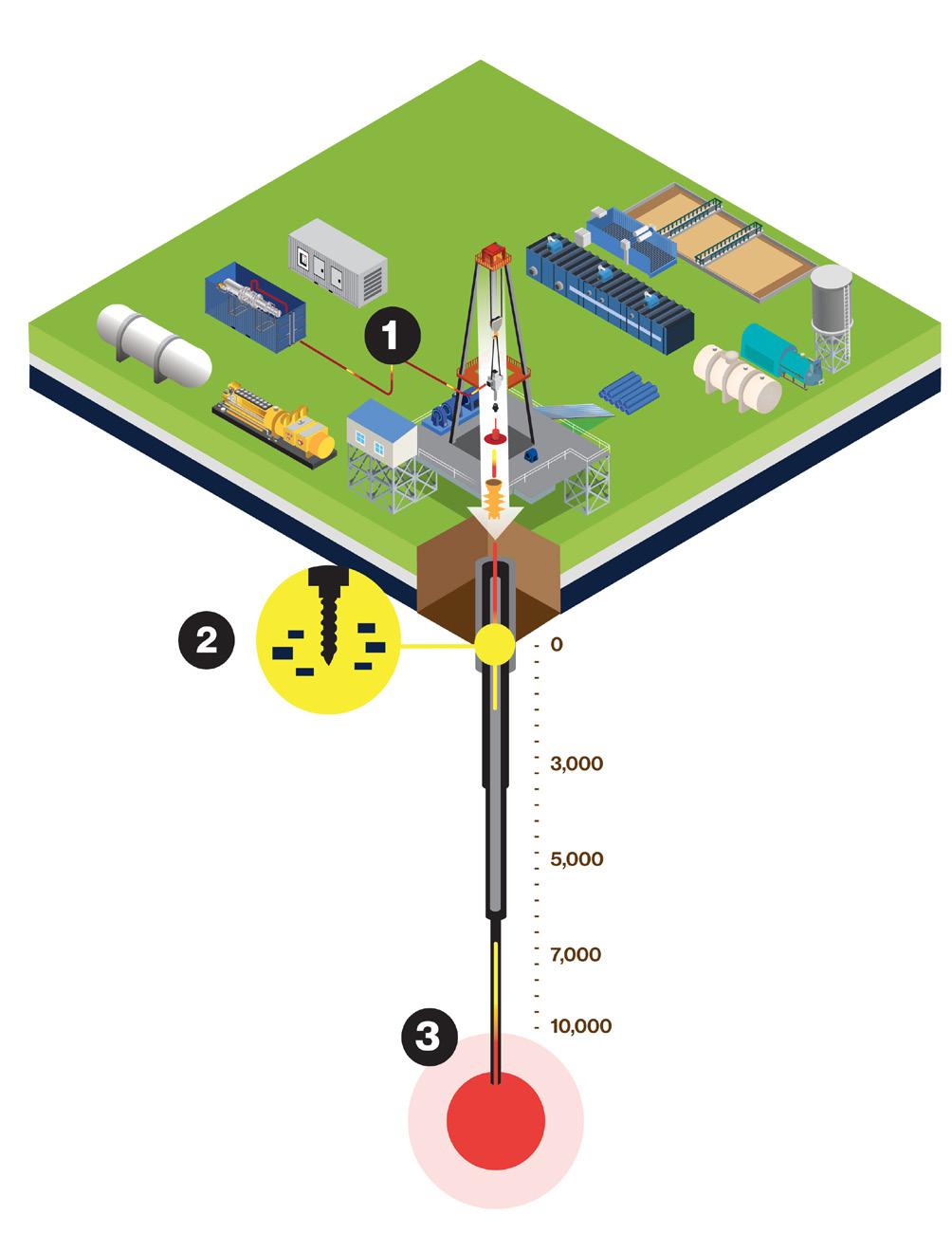
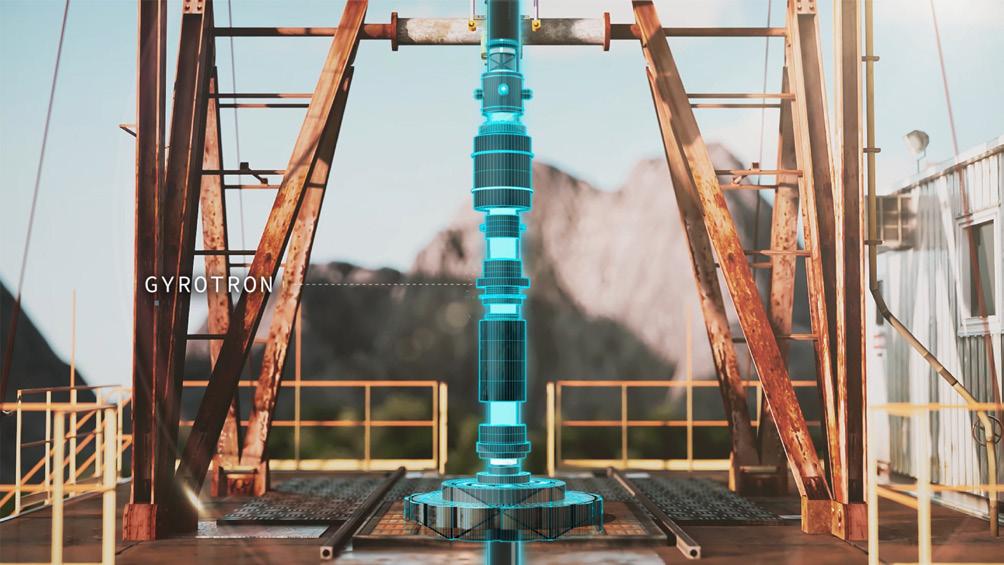
Several other companies are also developing advanced drilling technologies. GA Drilling, for example, uses plasma, an energised gas, to break deep, hard rock into tiny pieces (the GA stands for Geothermal Anywhere). Hypersciences tackles the challenge with hypersonic projectiles fired in front of a rotating bit that allow the bit to drill much faster. Yet another approach, being developed by the ORCHYD project, combines high-pressure water jetting with percussive drilling.
Case study
The general technique behind the Quaise approach was developed at MIT, where the core physics have been demonstrated over the last 15 years or so. MIT researchers have also used millimetre waves (MMWs) to drill a hole in basalt with a 1:1 aspect ratio (2 in. deep by 2 in. dia).
The main technology involved is not new. For example, the gyrotron technology (Figure 1) that produces the MMWs has been developed over 70 years for research toward nuclear fusion as an energy source. Quaise also takes advantage of the conventional drilling technologies developed by the oil and gas industry. These will be used to drill down from the surface through the sedimentary overburden – what they were optimised for – to basement rock, where MMWs will take over. Figure 2 shows a schematic of the overall drilling rig.
In addition to rock vaporisation, the MMW energy also melts an outer ring around the vaporised hole to create a strong glass ‘liner’ that prevents the hole from collapsing and protects the waveguide that serves as a conduit for MMW transmission. Once the MMW drilling kicks in, the glass liner replaces standard drilling muds to stabilise the borehole and prevent formation inflows while drilling.
How is the vaporised rock removed? Existing compressor technology is used to pump a purge gas down the waveguide, along with the MMW energy. The general set-up (Figure 3) is similar to a straw within a larger straw: the energy and gas travel downhole through the inner straw where they eventually reach and vaporise the rock at the bottom. Then the gas carrying the vaporised rock, or particulate, travels back up to the surface through the space between the inner and outer straws.
Figure 1 Artist’s rendering of the gyrotron device that is a key component of Quaise Energy’s geothermal drilling rig. The gyrotron, long used in fusion research, will produce millimetre energy waves to vaporise rock at great depths.Progress to-date and coming milestones
Last year, Quaise engineers and colleagues from industry and Oak Ridge National Laboratory (ORNL) extended the MIT laboratory results using more powerful gyrotron systems. At Quaise’s engineering facility in Houston, Texas, the US, they succeeded in scaling up the depth of a hole by a factor of 10 from the 1:1 aspect ratio accomplished in the lab at MIT to a 10:1 aspect ratio (10 in. deep by 1 in. in diameter) and a 100:1 aspect ratio (100 in. by 1 in. in diameter). Efforts are underway at Quaise and ORNL to scale up those 10:1 and 100:1 results to larger and deeper boreholes with higher operating power levels.
Scaling beyond 100:1 will require field-deployable versions of the MMW rig. To that end, Quaise is working with Nabors Industries, the largest manufacturer of onshore drilling rigs today, to integrate the MMW technology into an existing Nabors rig architecture. The goal is to develop the first full scale hybrid MMW drilling rig, which is expected to come online within the next two years (Figure 4). Finally, the company is developing a compact and more mobile truck-mounted MMW drilling rig that is intended to perform the first field demonstrations within the next year.
What about cost?
The company’s cost models indicate that its approach should enable a linear cost curve for drilling. In other words, drilling costs should remain constant regardless of depth. Conventional drilling, in contrast, gets exponentially more expensive with depth.
Assuming certain capital costs of the equipment and a MMW penetration rate of 3 – 5 m/hr, Quaise anticipates that it can drill holes deeper than 5 – 10 km and hotter than 300 – 500˚C for approximately US$1000 – US$2000/m. To do so today with conventional technology would cost more than US$5000 – US$10 000/m, and in many cases would be impossible due to the elevated rock temperatures.
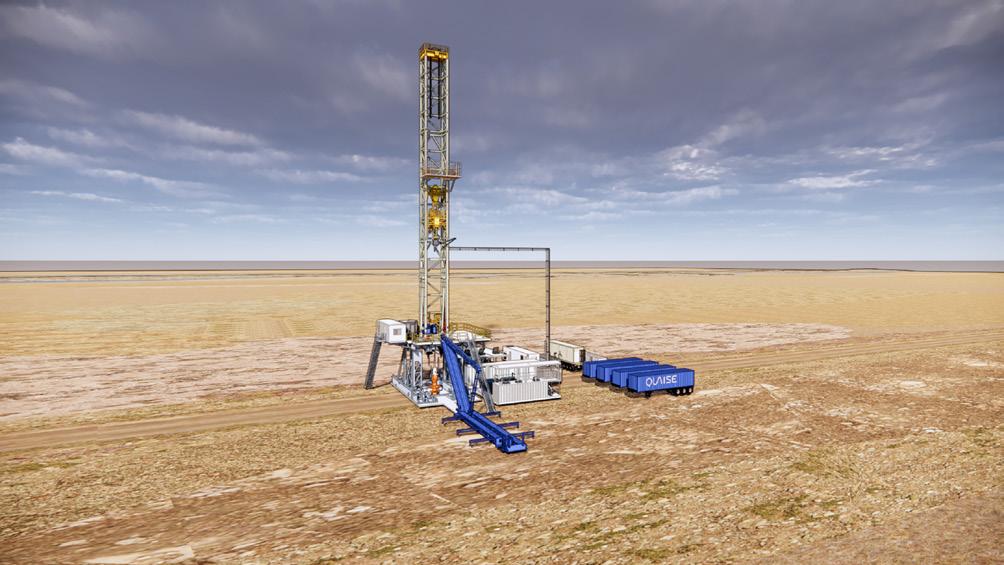
All in all, the company expects that hybrid MMW drilling should enable a levelized cost of electricity (LCOE) that can beat US$40/MWh for a greenfield development. LCOE values drop to US$10 – US$30/MWh for brownfield developments where Quaise takes advantage of existing fossil-fired power generation infrastructure and swaps a geothermal wellfield for their boilers for producing steam. The company aims to bring its pilot and first commercial applications online toward the end of this decade.
To put Quaise’s projected LCOEs in perspective, last September the DOE launched the Enhanced Geothermal Shot, part of its overall Energy EarthShots Initiative.5 The goal of the Enhanced Geothermal Shot is to cut the cost of geothermal energy 90% by 2035 to US$45/MWh. That would make the resource competitive with other renewables, such as solar (currently at US$36/MWh) and wind (US$38/MWh onshore; US$83/MWh offshore).6 It could also be competitive with fossil fuels, given increasing prices for the latter due to tighter regulations.
In sum, cracking the drilling challenge will bring the opportunity for humankind to take geothermal to an entirely new level: a hotter, more powerful, global resource with the
capacity to repower civilisation away from carbon-intensive fossil fuels.
References
1. ‘Superhot Rock Energy: A Vision for Firm, Global Zero-Carbon Energy’, Clean Air Task Force, (November 2022)
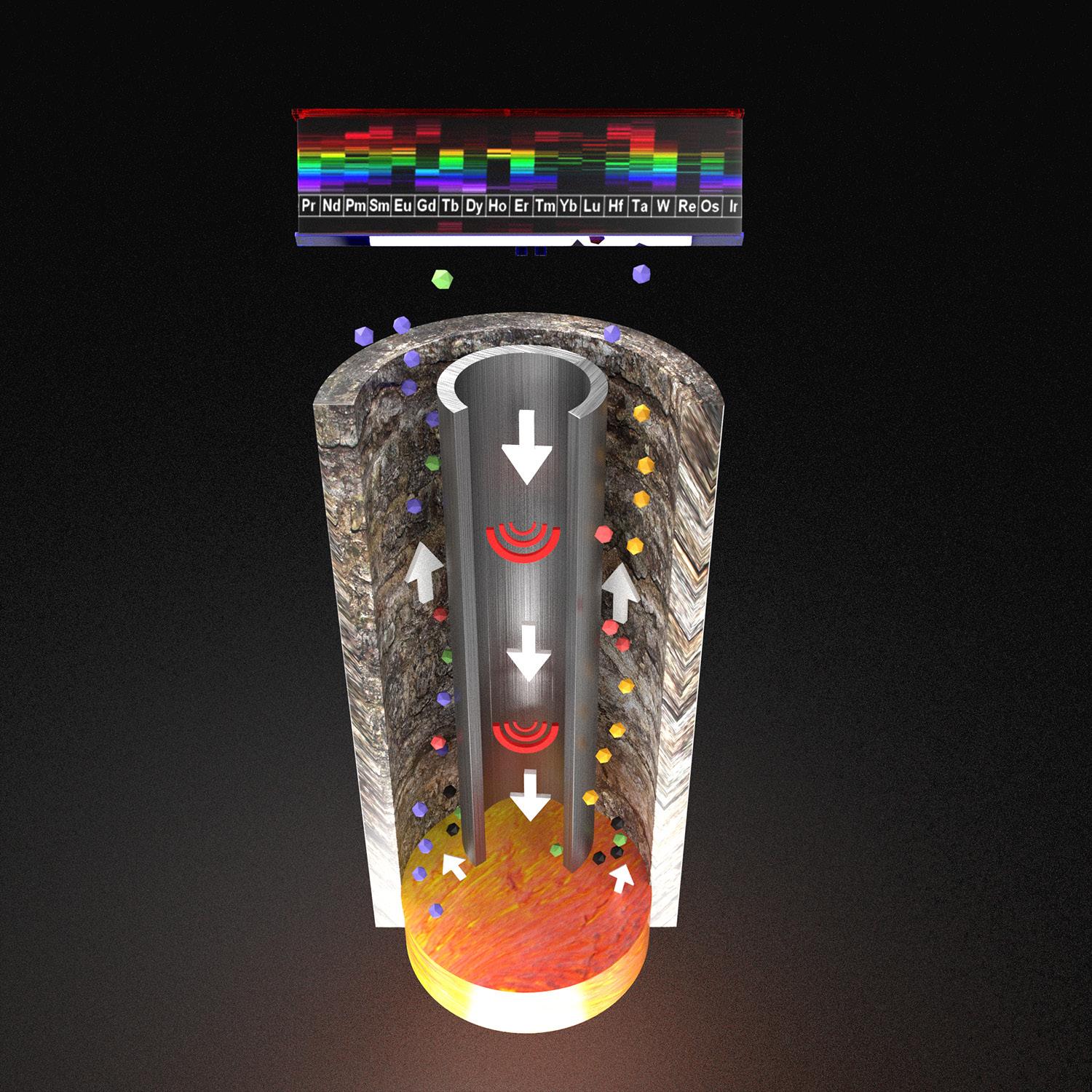
2. MAKOWER, J., ‘The State of Green Business 2023’, GreenBiz, (23 January 2023), www.greenbiz.com/article/state-green-business-2023
3. ‘The Future of Geothermal in Texas: The Coming Century of Growth & Prosperity in the Lone Star State’, The University of Texas at Austin, (January 2023), https://energy.utexas.edu/research/geothermal-texas
4. ‘DOE Analysis Highlights Opportunities to Expand Clean, Affordable Geothermal Power’, Office of Energy Efficiency & Renewable Energy, (25 January 2023), www.energy.gov/eere/articles/doe-analysis-highlights-opportunities-expand-cleanaffordable-geothermal-power
5. ‘Energy Earthshots Initiative’, Office of Policy www.energy.gov/policy/energy-earthshots-initiative
6. ‘Lazard’s Levelized Cost of Energy Analysis – Version 15.0’, Lazard www.lazard.com/research-insights/levelized-cost-of-energy-levelized-cost-of-storageand-levelized-cost-of-hydrogen-2021/
Figure 3 . Schematic of the novel drilling technology being developed by Quaise Energy. Image shows how millimetre waves and a purge gas travel from the surface to the bottom of the well inside the waveguide. Once there, the waves vaporise the rock and the gas blows those vapours back to the surface outside the waveguide.GLOBAL NEWS
SeaRenergy joins Iberdrola on Baltic Eagle offshore wind farm
SeaRenergy has been awarded by Iberdrola for the Baltic Eagle offshore wind farm in the German Baltic Sea for two different service scopes. SeaRenergy provides inspection services, maintenance, and repair works on monopiles and transition pieces as well as client representatives for all phases of the installation of Baltic Eagle.
The execution of the project scope started in April 2023 and will continue until the end of 2024. SeaRenergy’s client representatives are involved in the installation of the monopiles and the transition pieces (TP), and will further support the seabed preparation, the cabling works and the the final installation of wind turbines, including towers and nacelles. The service includes all necessary inspection, maintenance, and repair work during the windfarm’s construction phase as well as the creation of risk assessments and method statements, handling tool and equipment logistics, project management, site co-ordination and deployment of qualified offshore professionals.
Located in the German Baltic Sea, northeast of Rügen island, the Baltic Eagle offshore wind farm of Iberdrola Germany encompasses an area of almost 43 km2 and includes 50 wind turbines in total, which will be installed in water depths of 40 – 45 m. Once operational, the Baltic Eagle wind farm will generate clean and renewable energy equivalent to supplying over 475 000 households. Furthermore, this project will contribute to an annual reduction of approximately 800 000 t of carbon dioxide emissions.
Freja Offshore submits application for Swedish offshore wind farm
Hexicon, a project-developer and technology provider within the floating wind industry, has announced that the joint venture with Mainstream Renewable Power, Freja Offshore, has submitted an application for an offshore wind farm in the Baltic Sea named Cirrus, just off the south coast of Sweden. With its potential to deliver 10 TWh/y, Cirrus can provide renewable and cost-effective energy to over 2 million households annually, or the equivalent of 500 000 single-family Swedish homes.
The location for Cirrus has been carefully selected based on the relevance of energy demands and has been meticulously designed to minimise any disturbances and seamlessly co-exist with other vital societal concerns, particularly addressing the needs of other users of the sea such as the Swedish Armed Forces. Considering the expected substantial surge in electricity demand in Sweden, specifically in the southern region, the wind farm will play a crucial role in effectively meeting the growing need for electricity. By boosting renewable energy production, Cirrus can reduce energy costs, generate more employment opportunities, and foster economic prosperity in the region.
Cirrus is planned to be an offshore wind farm with bottom-fixed turbines, but Freja Offshore will also investigate the potential use of floating wind turbines for the deepest sections. The offshore wind farm will however still be beyond the horizon and just barely visible during clear weather conditions as it will be situated 50 km off the coastline.
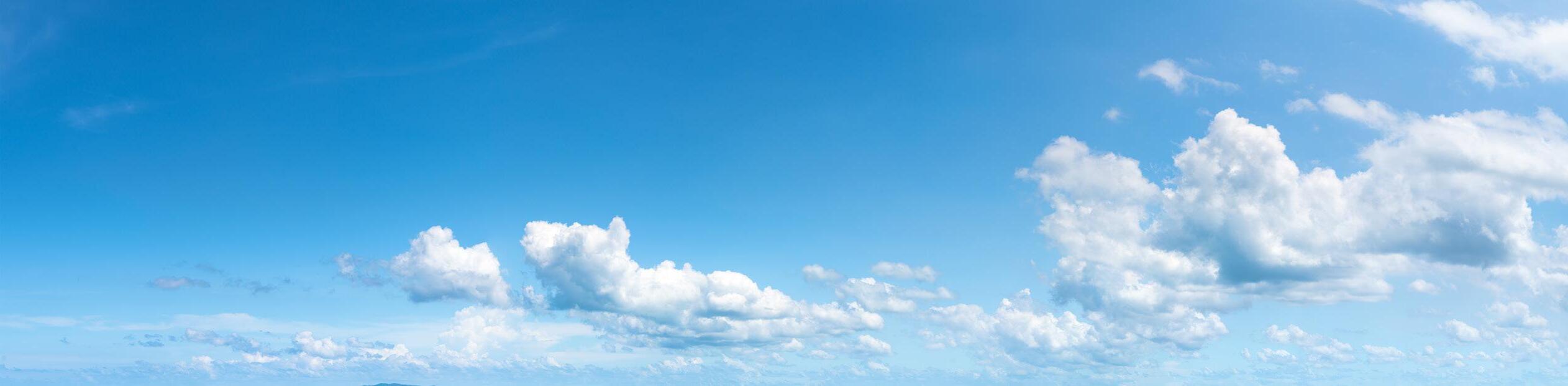
ScottishPower to repower Scotland’s first commercial wind farm
After almost 30 years of service, Scotland’s first commercial wind farm has been powered down in preparation of the site being supercharged to help meet the UK’s Net Zero targets.
Operational since 1995, ScottishPower Renewables’ Hagshaw Hill wind farm in South Lanarkshire generated more than 895 MWh over its 28-year lifespan. Now, it is about to undergo a process of repowering, becoming home to 14 new wind turbines with a combined capacity of over 79 MW. Once complete, the wind farm will be capable of producing around
five times the amount of clean, green energy than before, from just over half the number of turbines.
The Hagshaw Hill repowering project is part of ScottishPower Renewables’ wider ambition to maximise the efficiency of its existing sites by replacing older turbines with new, more powerful and efficient models.
The decommissioning process will continue over the summer and into early autumn, and the delivery of the first new turbine components to site expected in May 2024. The new site should be fully operational by early 2025.
WAVE & TIDAL
GLOBAL NEWS
CorPower Ocean resumes C4 deployment programme
Earlier this year, CorPower Ocean identified cable damage at the offshore site. It initiated a series of operations to recover and refurbish the electrical export cable involving remote operated vehicles (ROVs) and offshore construction vessels, which was successfully concluded in June 2023. With the site infrastructure restored, CorPower Ocean has resumed the C4 deployment program by testing operational methods for connecting and disconnecting its C4 device. This follows the successful site installation of the UMACK anchor and subsea electrical export cables in mid-2022 and the completion of C4 assembly and successful pre-deployment check programme at the quay-side launch pad in Viana do Castelo in December 2022.
Innovative methods for pulling down and mating the C4 device to the UMACK anchor using a fully surface operated installation sequence have recently been verified. In the next phase of the programme, the C4 device will be taken through cycles of testing safety, control, and grid functions, followed by first power export to the Portuguese grid.
CorPower Ocean is currently undergoing certification of the C4 technology with DNV – a leading supervisory to the global renewables industry – to ensure reliability of components and systems in the marine environment. For marine operation methods, Global Maritime acts as Marine Warranty Surveyor on behalf of underwriters to ensure compliance with offshore standards.
The CorPower C4 features solutions that make the device naturally protected from storms and advanced control technology to amplify power capture in regular ocean conditions. The interface to the anchor has quick connections for power export and data communication to an on-land substation, where a SCADA system is used for remote monitoring and control.
Diary dates
RE+ 2023
11 – 14 September 2023
Las Vegas, USA
https://www.re-plus.com/
2023 Deloitte Renewable Energy Seminar
27 – 29 September 2023
Los Angeles, USA
www2.deloitte.com/us/en/events/energy-andresources/2023/deloitte-renewable-energy-seminar.html
Windstaller Alliance appointed to deliver tidal power FEED
Windstaller Alliance – the joint venture between Aker Solutions, DeepOcean, and Solstad Offshore –has been subcontracted to deliver the FEED for the subsea installation scope of the FloWatt Tidal Pilot to be installed offshore Normandy, France.
The study is financed by the EU programme ‘The Tidal Stream Industry Energiser Project’, known as TIGER. The TIGER project will drive the growth of tidal stream energy to become a greater part of the energy mix, with significant benefits for coastal communities.
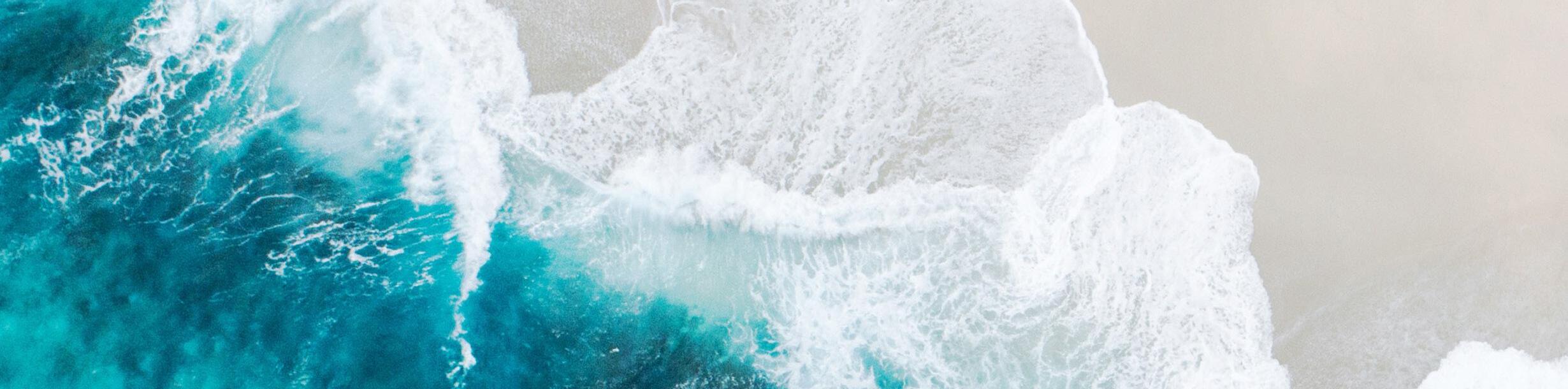
The FloWatt project incorporates seven 2.5 MW tidal turbines of Hydroquest technology – 17.5 MW in total – which will make it the world’s most powerful tidal power farm. It is located in the Raz Blanchard passage, at the northwestern tip of France, which is home to one of the most powerful tidal currents in Europe.
To deliver its FEED installation scope, Windstaller Alliance will draw upon Aker Solutions’ competence within subsea power distribution, DeepOcean’s experience from subsea power cable installation, and Solstad Offshore’s track record from installation of tidal water turbines. The FEED covers installation of a subsea HV power collection system which connects seven 2.5 MW tidal turbines to a subsea connection hub, inter-array cables, and power export cable to shore.
Windstaller Alliance will deliver its FEED installation scope as a subcontractor to Aker Solutions, which has been appointed by HydroQuest to deliver the complete FEED study for the subsea interconnection system of the FloWatt project. The FEED will be completed in the summer of 2023. The intention is to utilise the most efficient partner resources during a potential build-out of the project.
The FloWatt project is developed by HydroQuest and Qair, with a final investment decision expected in 2024.
Solar & Storage Live 2023
17 – 19 October 2023
Birmingham, UK
www.terrapinn.com/exhibition/solar-storage-live
Offshore Wind North East 2023
08 – 09 November 2023
Sunderland, UK
www.nof.co.uk/events/owne-2023/
GLOBAL NEWS
Linde signs renewable energy agreements in Brazil
Linde has announced it has signed two agreements in Brazil for the supply of renewable energy.
White Martins, Linde’s subsidiary, has entered into agreements to secure more than 2 million MWh/y of renewable energy, replacing almost half of its existing power usage in Brazil. Supply has already commenced from the Futura I solar complex, one of the largest photovoltaic plants in Latin America, and the Chuí wind farm, in Rio Grande do Sul.
The new agreements will increase Linde’s global active renewable energy by more than 60%. More broadly, Linde is on track to meet its commitment to double the purchase of low carbon energy by 2028. The agreements support Linde’s science-based absolute greenhouse gas reduction target for 2035 and its 2050 climate neutrality ambition.
In addition to reducing White Martins’ emissions in Brazil, the renewable energy will help customers to decarbonise their processes by using industrial gases with a lower carbon footprint. Linde estimates that its solutions, technologies, and services help its customers to avoid more than two times the emissions generated by all of Linde’s own operations.
ib vogt agrees to sell 98.4 MWp project portfolio in Italy
International solar developer, ib vogt, has entered into an agreement to sell a 98.4 MWp solar photovoltaic project portfolio to Rubis Photosol, one of France’s leading producers of photovoltaic energy. The portfolio is comprised of 10 photovoltaic and agrivoltaic projects in the Lazio region of Italy and has been 100% funded by ib vogt. The portfolio has been developed by ib vogt and its two joint venture partners, Christoph Drewes Energieberatung GmbH and Hans Karl Pichler.
The projects are currently under development and will be purchased on a one-by-one basis over the next 12 – 18 months as each project reaches ready-to-build (RTB) status. The first two projects to have already reached RTB status represent 25 MWp of the nearly 100 MWp portfolio and were acquired on 28 June 2023. The remaining eight projects are expected to individually reach RTB over the next year, with the last project reaching RTB by 2Q24, upon which they will be financed and developed by Rubis Photosol.
BayWa r.e. provides updates on UK solar farm projects
BayWa r.e. has announced updates in relation to its Bracks, Clump and Hill solar farm projects.
Bracks solar farm in Cambridgeshire has achieved commercial operations and is now operating. The completion of this project brings the total solar capacity installed by BayWa r.e. in the UK to just over 350 MWp. Construction on Bracks solar farm started in September 2022, and the project was sold to the UK’s Railways Pension Scheme, managed by Railpen Limited, at the end of 2022. BayWa r.e. will provide long-term O&M, as well as commercial asset management services for the solar project.
In partnership with Grüne Energien Solar GmbH, BayWa r.e. has recently received planning consent for the development of the Clump Farm and Hill Farm solar projects in Leicestershire. With installed solar capacity of 49.9 MWp and 18 MWp respectively, these projects will be able to supply up to 22 000 Leicestershire homes with green energy.
The development teams have identified opportunities to create ecologically rich environments on both sites to achieve a ‘biodiversity net gain’ through the creation of new wildflower meadows and planting new native hedgerows.
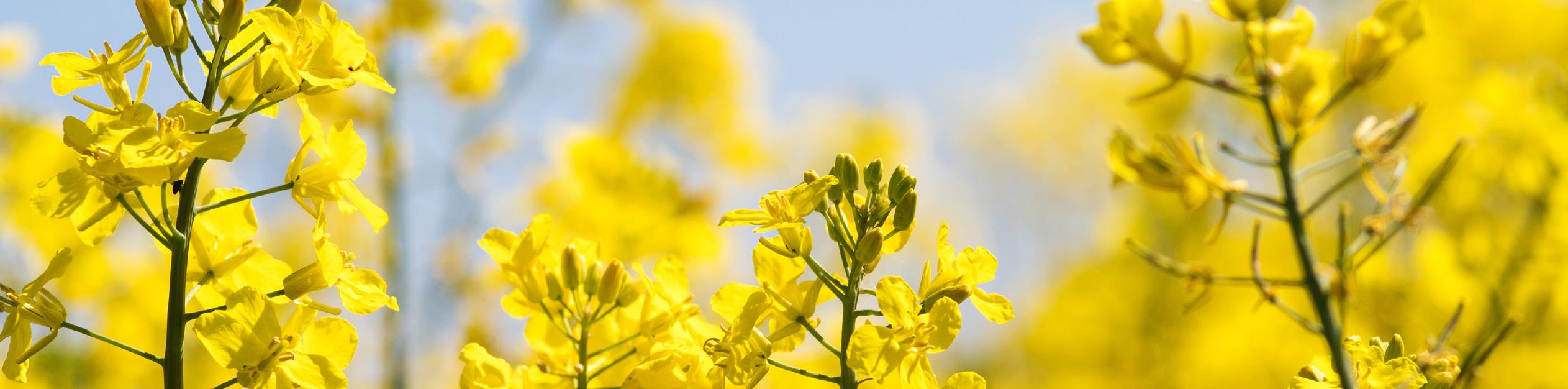
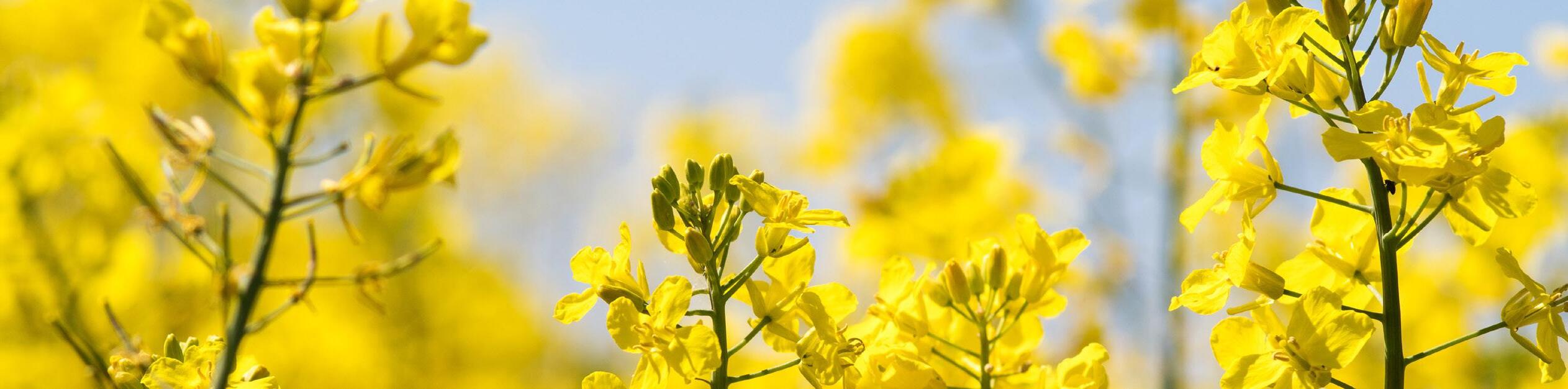
Now that consent has been granted, the Clump Farm and Hill Farm solar projects should commence construction in 2025.
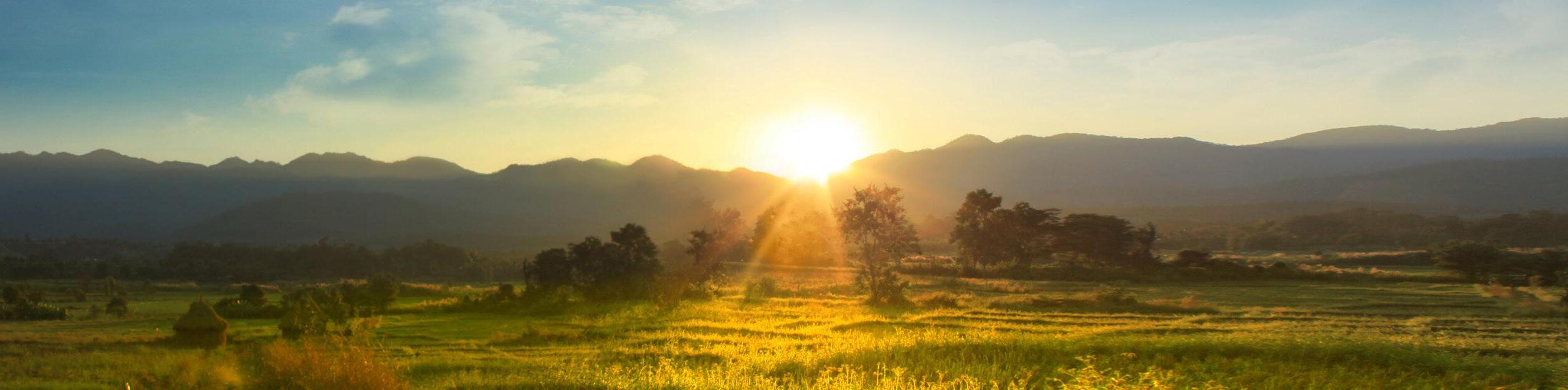
THE RENEWABLES REWIND
> Carlton Power and Superglass strike green hydrogen partnership
> AGR and Add Energy unite drilling and wells expertise
> GE Vernova to service Aurland hydropower plant
Follow
updates, industry news, and technical articles. www.energyglobal.com
GLOBAL NEWS
Cambridge Power secures BESS planning permission
Cambridge Power has secured planning permission for a 100 MW battery energy storage system (BESS) facility in Staploe, Bedfordshire, adjoining the Eaton Socon Grid Sub Station.
The site near Eaton Socon will see significant biodiversity net gains provided as part of the scheme. Extensive planting is proposed, alongside the technical infrastructure, to provide ecological benefit and landscape mitigation and achieve a very significant biodiversity net gain of 71%.
This is also the first site with planning permission that will benefit from a technical grid connection solution developed by UKPN as part of their Regional Development Programme (RDP).
The RDP’s are designed to unlock additional network capacity and reduce constraints by offering BESS a grid connection with restrictions in use based around peak demand and peak generation times on the network. They aim to introduce new ways of working that significantly enhance transmission and distribution systems coordination and control. The hope is that National Grid will adopt this successful RDP solution as part of the grid remodelling exercise currently going through consultation, enabling significant capacity to connect to the UK grid network ahead of current suggested delays.
Matrix Renewables to develop BESS in Italy
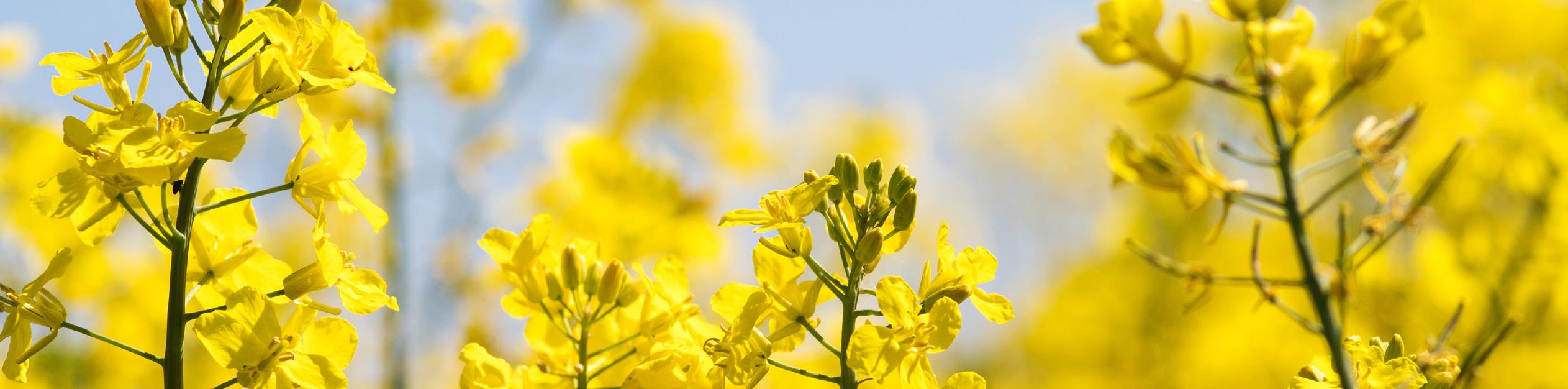
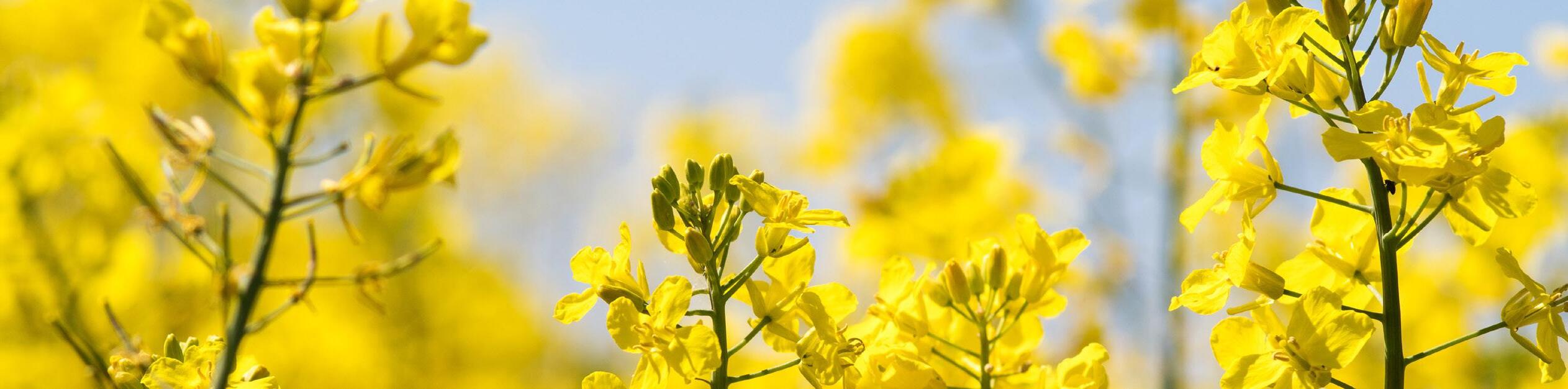
Matrix Renewables, a TPG Rise-backed global renewable energy platform, has started a partnership with Gravel A through a proprietary development service agreement (DSA) for the development of up to 1.5 GW of standalone battery energy storage systems (BESS) in Italy. The first stage of this partnership includes over 260 MW of standalone BESS projects.
Gravel A is a joint venture between Emeren Limited, a subsidiary of Emeren Group Ltd, a renewable energy developer with operations in 10 countries, Enerpoint, which is active in development and construction of large PV plants, and Kaizen Invest Holding, a private investment company in energy, real estate, and infrastructure. These well-known industry players have joined forces with Matrix Renewables to leverage their collective expertise in the renewable energy sector.
The DSA consists of a series of standalone BESS projects with different sizes and geographies, which Gravel A will develop exclusively for Matrix until they reach Ready-to-Build status.
This partnership is of high strategic relevance to help the parties reach their ambitious growth plans in Italy, and it highlights Matrix as one of the first movers in the Italian standalone BESS market.
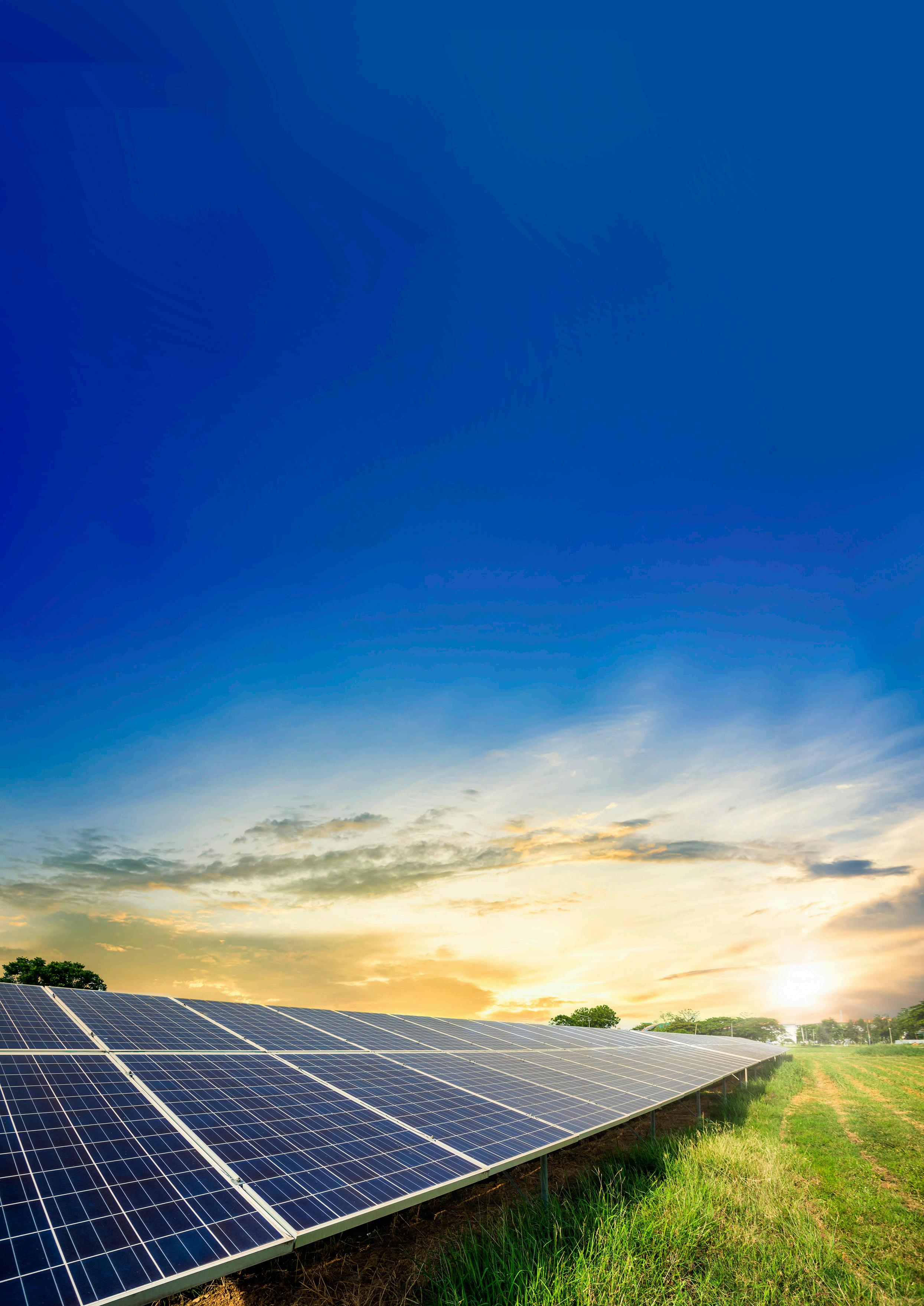