21 minute read
The effect of the curvature of a swimming paddle on the propulsion force
The effect of the curvature of a swimming paddle on the propulsion force
Jessica Hargreaves
Barker College
Purpose: This paper aims to investigate the effect of the curvature of a swimming paddle on the fluid resistance and therefore propulsion force. Its purpose is to understand the optimal curvature for the highest propulsion force to allow for optimal muscle and technical development in training. Design/methodology/approach: Swimming paddles of four different curvatures were attached via a bespoke apparatus consisting of a cable pulley system and secured before a pool swimming jet. The tensile force on the cable was measured for each manufactured swimming paddle. Findings: This investigation found that the propulsion force increased until 20° when decreased after, producing a lower reading at 30° curvature. The implication of this data is that a paddle of approximately 20° curvature has the optimal propulsion force compared to angles above and below it. Research Limitations/implications: An increase in curvatures tested would be an improvement of this investigation. Especially curvatures between 10° and 30° as the data implies that the optimal propulsion force is between these values. Practical implications: The inconsistent flow from the jet caused the paddle to move back and forth, in turn causing the force on the luggage scale to fluctuate, potentially causing inconsistent results. Social implications: This information allows for the swimming community to understand the most effect paddle to produce allowing them to be accessible and affordable to all. Originality/value: While various elements of swimmers' hand position and spread have been investigated, to the author’s knowledge, research surrounding the effect of curvature of swimming paddles on propulsion force has not been published in any form. Keywords: Swimming paddles, Muscle growth, Propulsion force, Training, Fluid resistance Paper Type: Research paper.
Literature Review
Elite swimmers often include sets in their trainings involving the use of swimming paddles which requires an athlete strapping a plastic paddle to their hand (Ogita, 1999) (See Figure 1). The purpose of the paddles is to increase the resistance of the hands as they move through the water, in turn strengthening the swimmers’ arm, shoulder and back muscles. Therefore, it is desirable to maximize fluid resistance through water for a given surface area of paddle (of similar size to a human hand).
As for all sports, there has been extensive research into the mechanics of a swimmers’ stroke where they pull their hand and arm backwards through the water to propel them forwards (Tsunokawa, 2019; Houwelingen, 2017). Due to the need to maximize pulling force, a number of these studies focus on hand position and finger spread to optimize the force produced by the stroke.
Sidelnik and Young (2006) produced a study that experimentally simulated the dynamics of the freestyle stroke in the pull-down phase by measuring the propulsive force created by two models of human hands: one with fingers held together and the other held with fingers spread at 10∘. It was determined with 95% confidence that a finger spread of 10∘ creates more stroke force than a fingers-together configuration across all pitch angles tested. This is due to the turbulence created between the fingers, in turn allowing for a larger propulsion force (Sidelnik and Young, 2006; Marinho, 2020; Vilas-Boas, 2015).
Figure 1: Photograph of man swimming with plastic paddles (Source: Smith, 2020)
Some experiments investigating both finger and thumb spread (Vilas-Boas, 2015) shown in Figure 2.
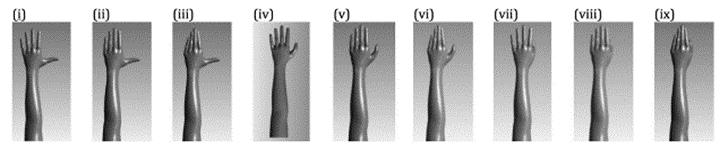
Figure 2: Hand models used in investigation of fingers and thumb spread (Source: Vilas-Boas, 2015)
The kinematic characteristics of the hand during a free-style stroke were investigated through computer simulation and recording devices (Gouroulis, Aggeloussis, Vezos, Antonoiu & Mavromatis, 2008). The hand orientation for optimal angles was found to change as the hand progressed through the stroke. It was determined that the larger the hand the more force produced, and that the angle that produces the optimal force is perpendicular (90°) to the direction the body in the water is moving, however the position of the hand with respect to the body also influences the force created to propel forwards through the water. Another investigation modelled all the orientations of the hand and the fingers as shown in Figure 3 and 4 which revealed the propulsion force for the combination of thumbs and fingers that are either separated or together (Vilas-Boas, 2015).
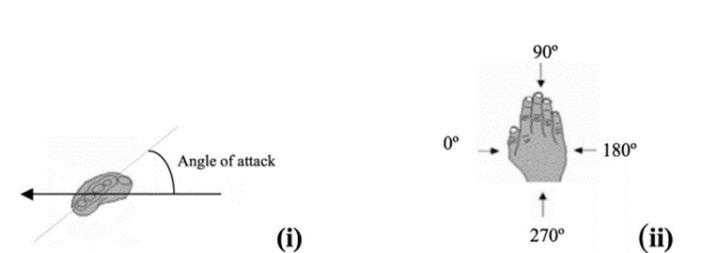
Figure 4: Hand orientation (Source: Vilas-Boas, 2015)
Other studies have focused on the use of paddles and their effect on producing the optimal force. Studies have shown that the larger the surface area of the paddles, the more resistance the paddle will have (Crocker et al., 2018, Hubb, 1991). The results from this study suggest that an optimal hand paddle size for proficient, adult swimmers is 210–358 cm2. The greater the surface area the more resistance and force that will need to be simulated to pull through the water. Therefore, the surface area of 210-358 cm2 for an average capable swimmer creates the optimal propulsion force whilst maintaining appropriate force and resistance on the swimmer’s body. It was also found that compared to free swimming, paddle

Figure 3: Results of different hand orientations (Source: Vilas-Boas, 2015)
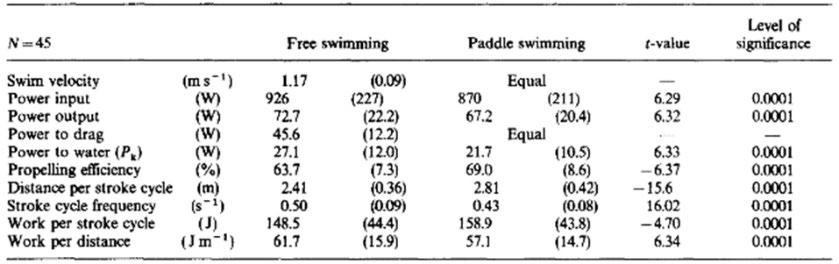
Figure 5: For both swimming free and paddle swimming, the data is given as mean (SD) of all measurements (N =45) on all subjects. The paddle and free-swimming scores were tested for differences using a two-tailed Student’s r-test for paired comparison (Source: Hubb, 1991) swimming increases your work per stroke (Minetti, 2021; Hubb, 1991) as shown in Figure 5.
The optimal shape of paddles can be drawn from a study that focus of the fluid mechanics of the paddle wheel (Hildebrandt, 2020, Peterson, 2001). The study found that cylindrical paddles created a larger propulsion force compared to cubic paddles with the same surface area.
However only certain areas of this topic have been investigated such as the shape of the paddle, size and other factors that have been acknowledged and incorporated into the deeper understanding of this topic, and therefore it is important for this investigation to consider optimization of paddles for a given surface area and in turn making the independent variable of this experiment the concave curvature of the paddle. This gap in the literature relating to optimal paddle curvatures was the motivation behind this investigation.
Scientific Research Question
What is the optimal curvature of a swim paddle, to allow for maximum propulsion force?
Scientific Hypothesis
It is predicted that the force exerted by the swim paddle will increase as the angle of the curvature increase, until a certain point, then the force will begin to decrease.
Methodology
Apparatus
The apparatus was invented during the course of this investigation for the sole purpose of testing the force of water pushing against swimming paddles. The core of the apparatus was constructed out of two pieces of wood, drilled together at right angles, one piece horizontal and the other vertical, as shown in Figure 6. Two large hooks were screwed next to each other at the top of the vertical piece of wood to hold the luggage scales. Handheld luggage scales were used as when a force pulls them down they give a reading of a mass, which can be converted to force, using the formula F=ma. A small pulley was attached near the bottom of the vertical piece of wood, making sure it was above the water level. A narrow 25cm long piece of dowel was attached to the paddles on one side to increase the stability when testing. The other side of the dowel was attached to the string that went through the pulley and attached to luggage scales
Paddles
The four paddles were made from four different colored acrylic materials. Each sheet of acrylic was cut in a laser cutter with the 26x15cm oval being cut out of each one. They were all cut the same size to keep surface area constant. Once they were cut, they were placed in the oven at 200 degrees Celsius for 20 minutes to soften. While they were in the oven, four identical pieces of aluminum sheets were curved to four different curvatures (Figure 7), the angles were measured from the tangent of the top of the curve as shown in Figure 8. The angles included were 0° (flat piece of acrylic), 10°, 20°, 30°. Once the acrylic had softened, the acrylic was laid on the different curvatures as shown in Figure 7. After 20 minutes, once cool, it retained its curvature, and the final product is shown in Figure 9.
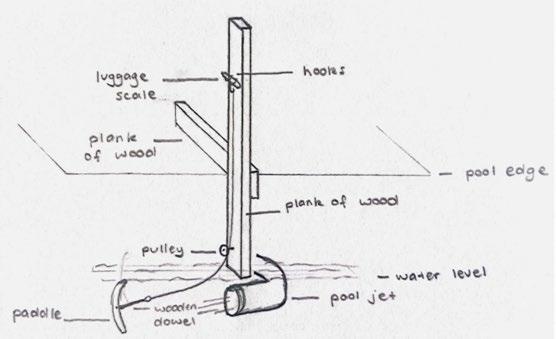
Figure 6: Experimental Setup
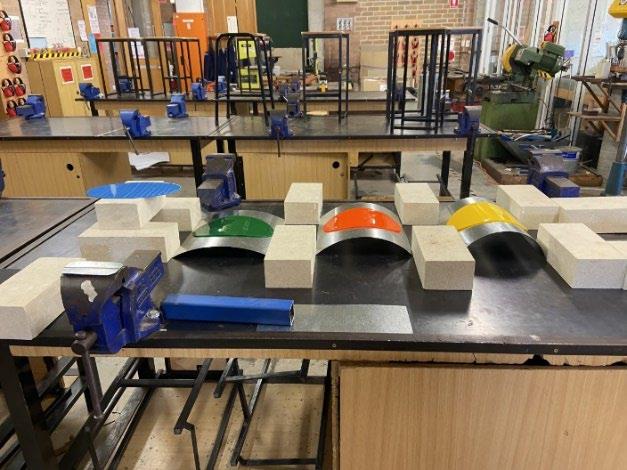
Figure 7: Paddle production of curvatures 10° (green), 20°(orange) and 30°(yellow)
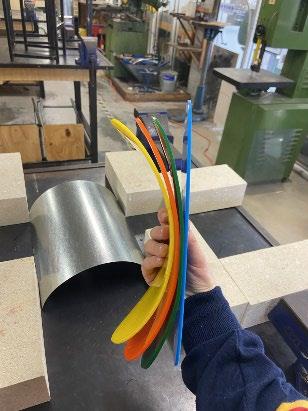
Figure 9: All four paddles made
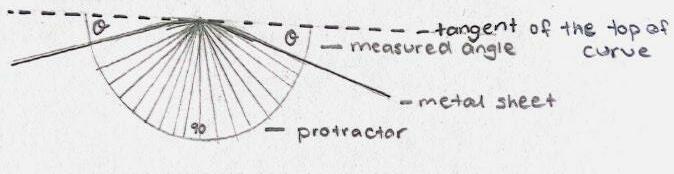
Figure 8: method to form accurate curvature
Experimentation
The experimentation process began by attaching the first paddle (0°) to the wooden dowel of the apparatus by tightly screwing the two together, as shown in Figure 6 and Figure 10. The dowel was then connected to the 1m long string that ran through the pulley and attached to the bottom luggage scale. The apparatus was set up on the edge of the pool with the vertical piece of wood directly above the mouth of the jet. Before lowering the paddle into the water, the luggage scale was zeroed, then the paddle was lowered into the water.
The force produced by the jet against the paddle caused a downwards force on the luggage scale, providing a mass reading (g). Since Force is proportional to mass, by the equation F=ma, as the mass increased, the force also did. Once a fixed mass was measured, the data was written down. This reading in grams was divided my 1000 to give kg, then times by 9.8 (acceleration due to gravity) to produce a force result.
The paddle was then taken out of the water to then zero the scale again. The experiment was repeated for 2 more trials for the 0° angled paddle, then that paddle was unscrewed from the dowel and the 10° paddle was attached. This was to keep the dowel and the string length constant for everything. The same process was completed for the 10°, 20° and 30° angled curvatures, therefore collecting three trials for each set of curvatures.
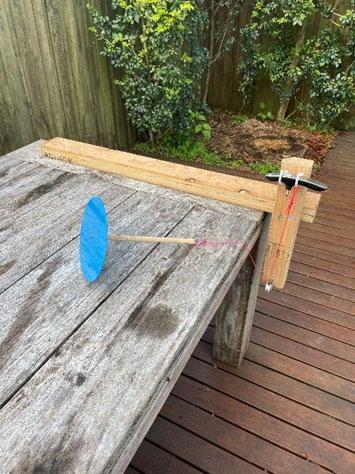
Figure 10: Fully constructed apparatus outside of the water with 0° paddle attached
Results
Table 1 shows the results for each trial and the average force of the water pushing on each curvature of paddle. The results are then presented in graphical form in Figure 11.
Table 1: The force in Newtons pushing against each of the paddles according to their curvature.
Figure 11: Graph showing the relationship between the paddle curvature and the average propulsion force
Curvature (°) Mass on scale (g) Mean Mass (g)
Mean Force Trial (N) 1 Trial 2 Trial 3
0 164 189 145 166 1.6268 10 140 168 201 169 1.6562 20 212 235 293 246 2.4108 30 190 167 207 188 1.8424
Force pushing against each of the paddles (N)
3
2.5
2
Paddle Curvature vs Propulsion Force
1.5
1
0.5
0
0 10 20 30 40 Paddle Curvature (°)
While completing the experiment there were a number of qualitative observations about the behaviour of the paddles which assisted in the interpretation of the results. These are reported in Table 2.
Table 2: Qualitative Observations of the paddles positioning in the water and effect the water flow against it had, for each curvature
Paddle
Curvature (°)
0
10
20
Observations
fell below the water flow line when unassisted was flimsy and unstable on some attempts fell below the water flow line when unassisted was quite unstable, moved around Held its position against the force of the water Stayed sturdy and movement was limited
30 Held its position against the force of the water Stayed sturdy and movement was limited
Discussion
The research question was to investigate what the optimal curvature of a swim paddle was, to allow for maximum propulsion force. The data produced through experimentation is shown in Table 1 and Figure 11. The relationship between the paddle curvature and the propulsion force in Figure 11 shows a minimal increase between 0° and 10° of only 0.0294N (1.6268N-1.6562N). Between 10° and 20° the propulsion force increases at a higher rate from 1.6562N to 2.4108N. It then began to decrease between 20° and 30°, with the paddle curvature of 30°having a propulsion force of 1.8424N. The results supported the hypothesis that the force exerted by the swim paddle will increase as the angle of the curvature increases, until a certain point, then the force will begin to decrease. This is evident in the graph in Figure 11, as there is an increase in force until 20° then it begins to decrease. Therefore, the certain point in which the force is maximized at for this experiment, is a curvature of 20°.
The conclusion drawn from these results is that the optimal curvature for a swimming paddle lies between 10° and 30°, evident in the results that show a peak between the two curvatures. This is due to the combination of curvature and surface area, which are both factors that influence the amount of water that can be held in a paddle. As a paddles curvature increases its frontal surface area decreases as shown in Figure 12. Frontal surface area is important as it allows for a larger amount of water to be pulled through when propelling through the water. Curvature is also important in making sure the water is able to be held throughout the pulling motion of the swimming stroke. This inversely proportional relationship means that the correct balance of both will result in the optimal propulsion force. The paddle curvature of 20° produces the optimal propulsion force as it’s amount of curvature effectively holds water whilst maintaining a large frontal surface area. As observed in Table 1, there is a lot of variation in the raw data used to make the averages. Whilst 20° is clearly higher than the others for all three trials, there is a lot of fluctuation amongst the others suggesting a lack of reliability in single tests. Therefore, three trials allowed the average of the trials to be used as a better representation of the data.
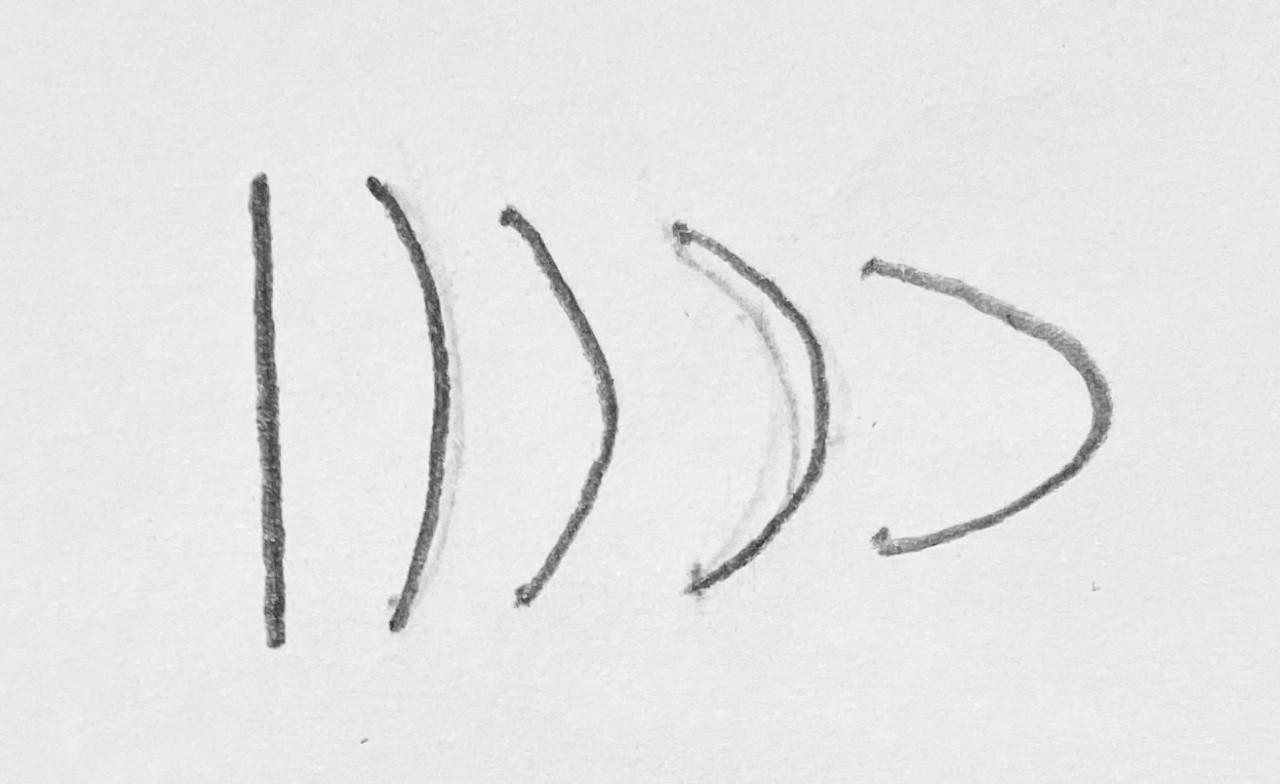
Figure 12: Different curvatures producing different frontal surface areas with same sized paddle
While conducting the experiment, observations (in figure 15) highlighted the positioning of each paddle and how they reacted to the force of the water against it as shown in figure Curvature angles of 0° and 10°, when placed in the direct flow of the water, sunk below the flow as shown in Figure 13, causing the water to not directly contact the paddle. This was caused by the inability to hold water in the paddle, which is important for swim paddles as they must hold as much water as possible to then maximize the pull through the water. These two curvatures were also unstable and did not hold their position in the water, reiterating that the lower the curvature the less water that is able to be held in the paddle, in turn minimizing the stability of the paddle. Due to the instability of these paddles, when testing they had to be lightly held up to make sure that the water flow was directly hitting the paddle. They were held directly up with a piece of string around the wooden dowel, only being pulled in the upwards direction, not away or towards the apparatus to minimize the effect on the results.
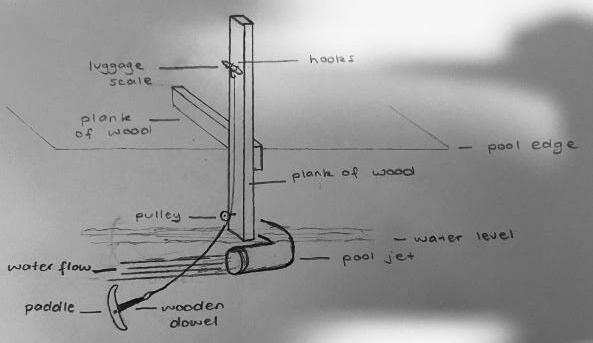
Figure 13: Paddle sinking below the water flow
As the curvatures increased the stability in the water increased as both the 20° and 30° paddles were able to stay stationary while being in the direct line of the water flow. This supports the concept that higher curvatures have the ability to hold water better, which is desirable when wanting to find a curvature that gives the optimal propulsion force.
The methodology of this investigation had limitations that restricted the overall outcome and results of the experiment. The amount of curvature angles measured and tested was limited to four in this investigation, reducing the overall knowledge of the relationship between the curvature of swim paddles and propulsion force. The limited number of curvatures made it hard to understand the trend, and other information relating to this experiment. This could be improved by including curvatures at 1-2 degree increments between 10 and 30° to gain a better understanding of the relationship.
Only three trials for each paddle were conducted throughout the experiment. This small sample size could misrepresent the correct and valid data. This could be improved by adding further trials in to remove outliers and gain valid and reliable data. The raw data received from this experiment varied a lot, making averages even more important as all of the raw data was so different.
Another limitation was the inconsistent flow of the water. The pool jet used in this investigation was dependent on the amount of water in the pool. For example, initial pre-tests were conducted when the water levels were low, resulting in the water flow being inconsistent and unreliable as it was causing the paddles to move back and forth, which produced unreliable results. The experiment was repeated, and final results obtained when the water levels were high again, which reduced the inconsistent flow, however it was still not flowing at a completely constant rate. To improve this, the experiment could be conducted in a flow tank or against a jet that produced a constant flow rate.
Another limitation of the experiment was that the paddles with curvatures of 0° and 10° had to be held up by a piece of string which may have affected the results for those two curvatures. This could be improved by doing this to all four of the curvatures to make the assistance constant throughout the entire experiment. Conducting the experiment in a flow tank may also address this limitation.
While a constant stream of water colliding with the paddle was an effective substitute for force output from a moving paddle, another expansion from this experiment could be investigating the curvature by pulling the paddle through the water using a weight and pulley system. This would simulate the exact movement of a paddle when it is being used by a swimmer.
Future projects relating to this investigation could include experimentation of the curvatures between 10° and 30° in smaller increments to find a more accurate value for the optimal curvature for a swimming paddle.
Conclusion
In conclusion the experimental data supported the hypothesis that the force exerted by the swim paddle will increase as the angle of the curvature increase, until a certain point, then the force will begin to decrease. The data showed that the optimal curvature of a swimming paddle was approximately 20 for maximum propulsion. This was found through experimentation using a pulley-based apparatus to measure the force exerted on the paddle when in direct contact with water flow. While there was a clear maximum around 20°, further experimentation could increase the precision and reliability of the result including a greater variety of paddle curvatures, a higher volume of testing, and testing using various alternative apparatus.
Acknowledgments
I would like to thank Dr Matthew Hill for encouraging me to dive deeper into my research and explore an area of real interest to me. Thank you for regular conversations about the science process and troubleshooting ideas about the apparatus.
I would also like to thank Mr Tim Grimes (D & T teacher, Barker College) for assisting and guiding me through the design and production process of creating my four acrylic paddles that were used in the experimentation component of this investigation.
References
220 Triathlon. (n.d.). Swimming with paddles: a training session to develop power. [online] Available at: https://www.220triathlon.com/training/swimtraining/swimming-with-paddles-a-training-session-todevelop-power/ [Accessed 20 Jun. 2022].
Barbosa, A.C., Castro, F.D.S., Dopsaj, M., Cunha, S.A. and Júnior, O.A. (2013). Acute responses of biomechanical parameters to different sizes of hand paddles in front-crawl stroke. Journal of Sports Sciences, 31(9), pp.1015–1023. doi:10.1080/02640414.2012.762597.
Crocker, G.H., Moon, J.F., Nessler, J.A. and Newcomer, S.C. (2018). Energetics of Swimming With Hand Paddles of Different Surface Areas. Journal of Strength and Conditioning Research, p.1. doi:10.1519/jsc.0000000000002637.
D Shaw, T Gorely and R Corban (2005). Instant notes in sport and exercise psychology. New York, Ny: Garland Science/Bios Scientific Publishers.
Gourgoulis, V., Aggeloussis, N., Vezos, N., Antoniou, P. and Mavromatis, G. (2008a). Hand Orientation in Hand Paddle Swimming. International Journal of Sports Medicine, 29(5), pp.429–434. doi:10.1055/s-2007-965570.
Gourgoulis, V., Aggeloussis, N., Vezos, N., Kasimatis, P., Antoniou, P. and Mavromatis, G. (2008b). Estimation of hand forces and propelling efficiency during front crawl swimming with hand paddles. Journal of Biomechanics, 41(1), pp.208–215. doi:10.1016/j.jbiomech.2007.06.032.
Hildebrandt, C., Gopireddy, S.R., Scherließ, R. and Urbanetz, N.A. (2020). A DEM approach to assess the influence of the paddle wheel shape on force feeding during pharmaceutical tableting. Advanced Powder Technology, [online] 31(2), pp.755–769. doi:10.1016/j.apt.2019.11.030.
Hollander, A.P., de Groot, G., van Ingen Schenau, G.J., Toussaint, H.M., de Best, H., Peeters, W., Meulemans, A. and Schreurs, A.W. (1986). Measurement of active drag during crawl arm stroke swimming. Journal of Sports Sciences, 4(1), pp.21–30. doi:10.1080/02640418608732094.
Jean-Claude Chatard (2003). Biomechanics and medicine in swimming IX : proceedings of the IXth World Symposium on Biomechanics and Medicine in Swimming, University of Saint-Etienne, France. Saint-Etienne, France: University Of Saint-Etienne.
Kudo, S., Sakurai, Y., Miwa, T. and Matsuda, Y. (2016). Relationship between shoulder roll and hand propulsion in the front crawl stroke. Journal of Sports Sciences, 35(10), pp.945–952. doi:10.1080/02640414.2016.1206208.
Lanotte, N., Annino, G., Bifaretti, S., Gatta, G., Romagnoli, C., Salvucci, A. and Bonaiuto, V. (2018). A New Device for Propulsion Analysis in Swimming. Proceedings, [online] 2(6), p.285. doi:10.3390/proceedings2060285.
Marinho, D.A., Barbosa, T.M., Reis, V.M., Kjendlie, P.L., Alves, F.B., Vilas-Boas, J.P., Machado, L., Silva, A.J. and Rouboa, A.I. (2010). Swimming Propulsion Forces Are Enhanced by a Small Finger Spread. Journal of Applied Biomechanics, [online] 26(1), pp.87–92. doi:10.1123/jab.26.1.87.
Messinis, S., Beidaris, N., Messinis, S., Soultanakis, H., Botonis, P. and Platanou, T. (2014). Swimming Stroke Mechanical Efficiency and Physiological Responses of 100-m Backstroke with and without the use of paddles. Journal of Human Kinetics, 40(1), pp.171–180. doi:10.2478/hukin-2014-0019.
Minetti, A. (2009). The optimum finger spacing in human swimming. Journal of Biomechanics, [online] 42(13), pp.2188–2190. doi:10.1016/j.jbiomech.2009.06.012.
OGITA, F., ONODERA, T. and TABATA, I. (1999). Effect of hand paddles on anaerobic energy release during supramaximal swimming. Medicine & Science in Sports & Exercise, 31(5), pp.729–735. doi:10.1097/00005768199905000-00017.
Peterson, E.L., Wadhwa, L.C. and Harris, J.A. (2001). Arrangement of aerators in an intensive shrimp growout pond having a rectangular shape. Aquacultural
Engineering, [online] 25(1), pp.51–65. doi:10.1016/S01448609(01)00072-3.
Seifert, L., Toussaint, H.M., Alberty, M., Schnitzler, C. and Chollet, D. (2010). Arm coordination, power, and swim efficiency in national and regional front crawl swimmers. Human Movement Science, [online] 29(3), pp.426–439. doi:10.1016/j.humov.2009.11.003.
Sidelnik, N.O. and Young, B.W. (2006). Optimising the freestyle swimming stroke: the effect of finger spread. Sports Engineering, 9(3), pp.129–135. doi:10.1007/bf02844114.
Tsunokawa, T., Mankyu, H., Takagi, H. and Ogita, F. (2019). The effect of using paddles on hand propulsive forces and Froude efficiency in arm-stroke-only frontcrawl swimming at various velocities. Human Movement Science, 64, pp.378–388. doi:10.1016/j.humov.2019.03.007.
van Houwelingen, J., Willemsen, D.H.J., Kunnen, R.P.J., van Heijst, G.F., Grift, E.J., Breugem, W.P., Delfos, R., Westerweel, J., Clercx, H.J.H. and van de Water, W. (2017). The effect of finger spreading on drag of the hand in human swimming. Journal of Biomechanics, [online] 63, pp.67–73. doi:10.1016/j.jbiomech.2017.08.002
Scientific Research in School Volume 4 Issue 1 2022 Pharmacalogical, Microbial & Applied Science
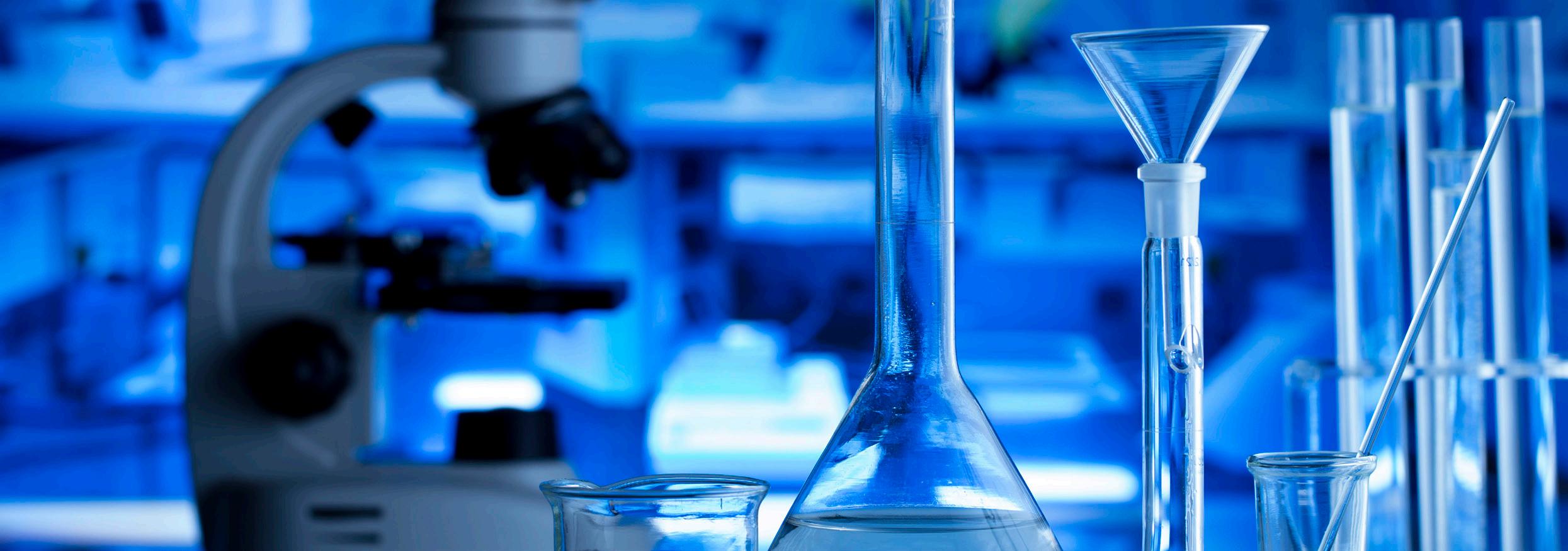
Biology is the study of living things, astounding in the breadth of opportunities it offers for student investigation. We had a large range of biologically-oriented projects this year that spanned the areas of pharmacology, microbiology and applied science.
We are delighted with the work from our students who pursued research projects in the area of Pharmacological, Microbial and Applied Science. Students’ research varied vastly in scale and topic: from the molecular to entire organisms; from pharmaceutical to aesthetic.
On the molecular scale, Josh collaborated with Breaking Good, an international citizen science project. He scanned a database to find potential compounds that may be useful for the treatment of the fungal infection Eumycetoma, a disease primarily affecting poor people living in remote rural regions of Asia, Latin America and Africa.
On the microscopic scale, we had several projects looking at antimicrobials. Ben explored ultrasound as a method for killing bacteria and rose to the challenge of making zinc-oxide “hedgehogs” as a catalyst to increase its efficiency. He collaborated with the University of Sydney for stunning electron microscope images of his zinc oxide structures. In a well-designed project, Nilan investigated the previously untested anti-microbial properties of an Australian scorpion, Urodacus elongatus. He raised the scorpions himself and perfected an elegant technique for venom-milking in the course of his work. Sass persisted through challenges to focus on determining whether an over-the counter antihistamine could act as an antimicrobial, comparing the effectiveness of racemic and enantiopure formulations of the drug. Luke obtained definitive results as he explored the relationship between the concentration of E. coli and the concentration of the well-known antibiotic, ampicillin, required to inhibit microbial growth. He raised the possibility that the cost of antibiotic treatment could be reduced if less antibiotic could be used with the same effectiveness.
On the macro scale, in a fascinating study, Cassie analysed the images of many hundreds of faces to scientifically determine whether symmetry is the basis of beauty. Her analysis yielded surprising results.
We hope you are inspired by the beauty in each of these reports.